Frontiers | Science News
- Science News

Research Topics
Reach for the stars: research topics on space exploration.
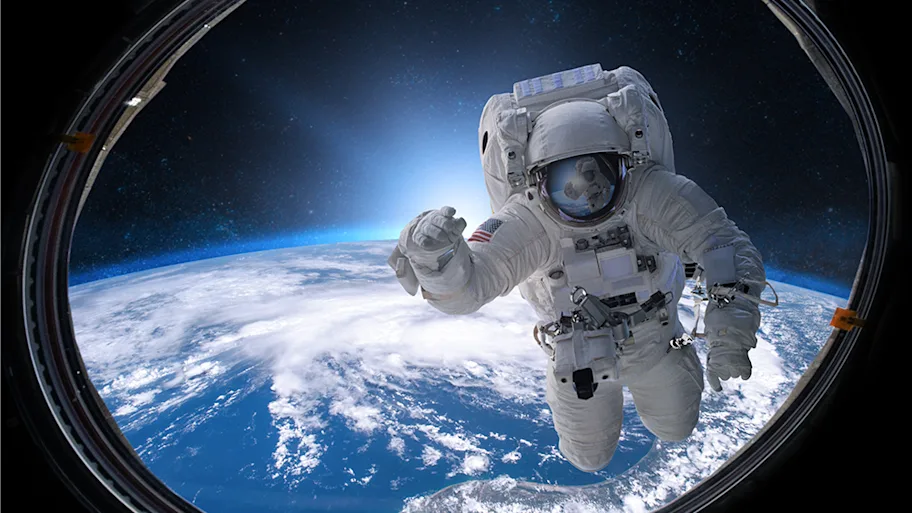
With recent advances in commercial space exploration, we have curated a list of our best Research Topics on outer space. Explore collections edited by experts from NASA, The Goddard Space Flight Center, Space Science Institute, German Aerospace Center, Canadian Space Agency, National Space Science Center, European Space Agency, International Space University, and many more.
Research Topics:
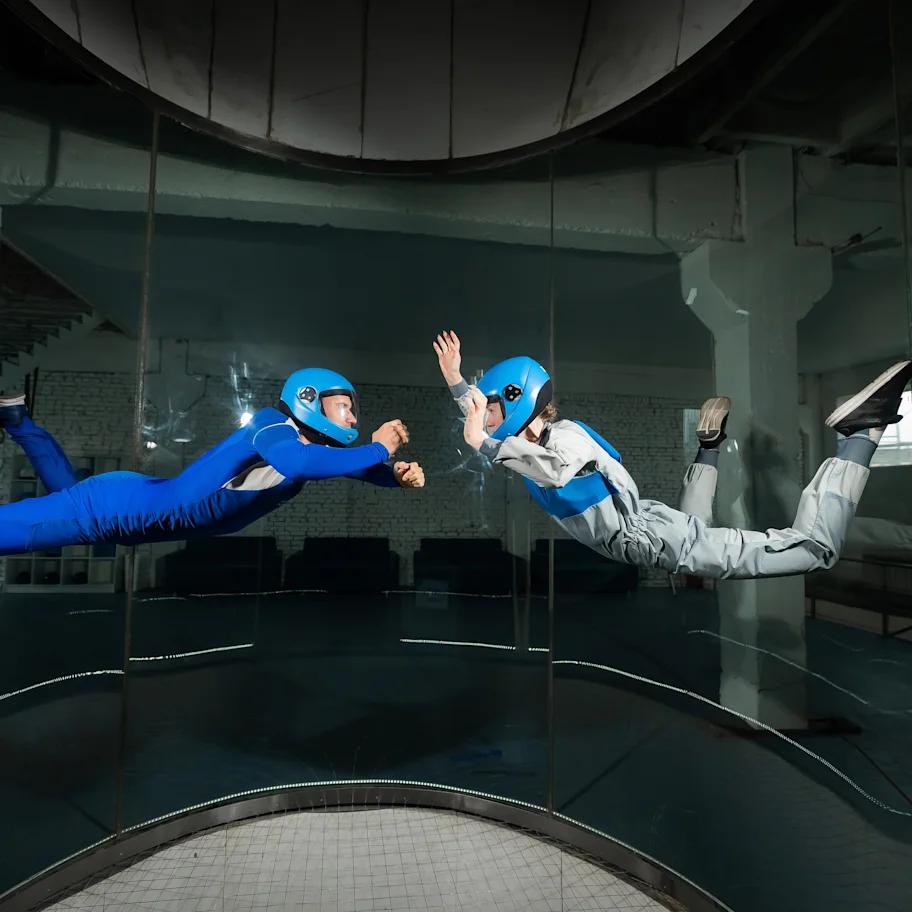
Optimization of Exercise Countermeasures for Human Space Flight – Lessons from Terrestrial Physiology and Operational Implementation
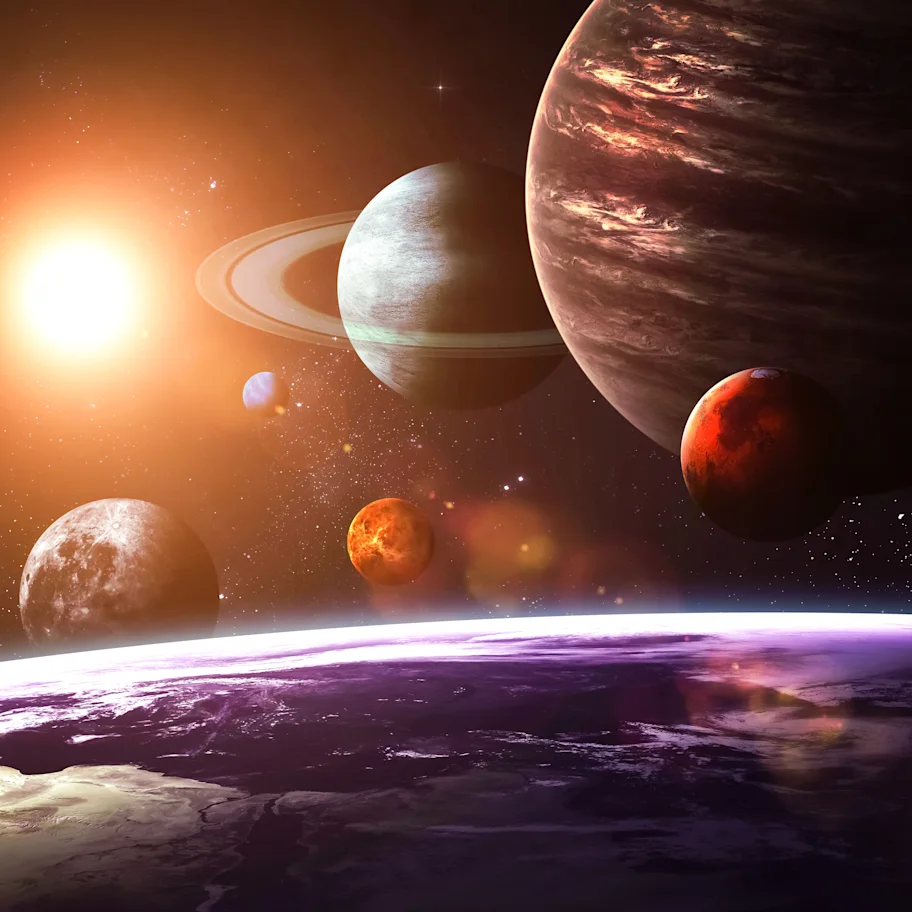
Biology in Space: Challenges and Opportunities
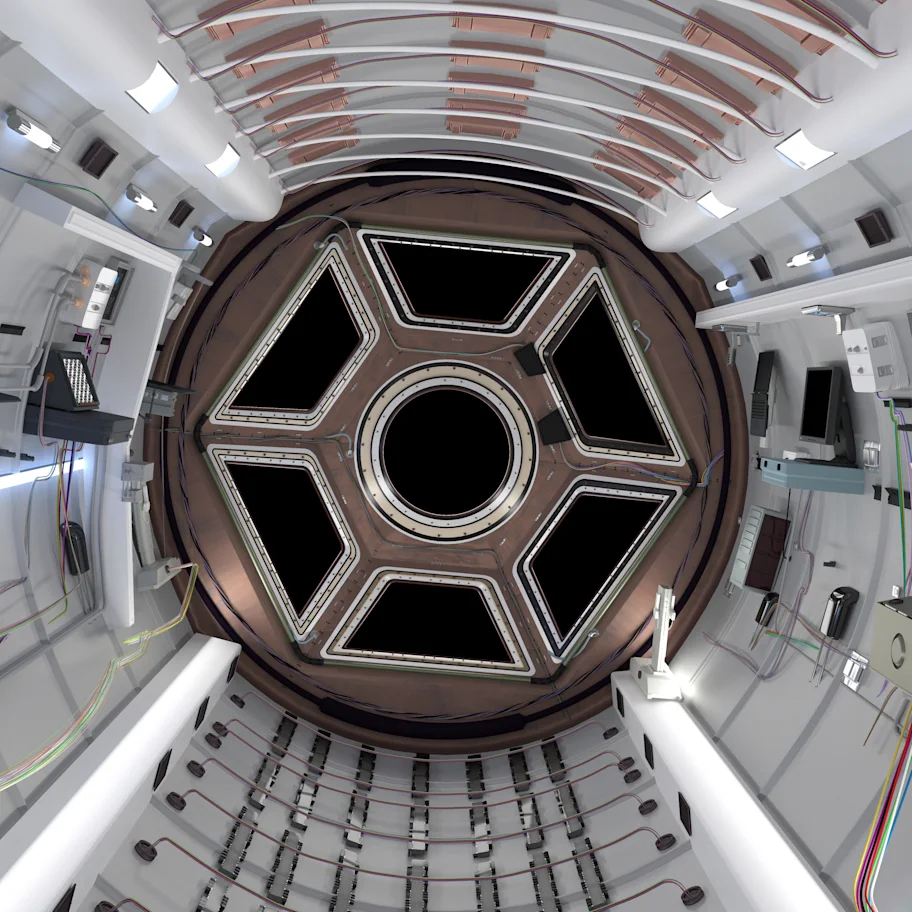
Microbiology of Extreme and Human-Made Confined Environments (Spacecraft, Space Stations, Cleanrooms, and Analogous Sites)
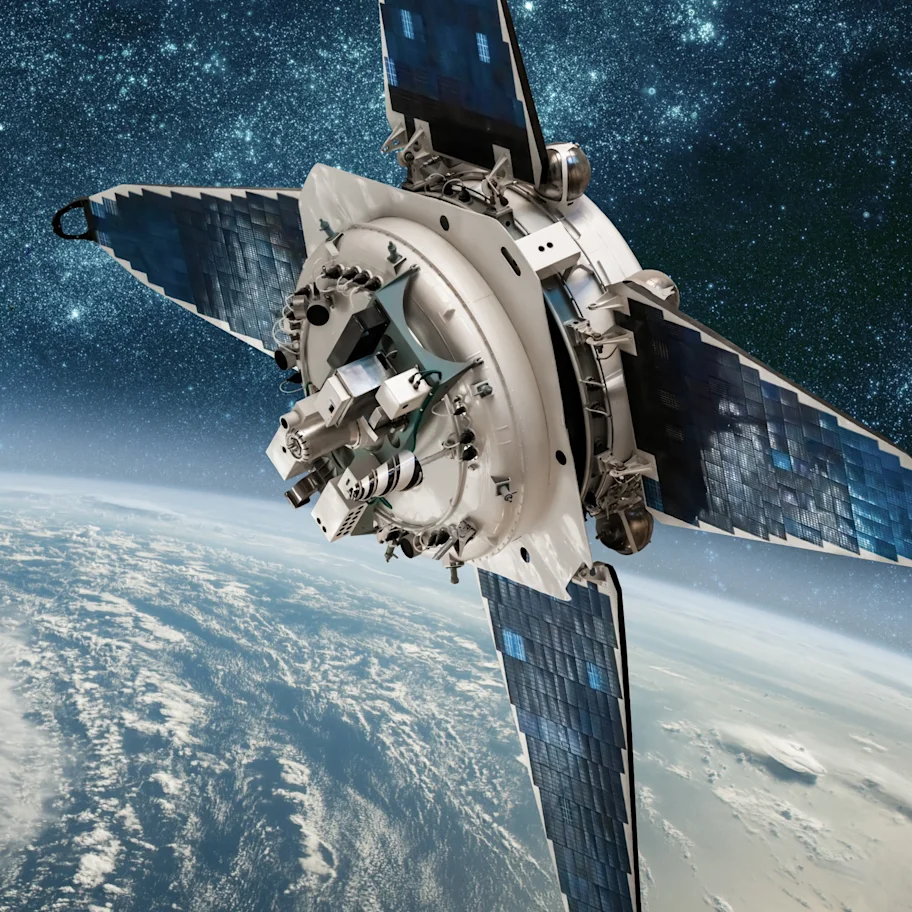
Geospace Observation of Natural Hazards
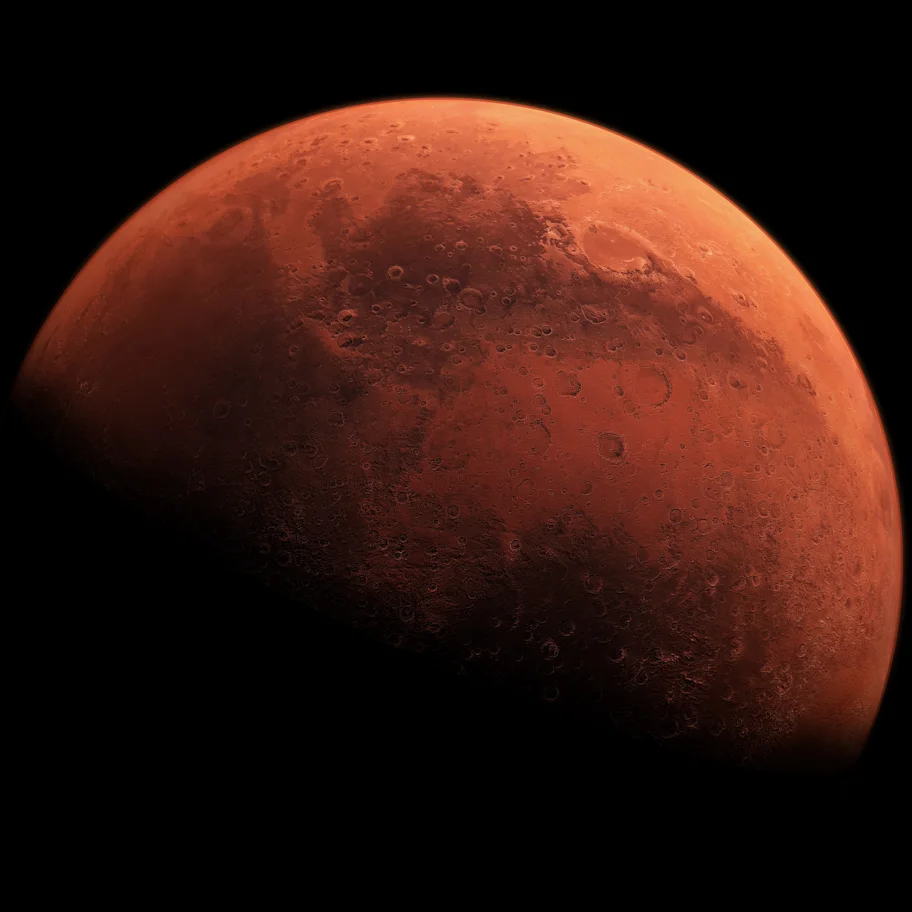
Astrobiology of Mars, Europa, Titan and Enceladus - Most Likely Places for Alien Life
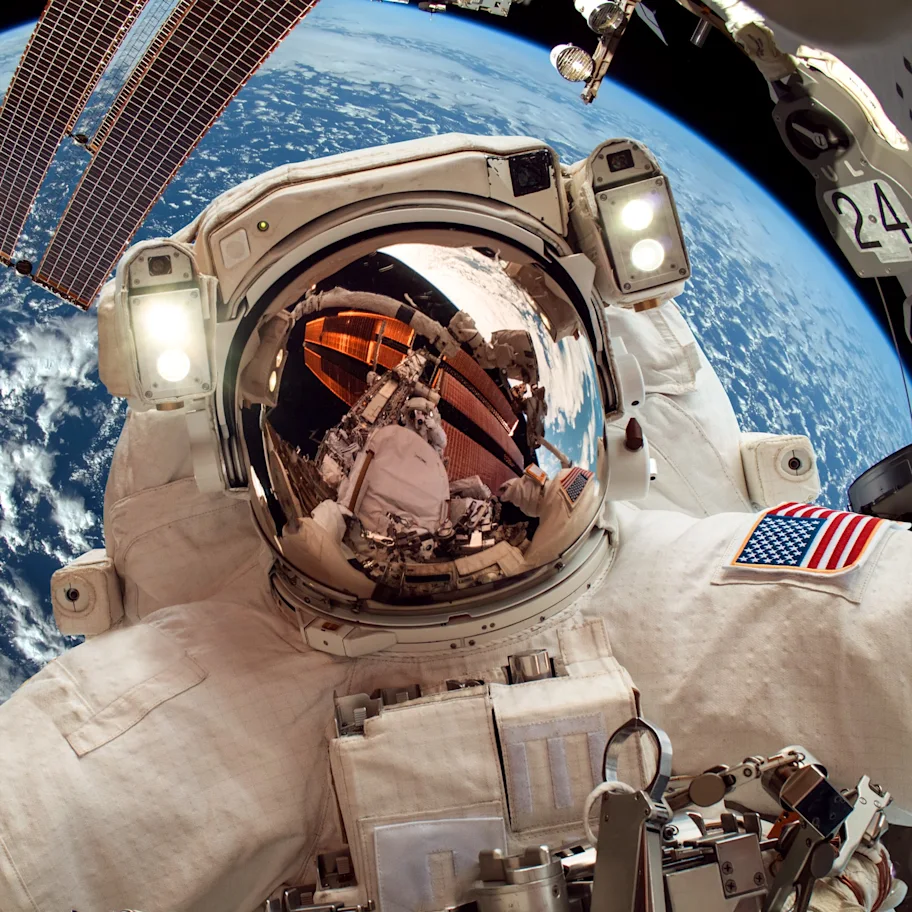
Imagining the Future of Astronomy and Space Science
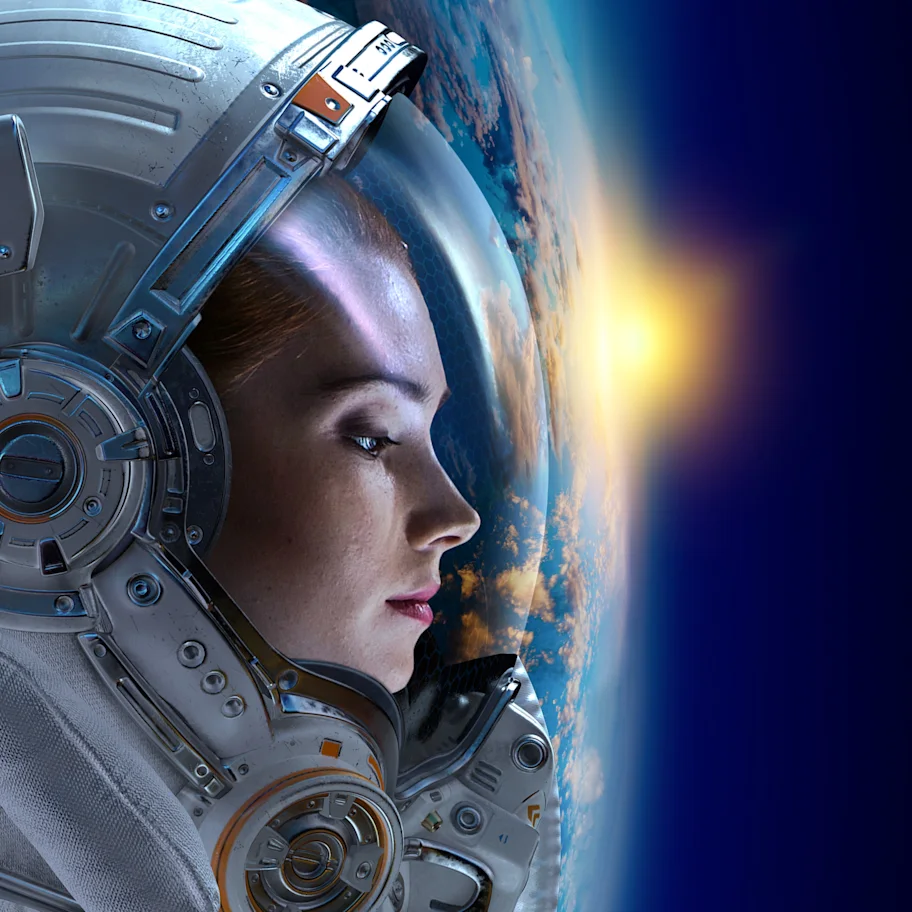
Brains in Space: Effects of Spaceflight on the Human Brain and Behavior
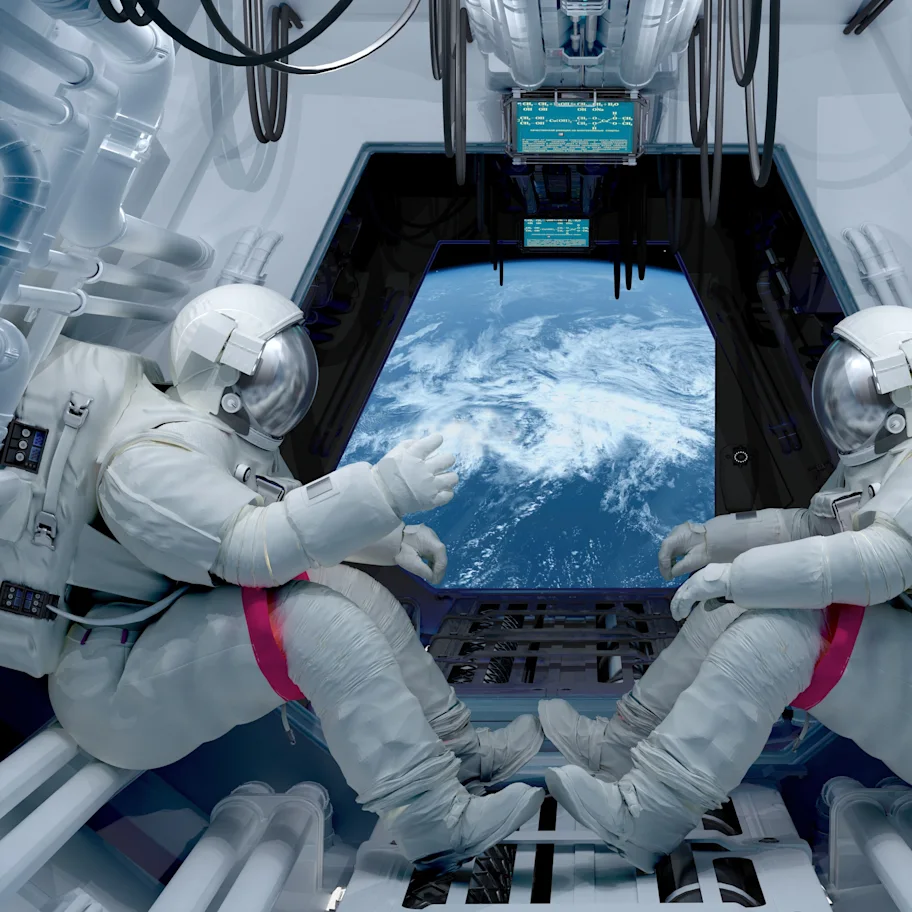
Creative Performance in Extreme Human Environments: Astronauts and Space
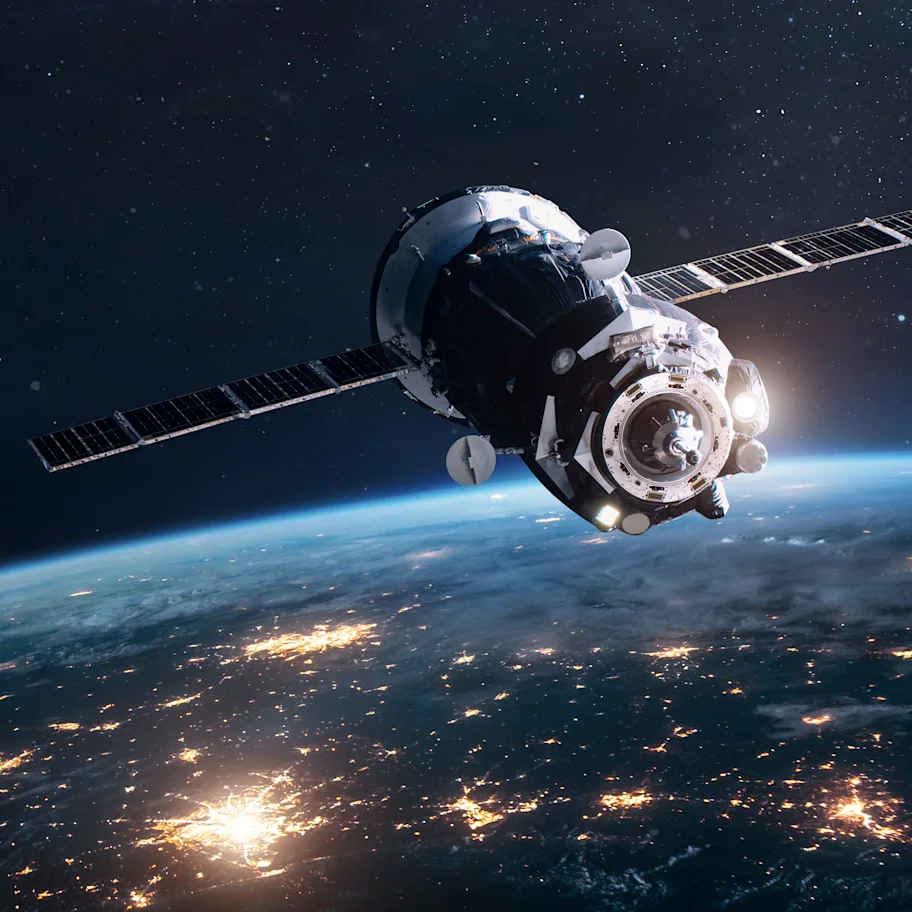
Space Traffic Management: a new era in Earth orbit
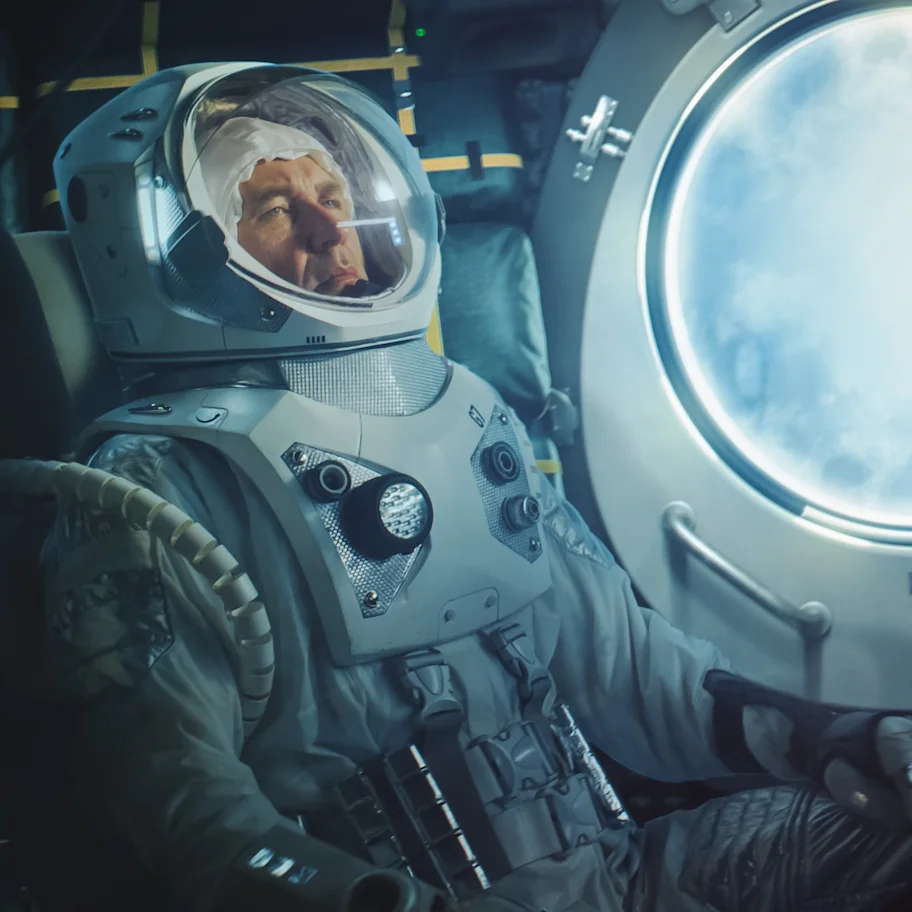
Wound Management and Healing in Space
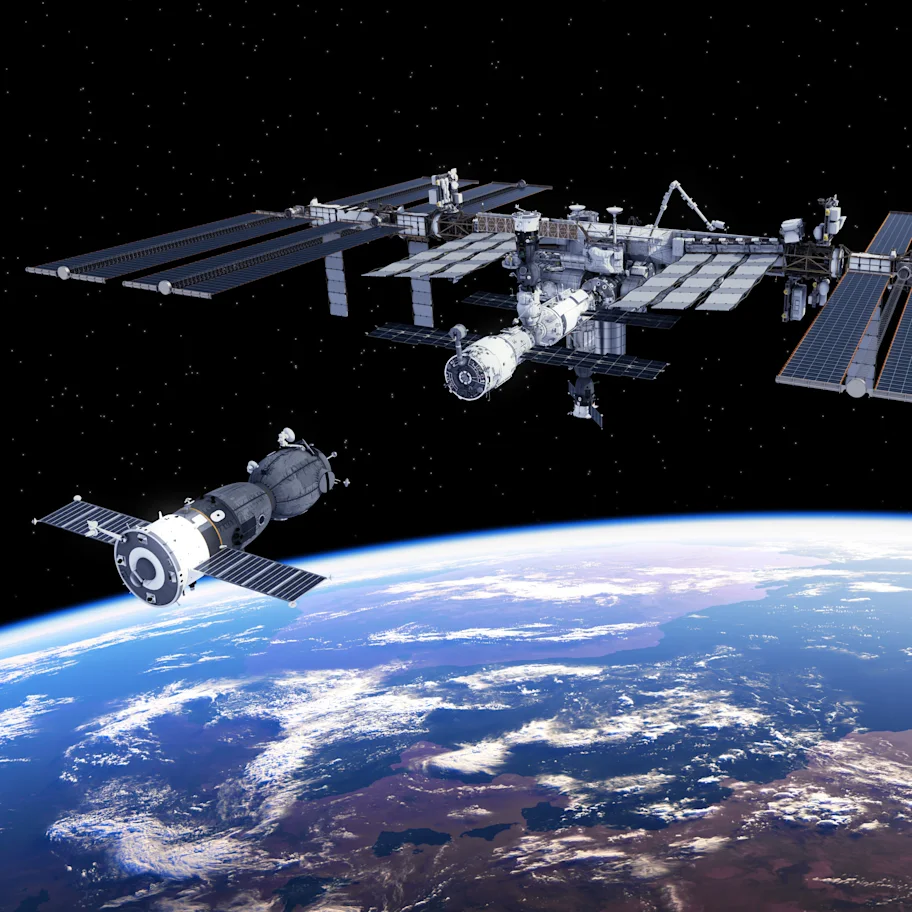
Robotic Manipulation and Capture in Space
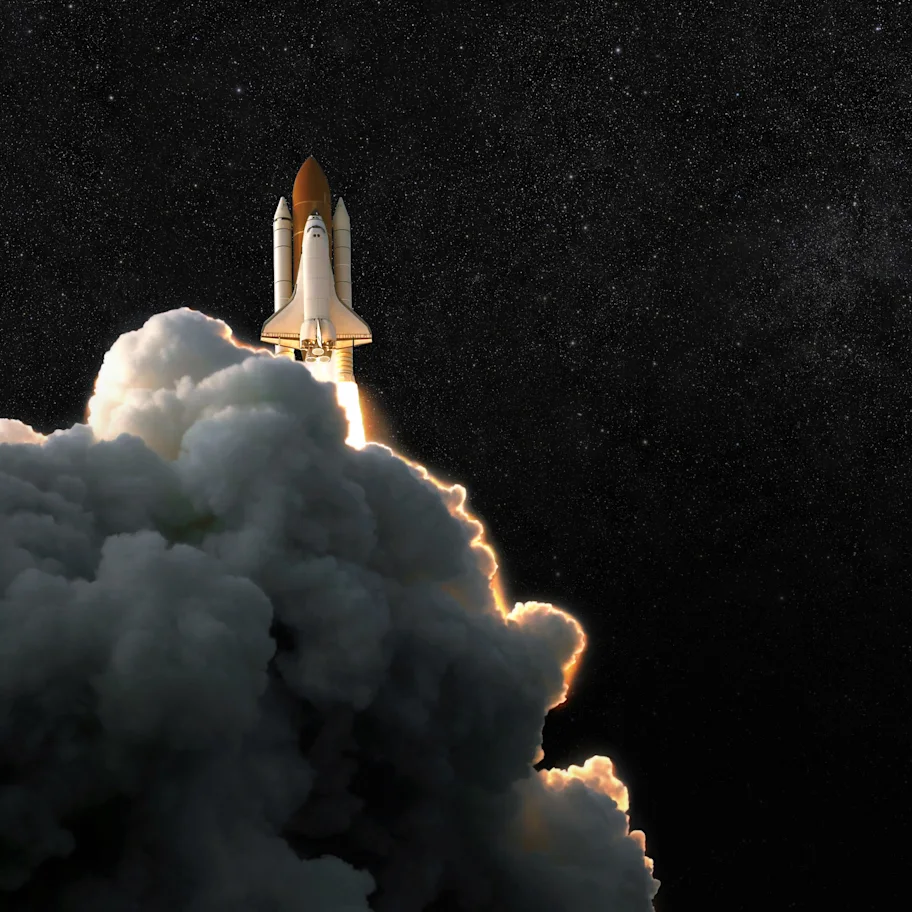
A Multidisciplinary Approach to designing Sensorimotor Adaptation countermeasures for space exploration missions
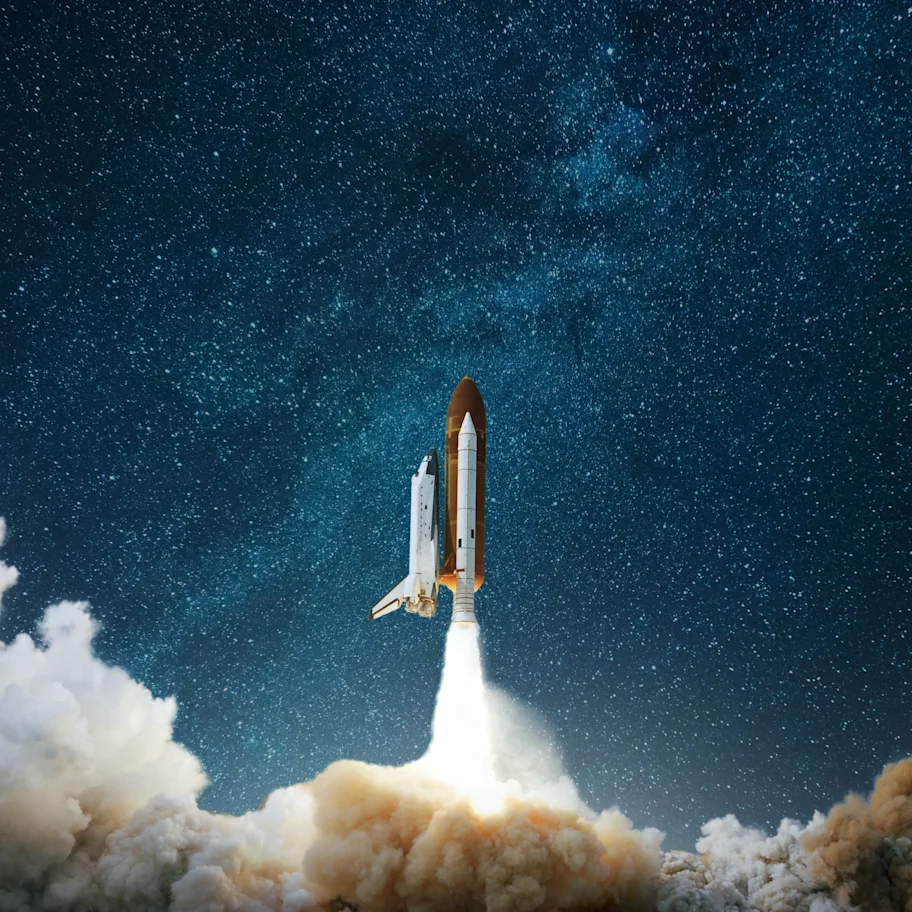
Active Experiments in Space: Past, Present, and Future
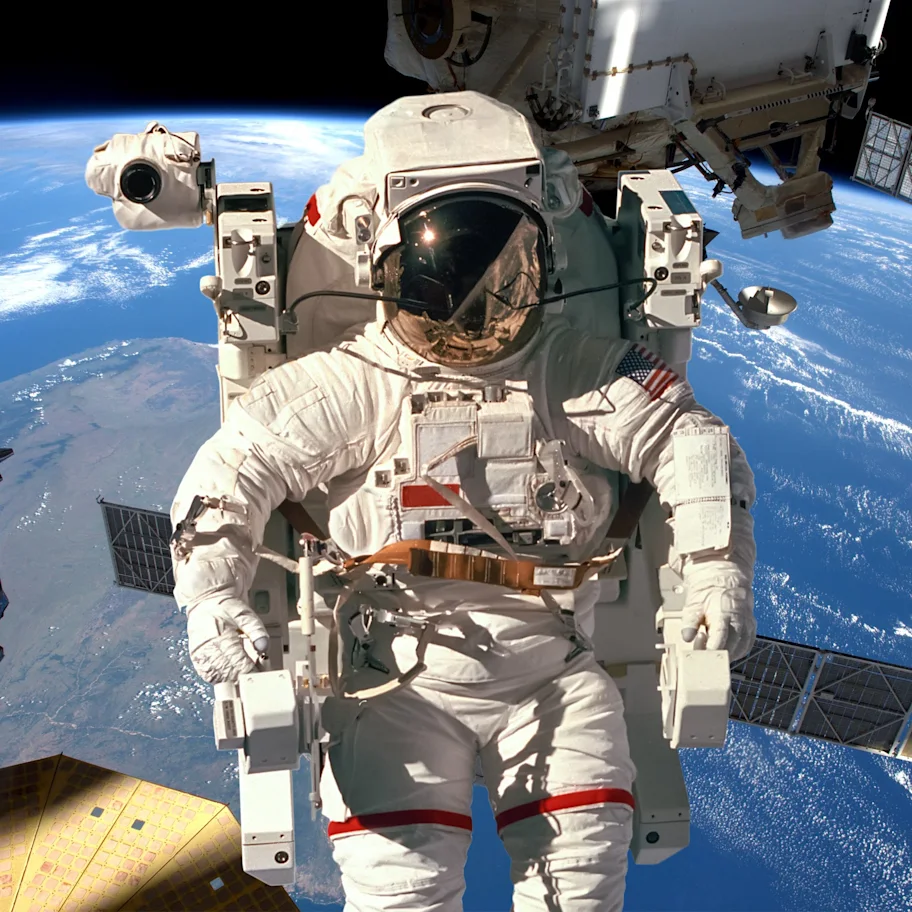
On-orbit Manufacturing and Assembly Technologies for Future Space Activities
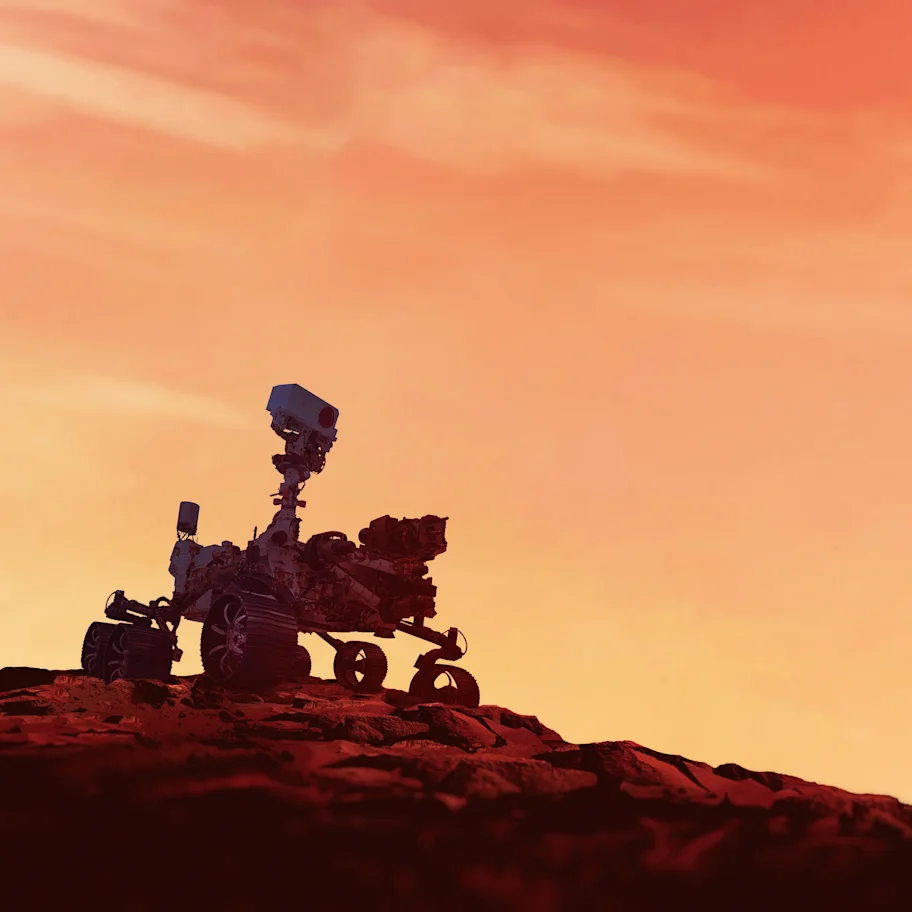
Current and Future Instrumentation for the Detection and Identification of Signatures of Life on Mars and Beyond
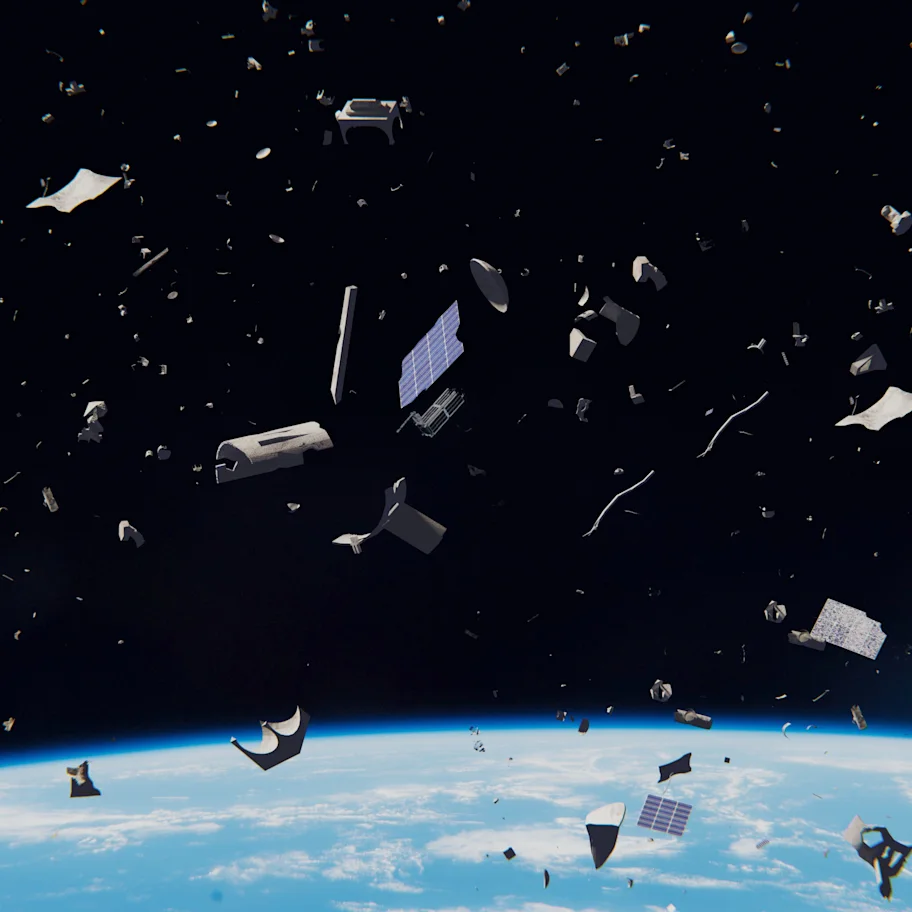
On-Orbit Servicing and Active Debris Removal: Enabling a Paradigm Shift in Spaceflight
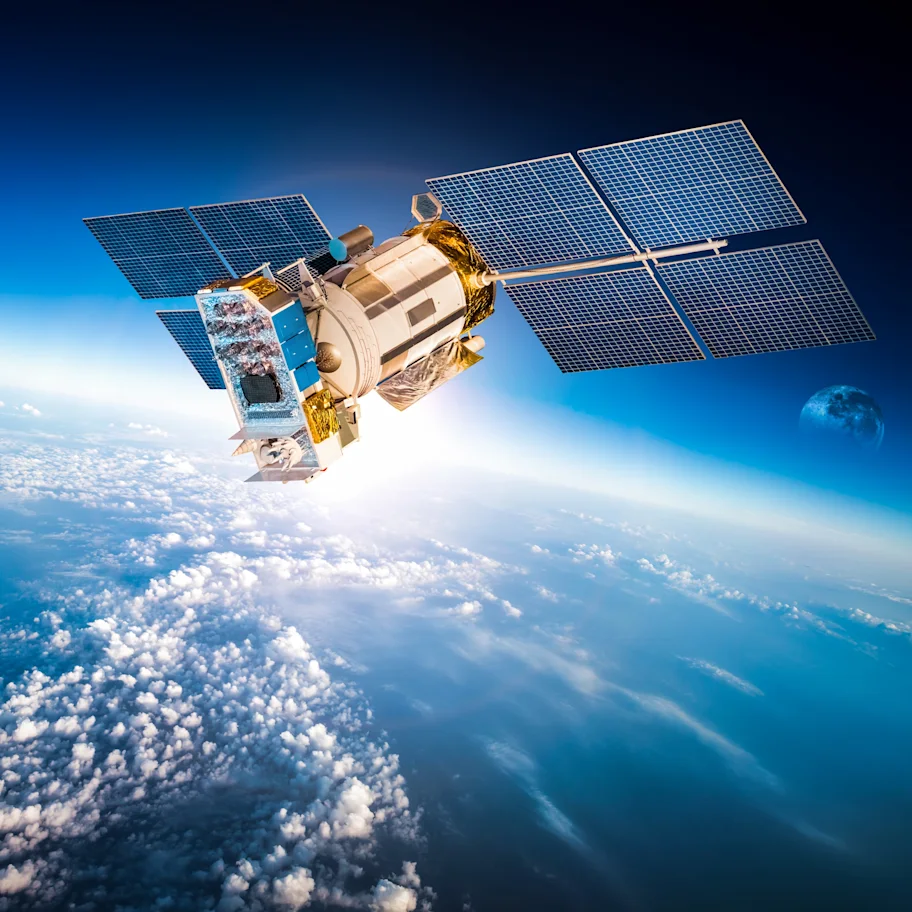
Space Weather with Small Satellites
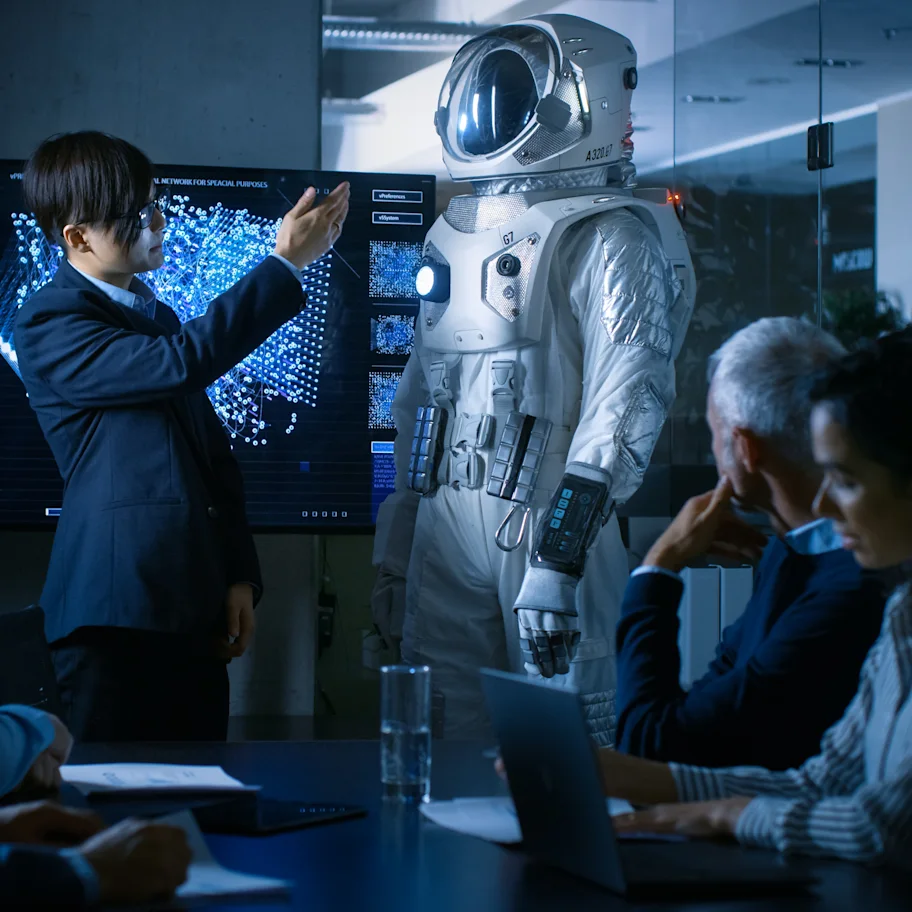
AI in the Space Sciences
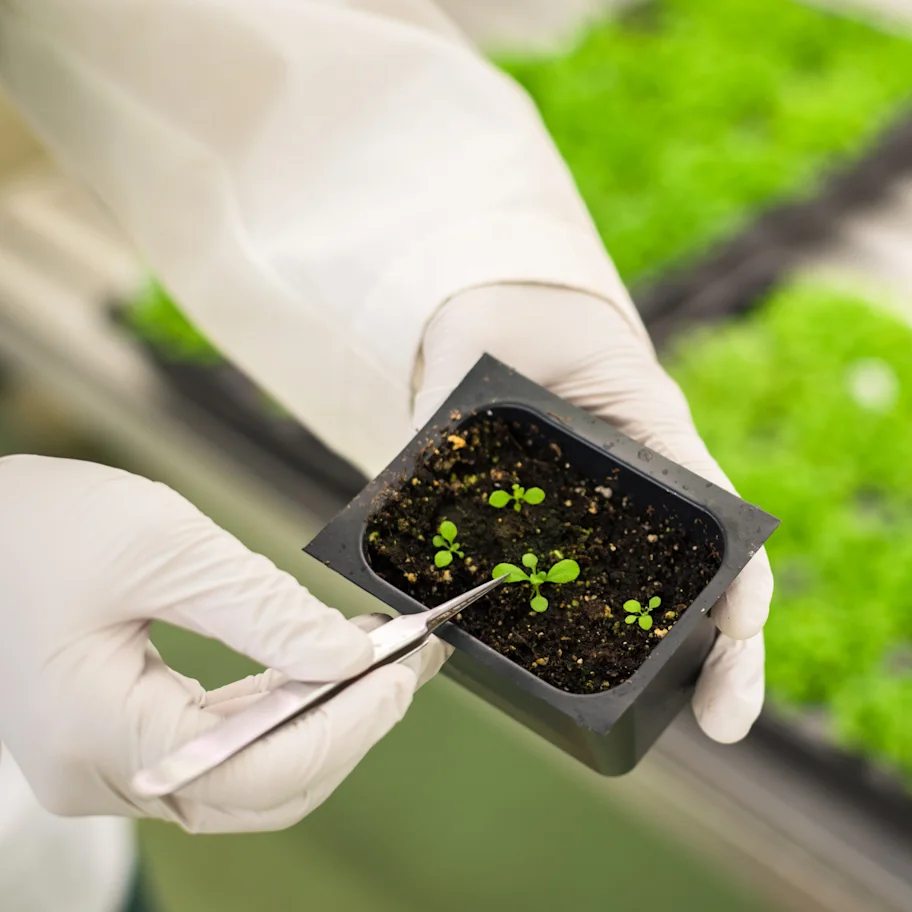
Higher Plants, Algae and Cyanobacteria in Space Environments
Post related info
October 11, 2021

Frontiers Communications
Post categories, space sciences and astronomy, related subjects, research topics, related content.

Life from Earth could temporarily survive on Mars

Meet a team of scientists working to prevent interplanetary pollution that could pose a threat to life on Earth and other planets

NASA space data can cut disaster response times, costs
Latest posts.
Ioly Kotta-Loizou – The role of mycoviruses for the future of disease in medicine and agriculture

Migrating birds have stowaway passengers: invasive ticks could spread novel diseases around the world

Students who use dating apps take more risks with their sexual health

Chlorine and cocoa butter could protect corals from disease and decrease antibiotic pollution of the oceans

Consistent climate action can change the air we breathe: Here are five Frontiers articles you won’t want to miss
Get the latest research updates, subscribe to our newsletter
Research Topics List
JPL's charter is to conduct robotic space missions for NASA, to explore our own and neighboring planetary systems, understand the origin and evolution of the universe and make critical measurements to understand our home planet and help protect it. We do this by developing integrated capabilities in engineering, science and technology, in a unique environment that strives for excellence in any of the three areas. To be successful in our ability to implement missions for NASA, we foster research in those areas of space-based science that establish our leadership in the science community and those technologies that allow the innovations that are crucial to maintaining our competitive edge. Our science, technology and engineering research covers many areas of planetary, astrophysics and Earth science, both as basic research leading to new observations and mission concepts, as well as research based on the data acquired by JPL flight projects. Our technology research covers areas ranging from robotic systems, a range of in-situ and remote sensing instruments, deep space communications and navigation, information systems, precision flying and planetary protection and survivability.
Big Questions
The research at the Center for Astrophysics | Harvard & Smithsonian centers on humanity’s greatest unresolved questions about the nature of the universe. These questions have been pondered for generations, and our research is bringing us closer to answers than ever before.
- What conditions are necessary for life?
- Does life exist outside of the solar system?
- Why do we need an extremely large telescope like the Giant Magellan Telescope?
- How do stars and planets form and evolve?
- What happened in the early universe?
- What do black holes look like?
- What happens to space time when cosmic objects collide?
- Why do galaxies differ so much in size, shape, composition and activity?
- What is the universe made of?
- How can astronomy improve life on earth?
Thank you for visiting nature.com. You are using a browser version with limited support for CSS. To obtain the best experience, we recommend you use a more up to date browser (or turn off compatibility mode in Internet Explorer). In the meantime, to ensure continued support, we are displaying the site without styles and JavaScript.
- View all journals
- My Account Login
- Explore content
- About the journal
- Publish with us
- Sign up for alerts
- Review Article
- Open access
- Published: 25 March 2023
Toward the utilisation of resources in space: knowledge gaps, open questions, and priorities
- Jan Cilliers 1 na1 ,
- Kathryn Hadler 1 , 2 na1 &
- Joshua Rasera ORCID: orcid.org/0000-0003-0136-3308 1 na1
npj Microgravity volume 9 , Article number: 22 ( 2023 ) Cite this article
3834 Accesses
7 Citations
3 Altmetric
Metrics details
- Engineering
- Scientific community
There are many open science questions in space resource utilisation due to the novelty and relative immaturity of the field. While many potential technologies have been proposed to produce usable resources in space, high confidence, large-scale design is limited by gaps in the knowledge of the local environmental conditions, geology, mineralogy, and regolith characteristics, as well as specific science questions intrinsic to each process. Further, the engineering constraints (e.g. energy, throughput, efficiency etc.) must be incorporated into the design. This work aims to summarise briefly recent activities in the field of space resource utilisation, as well as to identify key knowledge gaps, and to present open science questions. Finally, future exploration priorities to enable the use of space resources are highlighted.
Similar content being viewed by others
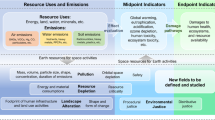
Conceptualizing space environmental sustainability
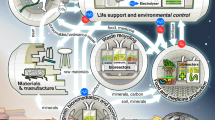
Toward sustainable space exploration: a roadmap for harnessing the power of microorganisms
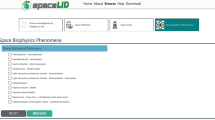
Exploring outer space biophysical phenomena via SpaceLID
Introduction.
The use of space resources is critical for the future of long-term and deep-space exploration. Space exploration presents challenges for sustainability; single-use launchers, non-refuelable satellites, and a need for all hardware and consumables to be supplied from Earth, all add appreciable resource use and cost to space programmes. Fortunately, significant progress is being made: SpaceX are Blue Origin are demonstrating the value of re-usable launch systems 1 ; on-orbit refuelling is being developed by start-ups such as Orbit Fab and Orbital Express, as well as established actors, such as Airbus and Busek 2 .
The use of space resources to provide propellant, habitation and materials critical to support human life (e.g. water, oxygen) will unlock the full potential of space exploration, enabling humans to travel further and spend longer in space 3 , 4 , 5 . This will transform the economics of space exploration.
The use of space resources, known as in situ resource utilisation (ISRU), or more generally as space resource utilisation (SRU), is not a new concept. A detailed history of SRU is provided by Meurisse and Carpenter 6 . In brief, the utilisation of space resources was first suggested by Konstantin Tsiolkovsky, widely considered the originator of modern approaches to rocketry, in 1903 7 , 8 . Lunar SRU was proposed by Clarke 9 in the 1950s. During the Apollo Era in the 1960s, SRU was suggested by Carr 10 as a practical means to reduce launch mass and terrestrial dependency. In the subsequent 50 years, the concept has grown in maturity. Many terrestrial studies have been undertaken to design and test candidate technologies (e.g., refs. 11 , 12 , 13 , 14 , 15 , 16 , 17 ).
As of 2022, SRU has been demonstrated only once in space, despite these technologies playing an key role in ESA’s and NASA’s space exploration road maps 12 , 18 . The MOXIE ( M ars OX ygen I SRU E xperiment) payload on board NASA’s Perseverance Rover produced oxygen from Mars’ CO 2 -rich atmosphere in 2021 by solid oxide electrolysis 19 . Lunar SRU demonstration missions are under development (e.g., refs. 20 , 21 ), and preliminary missions to test new SRU legal and economic frameworks are scheduled throughout 2023, for example ispace inc.’s HAKUTO-R Mission 1, currently en route to the Moon 22 , 23 .
Today, accessing and using space resources is a focus of many space agencies 18 , 24 , 25 , 26 , 27 , governments 28 , 29 , 30 , 31 , intergovernmental organisations 32 , 33 , and private industry 34 , 35 , 36 . More recently, there has been renewed interest in SRU for a number of applications, such as:
Producing oxygen and metals on the Moon and Mars (e.g. refs. 19 , 37 , 38 , 39 , 40 , 41 , 42 , 43 , 44 , 45 , 46 );
Extracting water from the lunar poles (e.g. refs. 47 , 48 , 49 , 50 , 51 );
Extracting water, volatiles and metals from near-Earth objects (e.g. refs. 52 , 53 , 54 , 55 , 56 , 57 , 58 );
Construction of habitats and thermal shelters, including by additive manufacturing (e.g. refs. 59 , 60 , 61 , 62 , 63 , 64 , 65 , 66 , 67 , 68 , 69 , 70 ); and,
The manufacture of equipment and technology from local resources (e.g. refs. 71 , 72 , 73 , 74 , 75 , 76 ).
Demonstration-scale SRU projects are a viable, necessary first step for the industry. Their success will broaden appreciably the knowledge base of the SRU and lunar science communities. Detailed knowledge of the local geology, mineralogy and regolith characteristics will enhance greatly confidence in the designs of mining, extraction and production systems at an industrial scale. Other science questions, intrinsic to each specific process, should be addressed to optimise the design of industrial-scale systems. Both the environmental operating conditions (e.g., local electrostatic and radiation environments) and engineering constraints (e.g. energy use, required throughput, expected efficiency, etc.) will affect equipment designs significantly 77 ). The success of large-scale resource utilisation processes is dependent therefore on a sufficient knowledge of the specific resource and region of interest, as well as the technology capability required to extract useful products.
This work was developed following the European Space Agency’s SciSpacE Space Resources White Paper exercise. Here, knowledge gaps, open science questions, and research priorities for the lunar science and SRU communities are identified. As the capabilities and limitations of SRU are clarified through in situ demonstrations, it will be possible to address many of these gaps and questions, and in doing so, will improve greatly the development of large-scale SRU technologies. Furthermore, answering these questions will provide tremendous value to the scientific community.
The SRU process
The extraction and use of space resources is analogous to the extraction and use of terrestrial resources 78 , 79 . First, the given resource (e.g. oxygen, water ice) must be identified through prospecting and ground truth exploration to increase certainty 80 , 81 . The composition of the surrounding material and the characteristics of the specific resource within that host material must be understood. The variability in the distribution of the resource in a given region is also required. For example, water ice present within regolith or buried under regolith at the lunar poles varies both spatially and by depth 50 , 82 . Adopting suitably modified terrestrial industry standards and best practices for exploration and reporting (e.g., JORC and LORS 81 ), as well as common terminology 78 will encourage participation of, and attract investment from non-space actors in SRU.
The chain of technologies linked together to process a particular ore body on Earth is described by a flowsheet 78 . The flowsheet can be subdivided broadly into three key stages: excavation, beneficiation, and extraction of the final product 78 . Excavation has been explored thoroughly in the literature 83 , as have extraction methods 84 . Beneficiation is the process in which mined material is broken or agglomerated and classified by size into a range suitable for further processing, and also to concentrate one component of interest (e.g. water or ilmenite) by physical removal of undesired components. The beneficiation of mined space material into a form suitable for extraction of the require final product has been studied far less in comparison 85 .
In terrestrial mining, the resource, the surrounding material, the location, and the technology used to extract the resource are matched in the process flowsheet such that either:
The specific resource and its location are targeted depending on available technology; or,
The technology is designed to meet the extraction requirements of a specific target resource.
Demonstration missions to prove SRU technologies and to raise TRLs have immense value for characterising the potential inputs to the flowsheet. However, the characteristics of resource host material on the Moon, Mars or elsewhere in space are also key inputs to flowsheet design. The processing technologies required must be chosen to maximise confidence in the production levels of the resource as well as the overall operational efficiency. It is inappropriate to assume that a ‘one size fits all’ approach to excavation, beneficiation and extraction would be suitable for SRU. Terrestrial mining operations select carefully the mining equipment used based on the characteristics of the target resource; a SRU will benefit undoubtedly from adopting a similar approach.
Space resource utilisation requires engineering solutions to produce a reliable supply of usable products from a naturally variable feedstock 77 . The use of mineral resources for SRU remains untested anywhere in space, however this will change in the coming years with demonstration missions (e.g. PROSPECT), the exploration of the lunar poles, and NASA’s upcoming regolith collection missions 20 , 22 . For SRU to become a realisable option for future space travel, it will be important for early demonstration missions to address as many open science questions as possible, as this will enable ultimately the implementation of SRU at an industrial scale.
Data: the key knowledge gap
There remain many aspects of SRU that are poorly quantified, through lack of available data and samples, and limitations with demonstrating space technologies on the surface of the Earth. The data required to enable SRU in the future can be categorised into two groups: environmental data and resource data. Such data will further have intrinsic scientific value.
Environmental data are critical for the development of robust equipment with high operational availability and long-term usage in mind. Deep knowledge of the local environmental conditions will impact directly the design choices made to ensure that only the most robust and reliable technologies are deployed. The operating environment will affect significantly the design and operation of any process, for example:
Variation in the electrostatic properties of regolith under different conditions (e.g. day and night);
Designing operations for lower gravity, different atmospheric characteristics, or no atmosphere at all;
Designing to withstand extremely high and low temperatures, and the process of cycling through them;
Material handling in dusty environments;
Local radiation environment; and,
Designing for reliability and durability.
Resource data are imperative for selecting appropriate technologies for SRU operations. These data must specify:
The location of the resource;
The resource properties (e.g. concentration, phase, associations);
The host material properties (e.g. regolith mineralogy, particle size distribution, particle shape, geotechnical properties);
The variability in the resource and host material properties (by region, by location and by environmental conditions); and,
The effect of the resource properties on utilisation (e.g. reactor efficiency, construction strength).
To bridge these gaps, high resolution orbital data sets must be captured and correlated to ground-truth exploration activities at select targets. As an illustration, of the proposals that have been developed previously for large-scale exploitation of resources, several have focused on the extraction of water ice at the lunar poles for propellant production (e.g., refs. 17 , 47 , 48 ). These detailed elaborations of production facilities on the Moon are based on assumptions about the form, quantity, variability, and behaviour of icy regolith. At present, there is no ground truth data to verify any of these assumptions, and there are major uncertainties associated with them 86 . Rigorous prospecting and ground truth exploration must be performed in order to raise the level of geological certainty 80 , 81 . This is standard practice on Earth for the economic development of mines, and will be equally relevant for SRU 80 , 81 .
The regolith samples returned by the Apollo and Luna missions of the 1960s and 1970s have incredible value for testing bench scale apparatuses, however the amount of lunar material made available for testing is insufficient to develop industrial-scale equipment. Furthermore, the successful development of terrestrial SRU demonstrators will be dependent on the availability of suitable simulants. However, the scientific community, along with private and public sector actors, must agree on a standardised approach for the characterisation of lunar regolith and lunar regolith simulants. Such a standard would enable honest, transparent, like-for-like comparisons of feedstocks and equipment performance, as well as provide justification for using certain simulants for any given technology demonstration.
Open science questions
There are many open science questions in space resource utilisation due to the novelty and relative immaturity. The following open questions are focused specifically on the applied science aspects needed upscale SRU to an economically viable, industrial scale. One of the benefits of this field is that, with careful design, data and samples required to design SRU processes can be used also to answer open questions of interest to the lunar science community.
Which resource characteristics are required to establish the viability of a resource? This encompasses characteristics of the specific resource such as concentration and occurrence, in addition to those of the host material. Regolith properties, such as size distribution, texture, cohesiveness, electrostatic charge and mineralogy, will be of interest 85 , 86 . The minimum amount of data to increase the geological certainty of a deposit and how it is collected should also be considered 77 , 78 , 80 , 81 . The use of such datasets in fundamental scientific studies (e.g. geology, planetary evolution) should be a key factor in extra-terrestrial mine planning.
How have geological and environmental processes affected properties of resources and how do these properties affect extraction processes? Environmental factors include geological processes (e.g. volcanism, crustal formation), impacts (delivery of resources versus loss of resources during impact reprocessing), solar wind and cosmic ray exposure, and magnetic anomalies. There are many fundamental science questions that can be addressed by understanding the geological and environmental processes occurring in the region of a given space resource, for example impact rate to create local regolith environment. For space resource applications, however, these processes will affect the composition and characteristics of the resource and the host material (e.g. burial depth, porosity, agglutinate content) 87 , 88 , 89 . Geotechnical properties, for example, are affected by the geological makeup (mineralogy, chemistry), impact and space exposure history of the lunar regolith 90 .
How do the local environmental conditions affect the resource and potential operations? For example, electrostatic charging of regolith, gravity, thermal conditions, atmospheric conditions, and radiation. Electrostatic charging of lunar regolith is known to present operational challenges, particularly with regards to reliability 91 , 92 , 93 , 94 . It is not possible to replicate simultaneously all aspects of the lunar environment on Earth, and while rapid developments are being made in the field of regolith simulants 95 , 96 , 97 , the production of agglutinates remains difficult at any scale 98 . Questions remain on the magnitude and distribution of electrostatic charging of regolith, and on how this can be mitigated. In situ studies are critical to enhance understanding. Another aspect of interest is the rate of change of environmental conditions (e.g. the atmosphere of Mars).
What is the variability of resources in a target region and the effect on processing and extracted product variability? Variability is an aspect of resource use that is critical in the long term. Variability in the resource and the host material affects every step of the process, from excavation through to purification of the final product 77 , 99 . Additionally, an understanding of the geological processes, as highlighted previously, will enable better prediction of the resource variability.
What are the physical and chemical processes that can be applied to extract and process local resources? Many processes have been proposed 83 , 84 , 85 , however not all are appropriate for all locations (e.g., hydrogen reduction in the lunar highlands 100 ). Strategies for establishing either the most suitable location or the most suitable process are required. Consideration also must be given to the effect of local conditions on process efficiency; this includes feedstock characteristics. End-to-end processing of the resource, including waste disposal/re-use and product storage are also required.
Outlook and summary
The confident design and successful operation of large- or industrial-scale SRU process operations requires detailed knowledge of the specific resource of interest and suitable extraction technologies. The priority for near-term demonstration missions and future exploration programmes must be to gather high-resolution, high-fidelity data about the performance characteristics of equipment, the local environmental conditions, and the availability of target resources. The terrestrial mining sector has immense expertise in resource exploration; combining this knowledge base with that of lunar/planetary scientists will enable the development of a realistic strategy, fulfilling both scientific goals and enabling SRU. Further, an extensive core and ancillary technology development programme, including optimisation and performance evaluation, is required. This will, in turn, improve the design and development of robust SRU technologies whilst contributing invaluable knowledge to the scientific community.
Orlova, A., Nogueira, R. & Chimenti, P. The present and future of the space sector: a business ecosystem approach. Space Policy 52 , 101374 (2020).
Article Google Scholar
Malyh, D., Vaulin, S., Fedorov, V., Peshkov, R. & Shalashov, M. A brief review on in-orbit refueling projects and critical techniques. Aerospace Syst . 5 , 185–196 (2022).
Bobskill, M. R. et al. Preparing for Mars: evolvable mars campaign “proving ground” approach. In 2015 IEEE Aerospace Conference , 1–19 (IEEE, 2015).
Mueller, R. P., Sibille, L., Mantovani, J., Sanders, G. B. & Jones, C. A. Opportunities and strategies for testing and infusion of ISRU in the evolvable mars campaign. In AIAA SPACE 2015 Conference and Exposition , 4459 (AIAA, 2015).
Keaton, P. W. A Moon base/Mars base transportation depot. In Lunar Bases and Space Activities of the 21st Century (ed. Mendell, W. W.) 141–154 (Lunar and Planetary Institute, 1985).
Meurisse, A. & Carpenter, J. Past, present and future rationale for space resource utilisation. Planet. Space Sci. 182 , 104853 (2020).
Tsiolkovsky, K. The exploration of cosmic space by means of reaction motors. Sci. Rev. Mosc. St. Petersburg 221 , 222 (1903).
Google Scholar
Mazanek, D. D., Merrill, R. G., Brophy, J. R. & Mueller, R. P. Asteroid redirect mission concept: a bold approach for utilizing space resources. Acta Astronaut. 117 , 163–171 (2015).
Clarke, A. C. Electromagnetic launching as a major contribution to space flight. J. Br. Interplanet. Soc. 9 , 261–267 (1950).
Carr, B. B. Recovery of water or oxygen by reduction of lunar rock. AIAA J. 1 , 921–924 (1963).
McKay, M., McKay, D. & Duke, M. Space resources: NASA SP-509 (NASA, 1992).
Drake, B. G., Hoffman, S. J. & Beaty, D. W. Human exploration of Mars, design reference architecture 5.0. In 2010 IEEE Aerospace Conference , 1–24 (IEEE, 2010).
Sanders, G. B. & Larson, W. E. Progress made in lunar in-situ resource utilization under NASA’s Exploration Technology and Development Program. In Earth and Space (NASA, 2012).
Mueller, R. et al. Swamp works: a new approach to develop space mining and resource extraction technologies at the National Aeronautics Space Administration (NASA) Kennedy Space Center (KSC). In Future Mining Forum 2015 , KSC-E-DAA-TN26835 (NASA, 2015).
Dalton, C. & Hohmann, E. Conceptual design of a lunar colony (NASA, 1972).
Linne, D. L. et al. Lunar production system for extracting oxygen from regolith. J. Aerosp. Eng. 34 , 04021043 (2021).
Linne, D. L., Kleinhenz, J. E. & Paz, A. Lunar water pilot plant conceptual design. In ASCEND 2020 , 4236 (AIAA, 2020).
ESA. ESA Space Resources Strategy, https://tinyurl.com/yck54d6t (2019).
Hoffman, J. A. et al. Mars oxygen ISRU experiment (MOXIE)-Preparing for human Mars exploration. Sci. Adv. 8 , eabp8636 (2022).
Article CAS PubMed PubMed Central Google Scholar
Barber, S. et al. ProSPA: analysis of lunar polar volatiles and ISRU demonstration on the Moon. In 49th Lunar and Planetary Science Conference (Lunar and Planetary Institute, 2018).
Sanders, G., Kleinhenz, J. & Linne, D. NASA plans for in situ resource utilization (ISRU) development, demonstration, and implementation. In Committee on Space Research (COSPAR) 2022 (COSPAR, 2022).
NASA. NASA selects companies to collect lunar resources for Artemis, https://www.nasa.gov/press-release/nasa-selects-companies-to-collect-lunar-resources-for-artemis-demonstrations (2021).
ispace. ispace Announces Mission 1 Launch Date, https://ispace-inc.com/news-en/?p=3939 (2022).
Comstock, D. & Petro, A. Nasa’s centennial challenges contributions to ISRU. In 47th AIAA Aerospace Sciences Meeting including The New Horizons Forum and Aerospace Exposition , 1205 (AIAA, 2009).
Sacksteder, K. & Sanders, G. In-situ resource utilization for lunar and mars exploration. In 45th AIAA Aerospace Sciences Meeting and Exhibit , 345 (AIAA, 2007).
Agency, L. S. Space resources, https://space-agency.public.lu/en/space-resources.html (2022).
NASA. The Artemis Accords, https://www.nasa.gov/specials/artemis-accords/index.html (2022).
Government of the United States of America. United States Space Priorities Framework . Tech. Rep. (Government of the United States of America, Washington D.C., 2021). https://tinyurl.com/297a9r5j .
The Government of Luxembourg. Spaceresources.lu: New space law to provide framework for space resource utilisation, https://tinyurl.com/5n8y7b3w (2016).
Library of Congress. Japan: Space Resources Act Enacted, https://www.loc.gov/item/global-legal-monitor/2021-09-15/japan-space-resources-act-enacted/ (2021).
The Government of the United Arab Emirates. UAE National Space Policy . Tech. Rep. (The Government of the United Arab Emirates, Abu Dhabi, 2021). https://tinyurl.com/y5pa3awy .
UNOOSA. Working group on legal aspects of space resource activities. https://www.unoosa.org/oosa/en/ourwork/copuos/lsc/space-resources/index.html (2021).
Association, M. V. Report of the Moon Village Association on the Global Expert Group on Sustainable Lunar Activities. In Committee on the Peaceful Uses of Outer Space, Legal Subcommittee, Sixtieth Session (United Nations Office for Outer Space Affairs, Vienna, 2021).
Gilber, A. Mining in space is coming (Milken Review, 2021). https://www.milkenreview.org/articles/mining-in-space-is-coming .
Jakhu, R. S., Pelton, J. N. & Nyampong, Y. O. M. Private sector space mining initiatives and policies in the United States. In Space Mining and Its Regulation (Springer, Cham 2017).
Bridenstine, J. Space resources are the key to safe and sustainable lunar exploration, https://blogs.nasa.gov/bridenstine/2020/09/10/space-resources-are-the-key-to-safe-and-sustainable-lunar-exploration/ (2020).
Kleinhenz, J. E. & Paz, A. An ISRU propellant production system for a fully fueled Mars Ascent Vehicle. In 10th Symposium on Space Resource Utilization , 0423 (AIAA, 2017).
Lee, K. A., Oryshchyn, L., Paz, A., Reddington, M. & Simon, T. M. The ROxygen project: Outpost-scale lunar oxygen production system development at johnson space center. J. Aerosp. Eng. 26 , 67–73 (2013).
Grill, L., Ostermeier, P., Würth, M. & Reiss, P. Behaviour of lunar regolith simulants in fluidised bed reactors for in-situ resource utilisation. Planet. Space Sci. 180 , 104757 (2020).
Article CAS Google Scholar
Schwandt, C., Hamilton, J. A., Fray, D. J. & Crawford, I. A. The production of oxygen and metal from lunar regolith. Planet. Space Sci. 74 , 49–56 (2012).
Lomax, B. A. et al. Proving the viability of an electrochemical process for the simultaneous extraction of oxygen and production of metal alloys from lunar regolith. Planet. Space Sci. 180 , 104748 (2020).
Allen, C. C., Morris, R. V. & McKay, D. S. Oxygen extraction from lunar soils and pyroclastic glass. J. Geophys. Res. Planets 101 , 26085–26095 (1996).
Sirk, A. H., Sadoway, D. R. & Sibille, L. Direct electrolysis of molten lunar regolith for the production of oxygen and metals on the moon. ECS Trans. 28 , 367 (2010).
Shaw, M. et al. Mineral processing and metal extraction on the lunar surface-challenges and opportunities. Miner. Process. Extractive Metall. Rev. 43 , 865–891 (2022).
Lu, Y. & Reddy, R. G. Extraction of metals and oxygen from lunar soil. High. Temp. Mater. Process. 27 , 223–234 (2008).
Gibson, M. A. & Knudsen, C. W. Apparatus for manufacture of oxygen from lunar ilmenite. US Patent 5,536,378 (1996).
Sowers, G. F. & Dreyer, C. B. Ice mining in lunar permanently shadowed regions. N. Space 7 , 235–244 (2019).
Kornuta, D. et al. Commercial lunar propellant architecture: a collaborative study of lunar propellant production. Reach 13 , 100026 (2019).
Blair, B. R. et al. Space resource economic analysis toolkit: the case for commercial lunar ice mining . Final report to the NASA Exploration Team (NASA, 2002).
Cannon, K. M. & Britt, D. T. A geologic model for lunar ice deposits at mining scales. Icarus 347 , 113778 (2020).
Brisset, J., Miletich, T. & Metzger, P. Thermal extraction of water ice from the lunar surface-A 3D numerical model. Planet. Space Sci. 193 , 105082 (2020).
Jedicke, R., Sercel, J., Gillis-Davis, J., Morenz, K. J. & Gertsch, L. Availability and delta-v requirements for delivering water extracted from near-Earth objects to cis-lunar space. Planet. Space Sci. 159 , 28–42 (2018).
Sonter, M. Near earth objects as resources for space industrialization. Sol. Syst. Dev. J. 1 , 1–31 (2001).
Zuppero, A. C. & Jacox, M. G. Near earth object fuels (neo-fuels): discovery, prospecting and use. In 43rd Congress of the International Astronautical Federation (IAF, 1992).
Sanchez, J.-P. & McInnes, C. Asteroid resource map for near-Earth space. J. Spacecr. Rockets 48 , 153–165 (2011).
Shoemaker, E. & Helin, E. Earth-approaching asteroids as targets for exploration (NASA, 1978).
Sonter, M. J. The technical and economic feasibility of mining the near-earth asteroids. Acta Astronaut. 41 , 637–647 (1997).
Gaffey, M. J. & Mccord, T. B. Asteroids: a source of natural resources for terrestrial and extra-terrestrial applications. In Abstracts of Papers Presented at a Special Session of the Seventh Annual Lunar Science Conference on Utilization of Lunar Materials and Expertise for Large Scale Operations in Space (Lunar Science Institute, 1976).
Jakus, A. E., Koube, K. D., Geisendorfer, N. R. & Shah, R. N. Robust and elastic lunar and martian structures from 3D-printed regolith inks. Sci. Rep. 7 , 1–8 (2017).
Goulas, A., Engstrøm, D. S. & Friel, R. J. Additive manufacturing using space resources. In Additive manufacturing , 661–683 (Elsevier, 2021).
Mueller, R. P. et al. Additive construction using basalt regolith fines. In Earth and Space 2014: Engineering for Extreme Environments (eds. Gertsch, L. S. & Malla, R. B.) 394–403 (ASCE, 2014).
Isachenkov, M., Chugunov, S., Akhatov, I. & Shishkovsky, I. Regolith-based additive manufacturing for sustainable development of lunar infrastructure–an overview. Acta Astronaut. 180 , 650–678 (2021).
Fateri, M. et al. Solar sintering for lunar additive manufacturing. J. Aerosp. Eng. 32 , 04019101 (2019).
Altun, A. A. et al. Additive manufacturing of lunar regolith structures. Open Ceram. 5 , 100058 (2021).
Reitz, B. et al. Additive manufacturing under lunar gravity and microgravity. Microgravity Sci. Technol. 33 , 1–12 (2021).
Labeaga-Martínez, N., Sanjurjo-Rivo, M., Díaz-Álvarez, J. & Martínez-Frías, J. Additive manufacturing for a Moon village. Procedia Manuf. 13 , 794–801 (2017).
Zocca, A. et al. Challenges in the technology development for additive manufacturing in space. Chin. J. Mech. Eng. Additive Manuf. Front . 1 , 100018 (2022).
Goulas, A., Harris, R. A. & Friel, R. J. Additive manufacturing of physical assets by using ceramic multicomponent extra-terrestrial materials. Addit. Manuf. 10 , 36–42 (2016).
Ulubeyli, S. Lunar shelter construction issues: the state-of-the-art towards 3D printing technologies. Acta Astronaut. 195 , 318–343 (2022).
Goulas, A., Binner, J. G., Harris, R. A. & Friel, R. J. Assessing extraterrestrial regolith material simulants for in-situ resource utilisation based 3D printing. Appl. Mater. Today 6 , 54–61 (2017).
Hogue, M. D., Mueller, R. P., Sibille, L., Hintze, P. E. & Rasky, D. J. Extraterrestrial regolith derived atmospheric entry heat shields. In Earth and Space 2016: Engineering for Extreme Environments (eds. Malla, R. B., Agui, J. H. & van Susante, P. J.) 699–712 (ASCE, 2016).
Hogue, M. D., Meuller, R. P., Sibille, L., Hintze, P. E. & Rasky, D. J. Regolith derived heat shield for planetary body entry and descent system with in situ fabrication . Tech. Rep., (NASA, 2012).
Horton, C. et al. First demonstration of photovoltaic diodes on lunar regolith-based substrate. Acta Astronaut. 56 , 537–545 (2005).
Waldron, R. Lunar manufacturing: a survey of products and processes. Acta Astronaut. 17 , 691–708 (1988).
Ellery, A. Generating and storing power on the moon using in situ resources. Proc. Inst. Mech. Eng. Part G J. Aerosp. Eng. 236 , 1045–1063 (2022).
Freundlich, A. et al. Manufacture of solar cells on the Moon. In Conference Record of the Thirty-first IEEE Photovoltaic Specialists Conference, 2005 ., 794–797 (IEEE, 2005).
Cilliers, J., Rasera, J. & Hadler, K. Estimating the scale of space resource utilisation (SRU) operations to satisfy lunar oxygen demand. Planet. Space Sci. 180 , 104749 (2020).
Hadler, K. et al. A universal framework for space resource utilisation (SRU). Planet. Space Sci . 182 , 104811 (2020).
Sour Gertsch, L. & Gertsch, R. E. Surface mine design and planning for lunar regolith production , vol. 654, 1108–1115 (American Institute of Physics, 2003).
Casanova, S. et al. Lunar polar water resource exploration–Examination of the lunar cold trap reservoir system model and introduction of play-based exploration (PBE) techniques. Planet. Space Sci. 180 , 104742 (2020).
Espejel, C. D. Lunar ore reserves standards 101 (LORS-101), a first code for the reporting of lunar exploration results, lunar resources, and lunar reserves. In Fourth International Future Mining Conference (AusIMM, 2019).
Luchsinger, K. M., Chanover, N. J. & Strycker, P. D. Water within a permanently shadowed lunar crater: Further LCROSS modeling and analysis. Icarus 354 , 114089 (2021).
Just, G., Smith, K., Joy, K. & Roy, M. Parametric review of existing regolith excavation techniques for lunar in situ resource utilisation (ISRU) and recommendations for future excavation experiments. Planet. Space Sci. 180 , 104746 (2020).
Taylor, L. A. & Carrier III, W. D. Production of oxygen on the Moon: which processes are best and why. AIAA J. 30 , 2858–2863 (1992).
Rasera, J., Cilliers, J., Lamamy, J. & Hadler, K. The beneficiation of lunar regolith for space resource utilisation: a review. Planet. Space Sci. 186 , 104879 (2020).
Taylor, G. J., Neubert, J., Lucey, P. & McCullough, E. The uncertain nature of polar lunar regolith. In Space Resources Roundtable VI , 45 (Lunar and Planetary Institute, 2004).
Taylor, S. R. Planetary science: a lunar perspective , vol. 3303 (Lunar and Planetary Institute Houston, 1982).
Ohtake, M. et al. Geologic structure generated by large-impact basin formation observed at the south pole-aitken basin on the moon. Geophys. Res. Lett. 41 , 2738–2745 (2014).
Vaniman, D., Dietrich, J., Taylor, G. J. & Heiken, G. Exploration, samples, and recent concepts of the Moon , 5–26 (Cambridge University Press Cambridge, 1991).
Jayathilake, B., Ilankoon, I. & Dushyantha, M. Assessment of significant geotechnical parameters for lunar regolith excavations. Acta Astronaut. 196 , 107–122 (2022).
Kawamoto, H. & Miwa, T. Mitigation of lunar dust adhered to mechanical parts of equipment used for lunar exploration. J. Electrost. 69 , 365–369 (2011).
Carpenter, J. D., Fisackerly, R., De Rosa, D. & Houdou, B. Scientific preparations for lunar exploration with the european lunar lander. Planet. Space Sci. 74 , 208–223 (2012).
Christoffersen, R. & Lindsay, J. F. Lunar dust effects on spacesuit systems: insights from the apollo spacesuits . Tech. Rep. (NASA, 2009).
Afshar-Mohajer, N., Wu, C.-Y., Curtis, J. S. & Gaier, J. R. Review of dust transport and mitigation technologies in lunar and martian atmospheres. Adv. Space Res. 56 , 1222–1241 (2015).
Taylor, L. A., Pieters, C. M. & Britt, D. Evaluations of lunar regolith simulants. Planet. Space Sci. 126 , 1–7 (2016).
Liu, Y. & Taylor, L. A. Characterization of lunar dust and a synopsis of available lunar simulants. Planet. Space Sci. 59 , 1769–1783 (2011).
Toklu, Y. C. & Akpinar, P. Lunar soils, simulants and lunar construction materials: an overview. Adv. Space Res. 70 , 762–779 (2022).
Sun, H., Yi, M., Shen, Z., Zhang, X. & Ma, S. Developing a new controllable lunar dust simulant: Bhld20. Planet. Space Sci. 141 , 17–24 (2017).
Kosick, G., Bennett, C. & Dobby, G. Managing company risk by incorporating the mine resource model into design and optimization of mineral processing plants . Tech. Rep., (SGS Mineral Services, 2002).
Sargeant, H. M. et al. Hydrogen reduction of lunar samples in a static system for a water production demonstration on the moon. Planet. Space Sci. 205 , 105287 (2021).
Download references
Acknowledgements
The authors would like to thank the European Space Agency (ESA) for the opportunity to contribute to the SciSpacE White Paper exercise, as well as for supporting this submission to the special issue of npj Microgravity. We would also like to thank the ESA Topical Team on ‘A complete resource production flowsheet for lunar materials’, funded by ESA Contract 4000123986/18/NL/PG.
Author information
These authors contributed equally: Jan Cilliers, Kathryn Hadler, Joshua Rasera.
Authors and Affiliations
Department of Earth Science and Engineering, Imperial College London, Exhibition Road, London, SW7 2AZ, United Kingdom
Jan Cilliers, Kathryn Hadler & Joshua Rasera
European Space Resources Innovation Centre (ESRIC), Luxembourg Institute of Science and Technology (LIST), Maison de l’Innovation, 5, avenue des Hauts-Fourneuax, Esch-sur-Alzette, L-4362, Luxembourg
Kathryn Hadler
You can also search for this author in PubMed Google Scholar
Contributions
J.J.C. and K.H. were responsible for developing the process background, the gap analysis, and identification of open questions, revising the paper, and general editing. J.N.R. was responsible for developing the introduction, the literature review, synthesis of literature and gaps/open questions, revising the paper structure, and general editing.
Corresponding author
Correspondence to Jan Cilliers .
Ethics declarations
Competing interests.
The authors declare no competing interests.
Additional information
Publisher’s note Springer Nature remains neutral with regard to jurisdictional claims in published maps and institutional affiliations.
Rights and permissions
Open Access This article is licensed under a Creative Commons Attribution 4.0 International License, which permits use, sharing, adaptation, distribution and reproduction in any medium or format, as long as you give appropriate credit to the original author(s) and the source, provide a link to the Creative Commons license, and indicate if changes were made. The images or other third party material in this article are included in the article’s Creative Commons license, unless indicated otherwise in a credit line to the material. If material is not included in the article’s Creative Commons license and your intended use is not permitted by statutory regulation or exceeds the permitted use, you will need to obtain permission directly from the copyright holder. To view a copy of this license, visit http://creativecommons.org/licenses/by/4.0/ .
Reprints and permissions
About this article
Cite this article.
Cilliers, J., Hadler, K. & Rasera, J. Toward the utilisation of resources in space: knowledge gaps, open questions, and priorities. npj Microgravity 9 , 22 (2023). https://doi.org/10.1038/s41526-023-00274-3
Download citation
Received : 03 August 2022
Accepted : 13 March 2023
Published : 25 March 2023
DOI : https://doi.org/10.1038/s41526-023-00274-3
Share this article
Anyone you share the following link with will be able to read this content:
Sorry, a shareable link is not currently available for this article.
Provided by the Springer Nature SharedIt content-sharing initiative
This article is cited by
Cryogenic propellant management in space: open challenges and perspectives.
- Alessia Simonini
- Michael Dreyer
- Jean-Baptiste Gouriet
npj Microgravity (2024)
Quick links
- Explore articles by subject
- Guide to authors
- Editorial policies
Sign up for the Nature Briefing newsletter — what matters in science, free to your inbox daily.

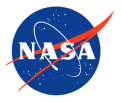

Suggested Searches
- Climate Change
- Expedition 64
- Mars perseverance
- SpaceX Crew-2
- International Space Station
- View All Topics A-Z
Humans in Space
Earth & climate, the solar system, the universe, aeronautics, learning resources, news & events.
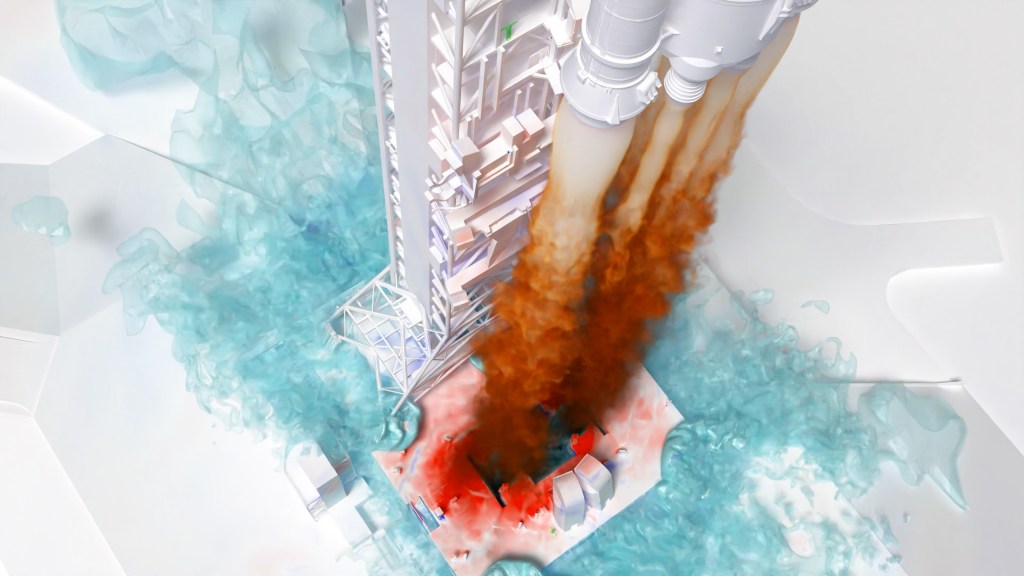
Six Ways Supercomputing Advances Our Understanding of the Universe
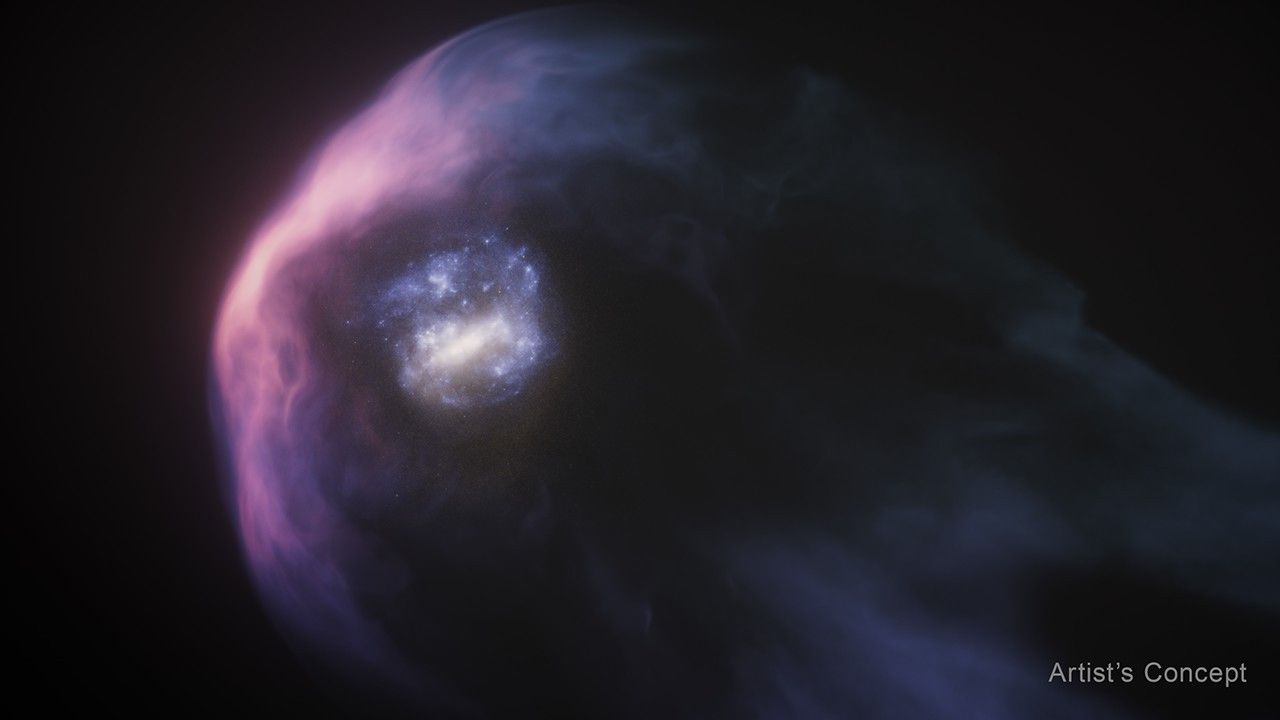
NASA’s Hubble Sees Aftermath of Galaxy’s Scrape with Milky Way
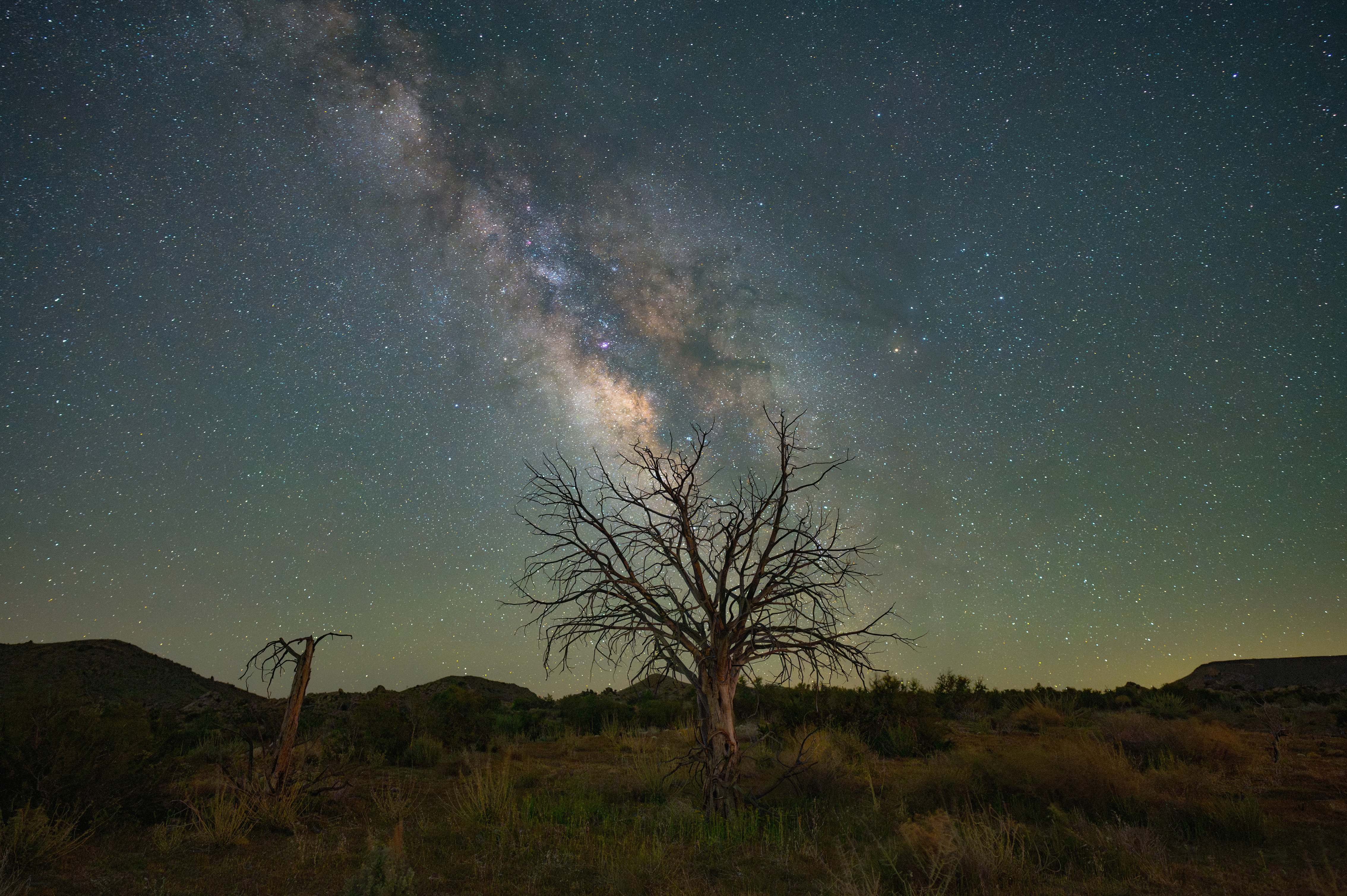
What’s Up: November 2024 Skywatching Tips from NASA
- Search All NASA Missions
- A to Z List of Missions
- Upcoming Launches and Landings
- Spaceships and Rockets
- Communicating with Missions
- James Webb Space Telescope
- Hubble Space Telescope
- Why Go to Space
- Commercial Space
Destinations
Living in Space
- Explore Earth Science
- Earth, Our Planet
- Earth Science in Action
- Earth Multimedia
- Earth Science Researchers
- Pluto & Dwarf Planets
- Asteroids, Comets & Meteors
- The Kuiper Belt
- The Oort Cloud
- Skywatching
- The Search for Life in the Universe
- Black Holes
- The Big Bang
- Dark Energy & Dark Matter
- Earth Science
- Planetary Science
- Astrophysics & Space Science
- The Sun & Heliophysics
- Biological & Physical Sciences
- Lunar Science
- Citizen Science
- Astromaterials
- Aeronautics Research
- Human Space Travel Research
- Science in the Air
- NASA Aircraft
- Flight Innovation
- Supersonic Flight
- Air Traffic Solutions
- Green Aviation Tech
- Drones & You
- Technology Transfer & Spinoffs
- Space Travel Technology
- Technology Living in Space
- Manufacturing and Materials
- Science Instruments
- For Kids and Students
- For Educators
- For Colleges and Universities
- For Professionals
- Science for Everyone
- Requests for Exhibits, Artifacts, or Speakers
- STEM Engagement at NASA
- NASA's Impacts
- Centers and Facilities
- Directorates
- Organizations
- People of NASA
- Internships
- Our History
- Doing Business with NASA
- Get Involved
NASA en Español
- Aeronáutica
- Ciencias Terrestres
- Sistema Solar
- All NASA News
- Video Series on NASA+
- Newsletters
- Social Media
- Media Resources
- Upcoming Launches & Landings
- Virtual Guest Program
- Image of the Day
- Sounds and Ringtones
- Interactives
- STEM Multimedia

Hubble Takes a Look at Tangled Galaxies
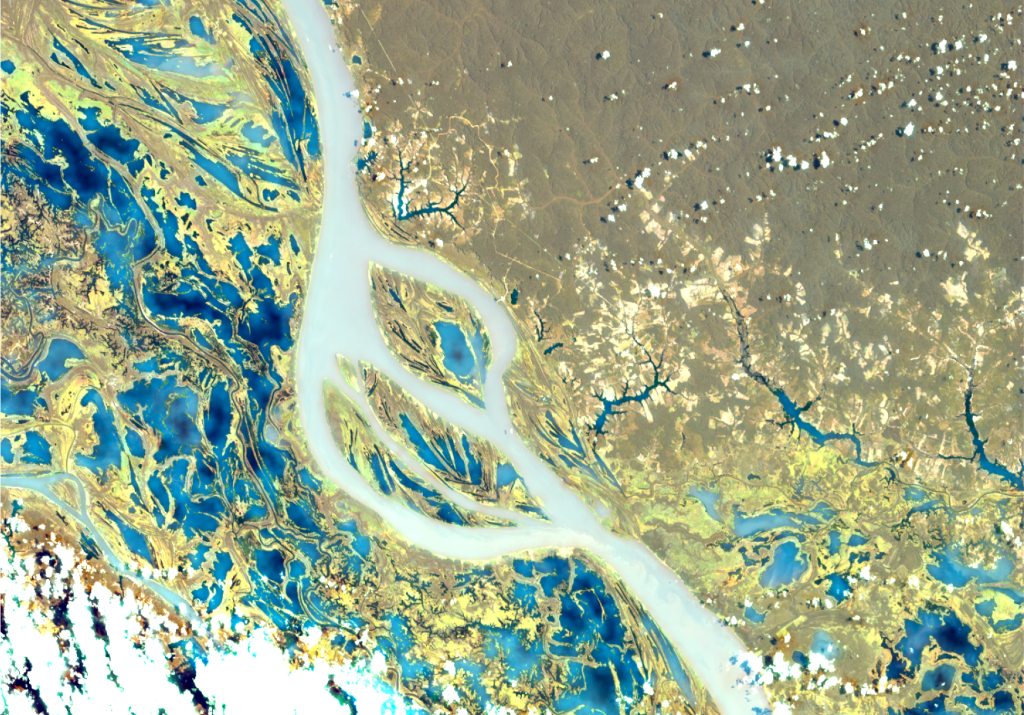
NASA’s EMIT Will Explore Diverse Science Questions on Extended Mission

Astronomers Find Early Fast-Feeding Black Hole Using NASA Telescopes
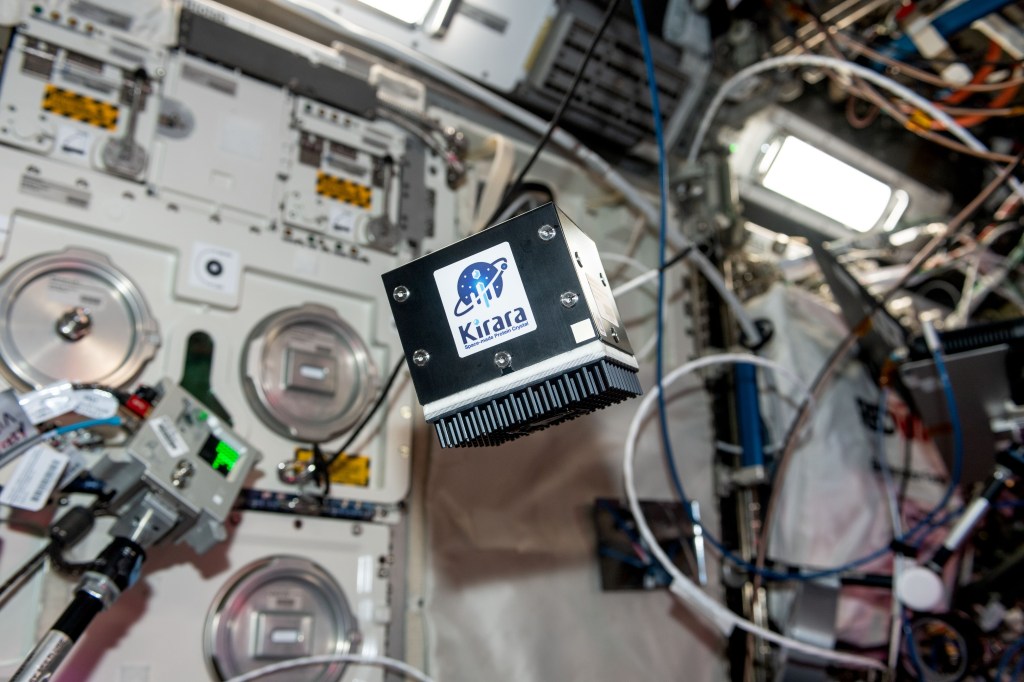
Station Science Top News: Nov. 15, 2024

Wearable Tech for Space Station Research

Station Science Top News: Nov. 8, 2024
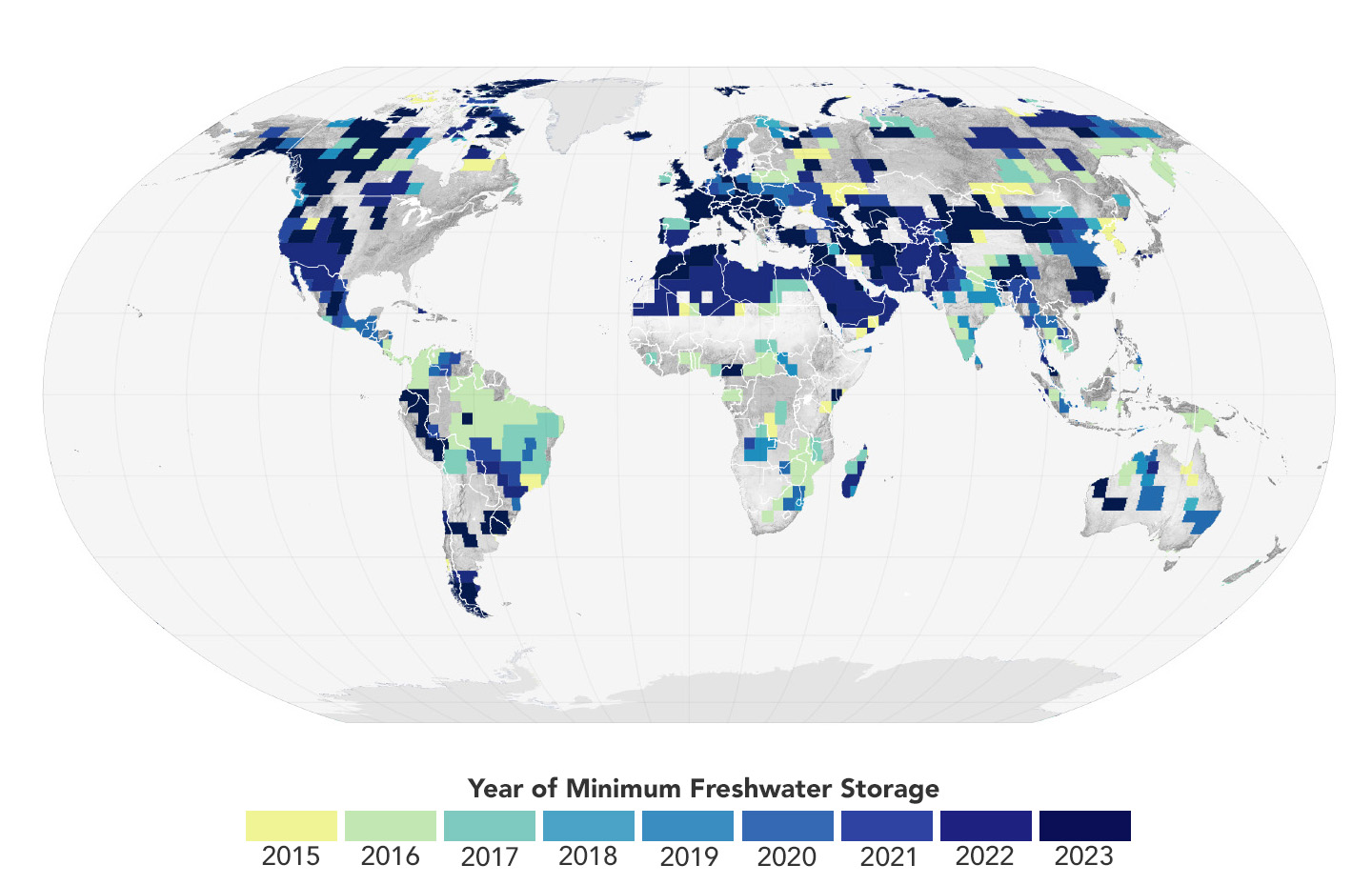
NASA Satellites Reveal Abrupt Drop in Global Freshwater Levels

NASA Data Helps International Community Prepare for Sea Level Rise

NASA’s Curiosity Mars Rover Takes a Last Look at Mysterious Sulfur
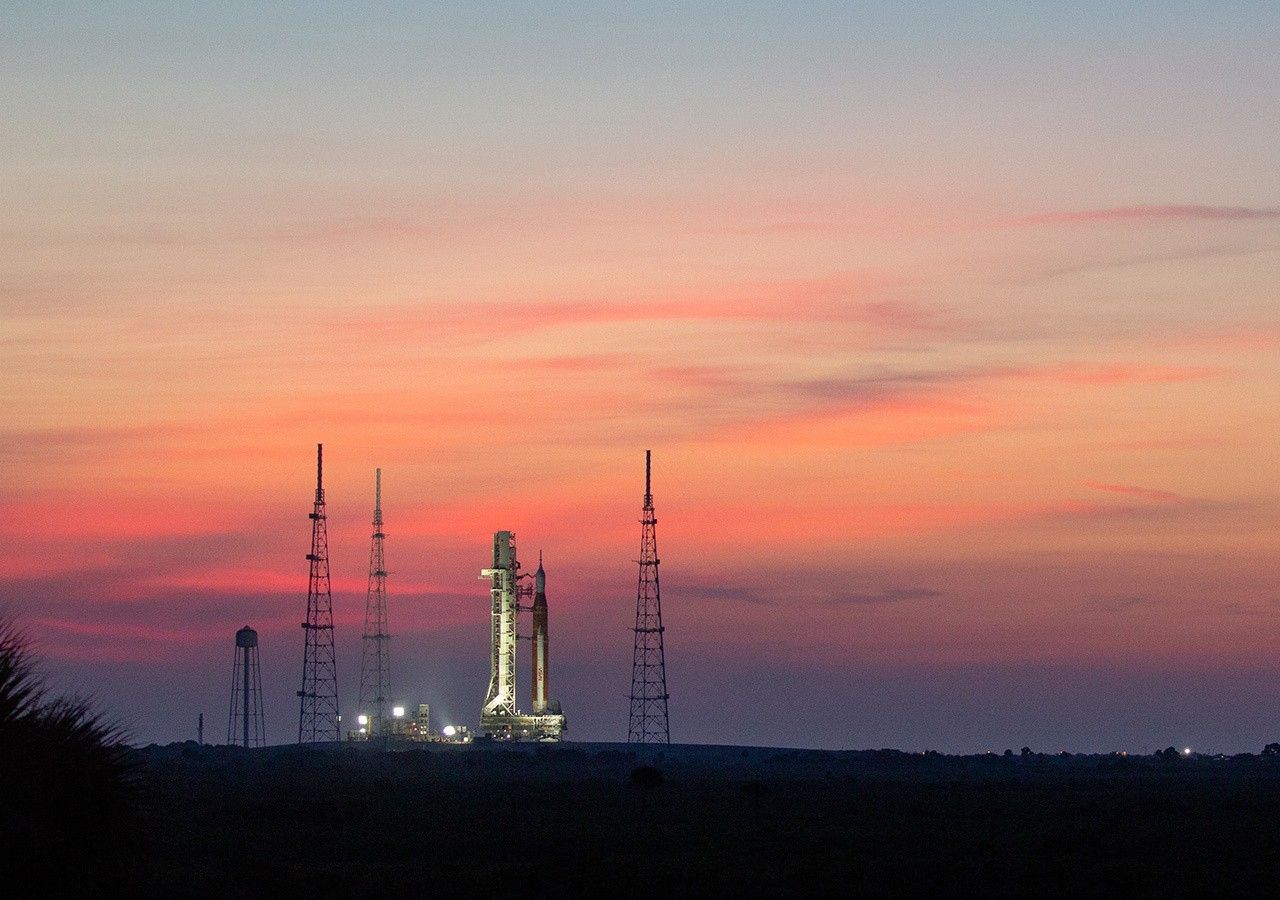
Amendment 68: F.12 Artemis IV Deployed Instruments Program Final Text and Due Dates Released.
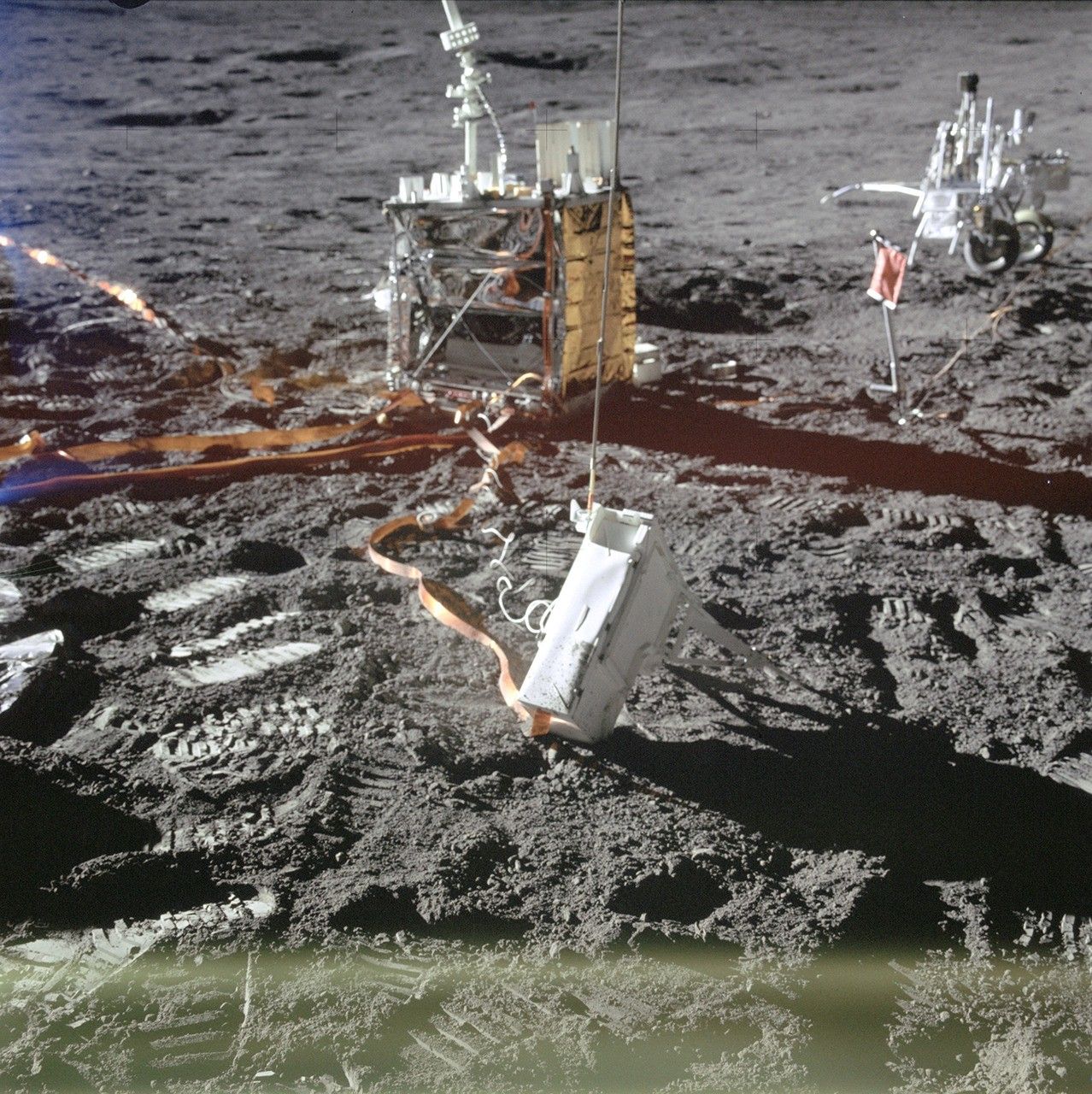
Amendment 69: DRAFT A.59 New or Modified GLOBE Protocols for Community Comment

Table 1 of ROSES-24 and the ROSES-2024 Summary of Solicitation Updated to Include Link to Grants Policy Guidance on Proposal Requirements.

NASA Electric Aircraft Testbed (NEAT)
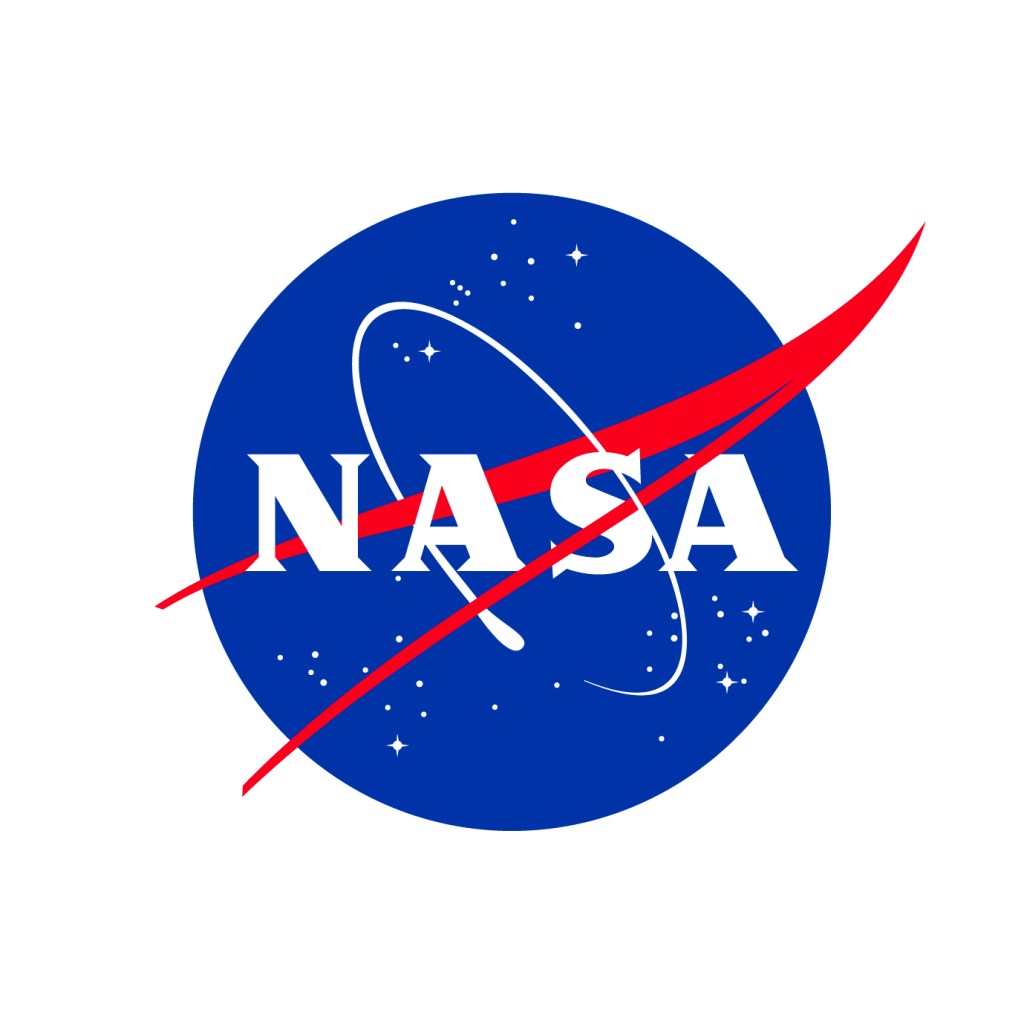
Dynamic Spin Rig Publications

NASA Program Aids Pediatric Patients Facing Medical Treatments

NASA Goddard Lidar Team Receives Center Innovation Award for Advancements
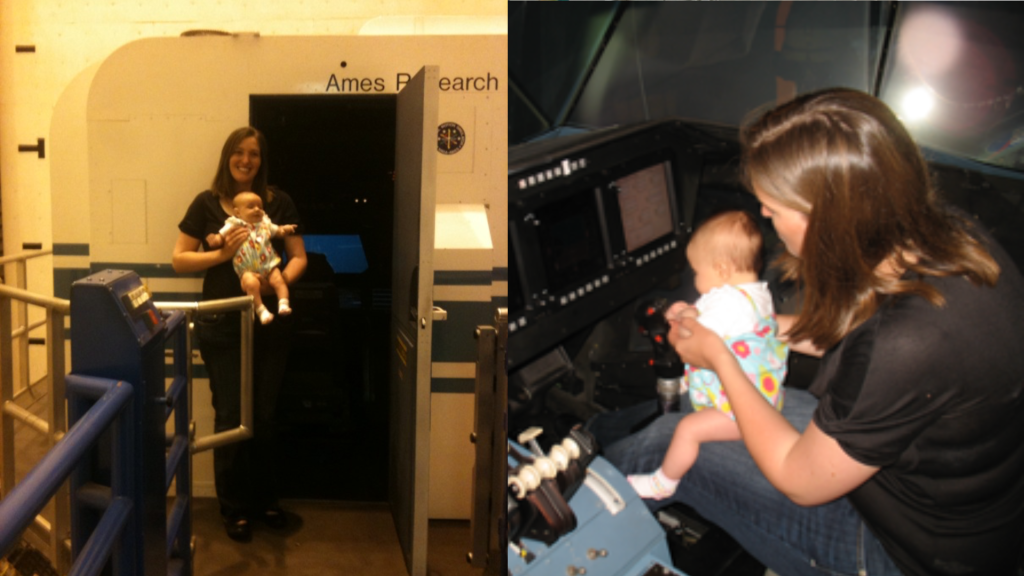
Math, Mentorship, Motherhood: Behind the Scenes with NASA Engineers

Student-Built Capsules Endure Heat of Re-entry for NASA Science
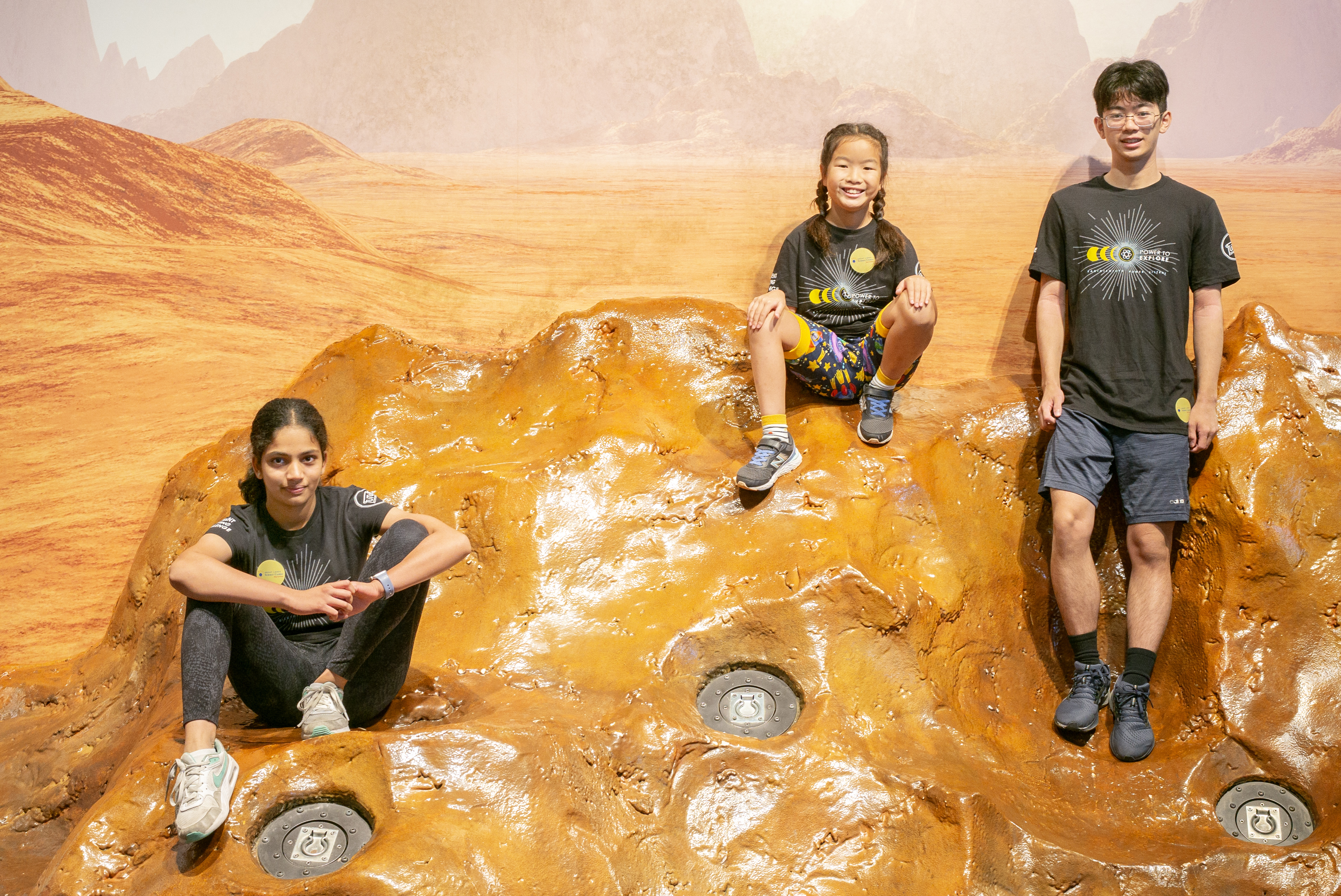
Power to Explore STEM Writing Challenge
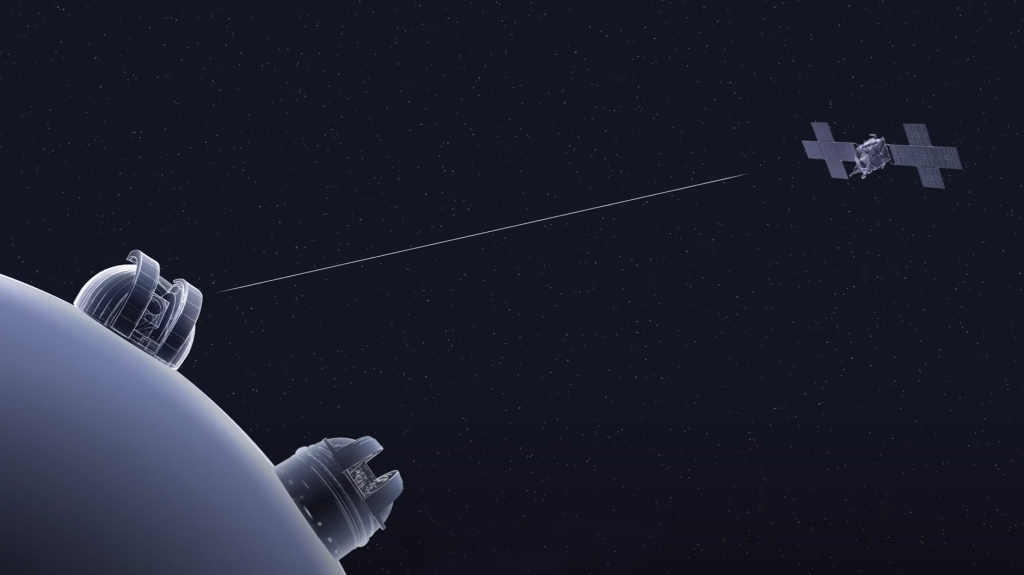
Precision Pointing Goes the Distance on NASA Experiment
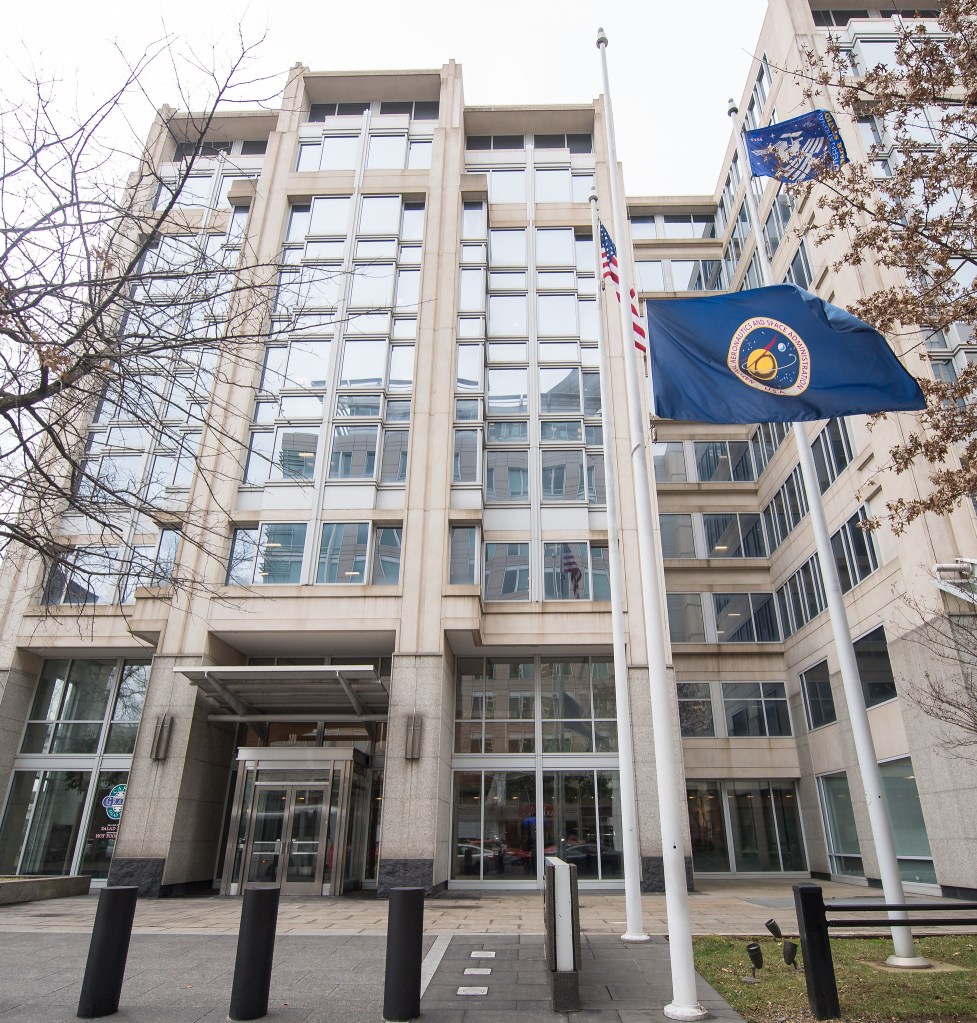
NASA Seeks Options for Future Headquarters Building
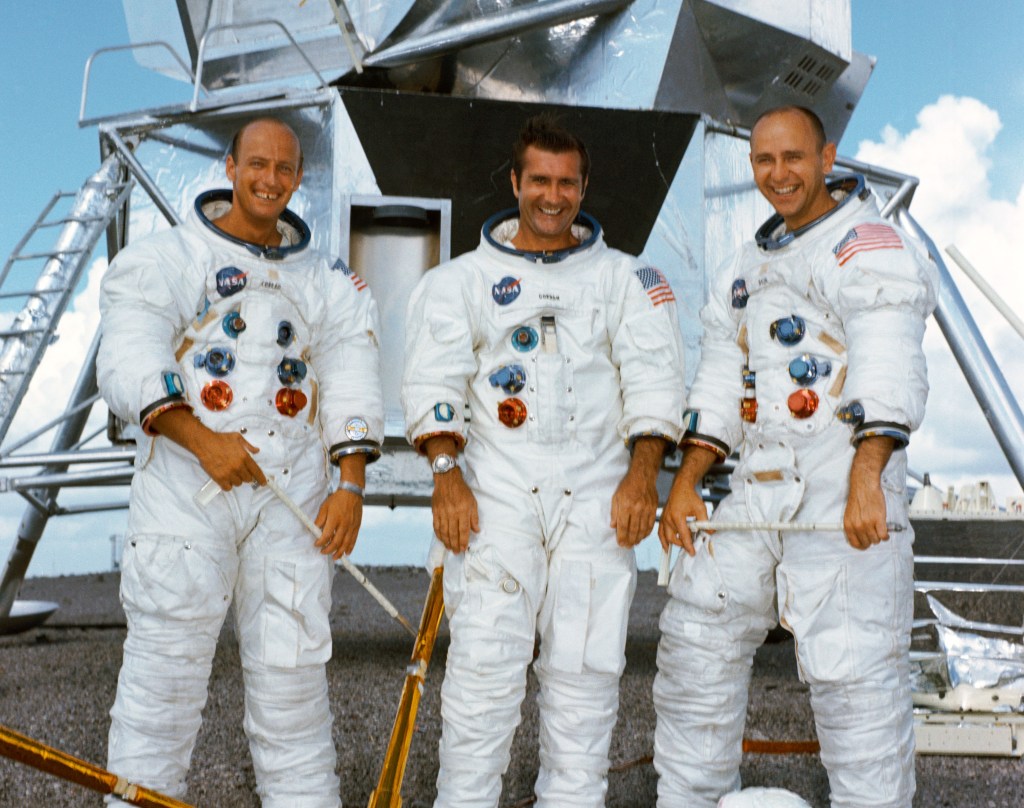
55 Years Ago: Apollo 12 Makes a Pinpoint Landing on the Moon
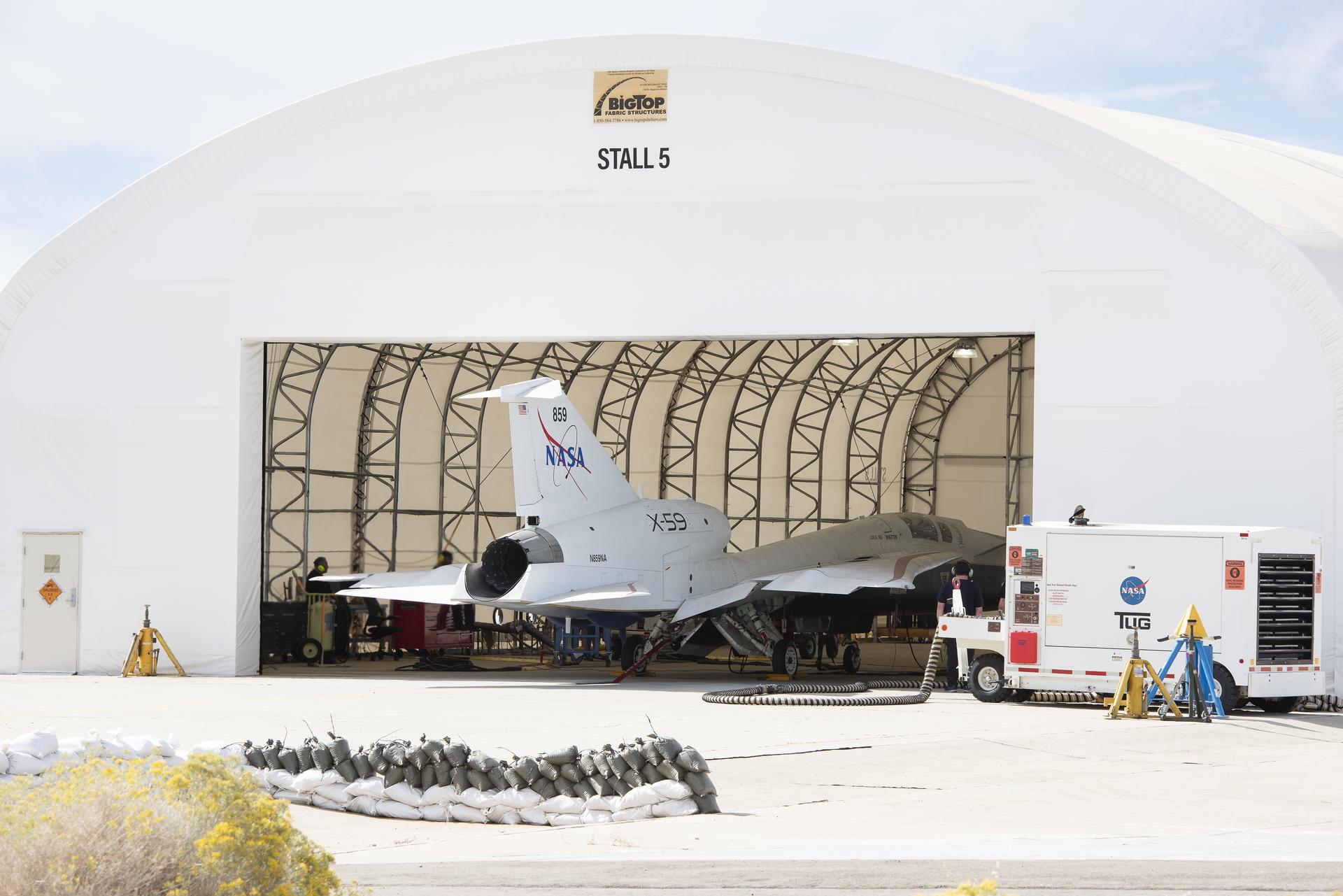
El X-59 enciende su motor por primera vez rumbo al despegue
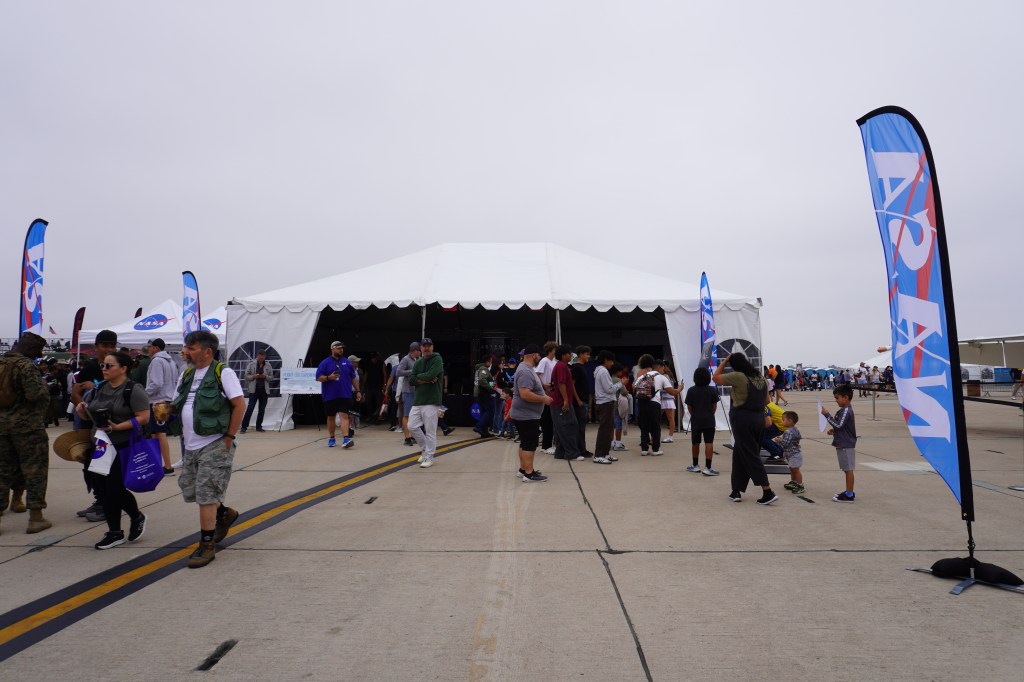
La NASA lleva un dron y un rover espacial a un espectáculo aéreo
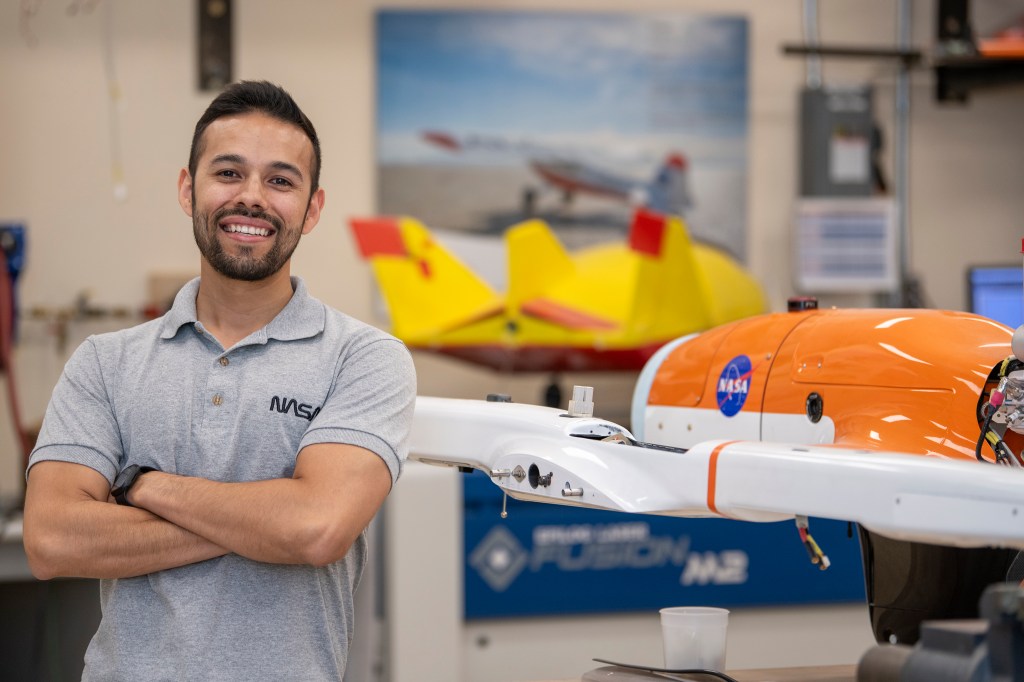
Destacado de la NASA: Felipe Valdez, un ingeniero inspirador
Why go to space.
The reasons to explore the universe are as vast and varied as the reasons to explore the forests, the mountains, or the sea. Since the dawn of humanity, people have explored to learn about the world around them, find new resources, and improve their existence.
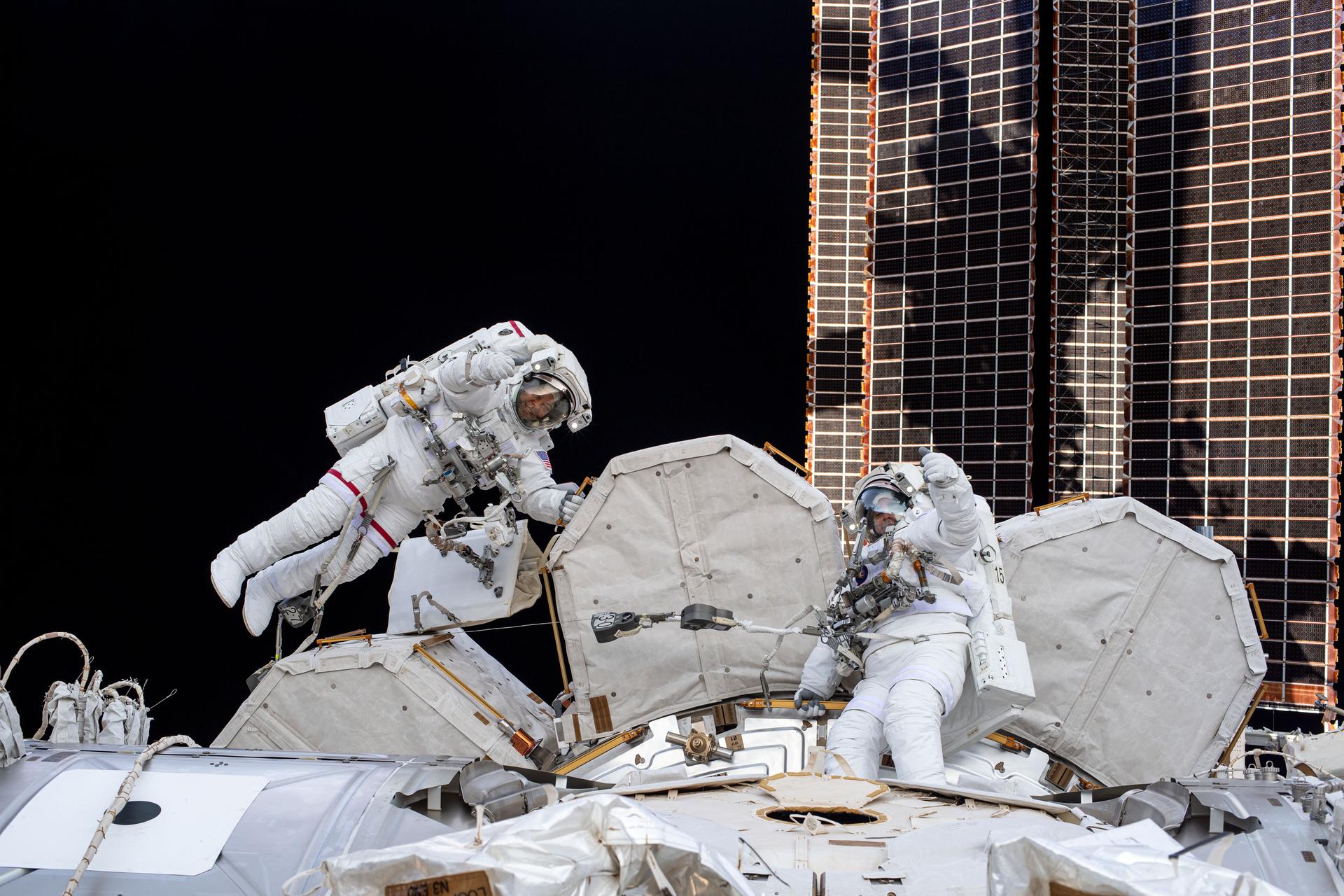
Why We Go to Space
At NASA, we explore the secrets of the universe for the benefit of all, creating new opportunities and inspiring the world through discovery.
NASA’s exploration vision is anchored in providing value for humanity by answering some of the most fundamental questions: Why are we here? How did it all begin? Are we all alone? What comes next? And, as an addendum to that: How can we make our lives better?
NASA was created more than half a century ago to begin answering some of these questions. Since then, space exploration has been one of the most unifying, borderless human endeavors to date. An international partnership of five space agencies from 15 countries operates the International Space Station, and two dozen countries have signed the Artemis Accords, signaling their commitment to shared values for long-term human exploration and research at the Moon. Through space exploration, we gain a new perspective to study Earth and the solar system. We advance new technologies that improve our daily lives, and we inspire a new generation of artists, thinkers, tinkerers, engineers, and scientists.
Benefits to Humanity
Space exploration unites the world to inspire the next generation, make ground-breaking discoveries, and create new opportunities.
Technologies and missions we develop for human spaceflight have thousands of applications on Earth, boosting the economy, creating new career paths, and advancing everyday technologies all around us.
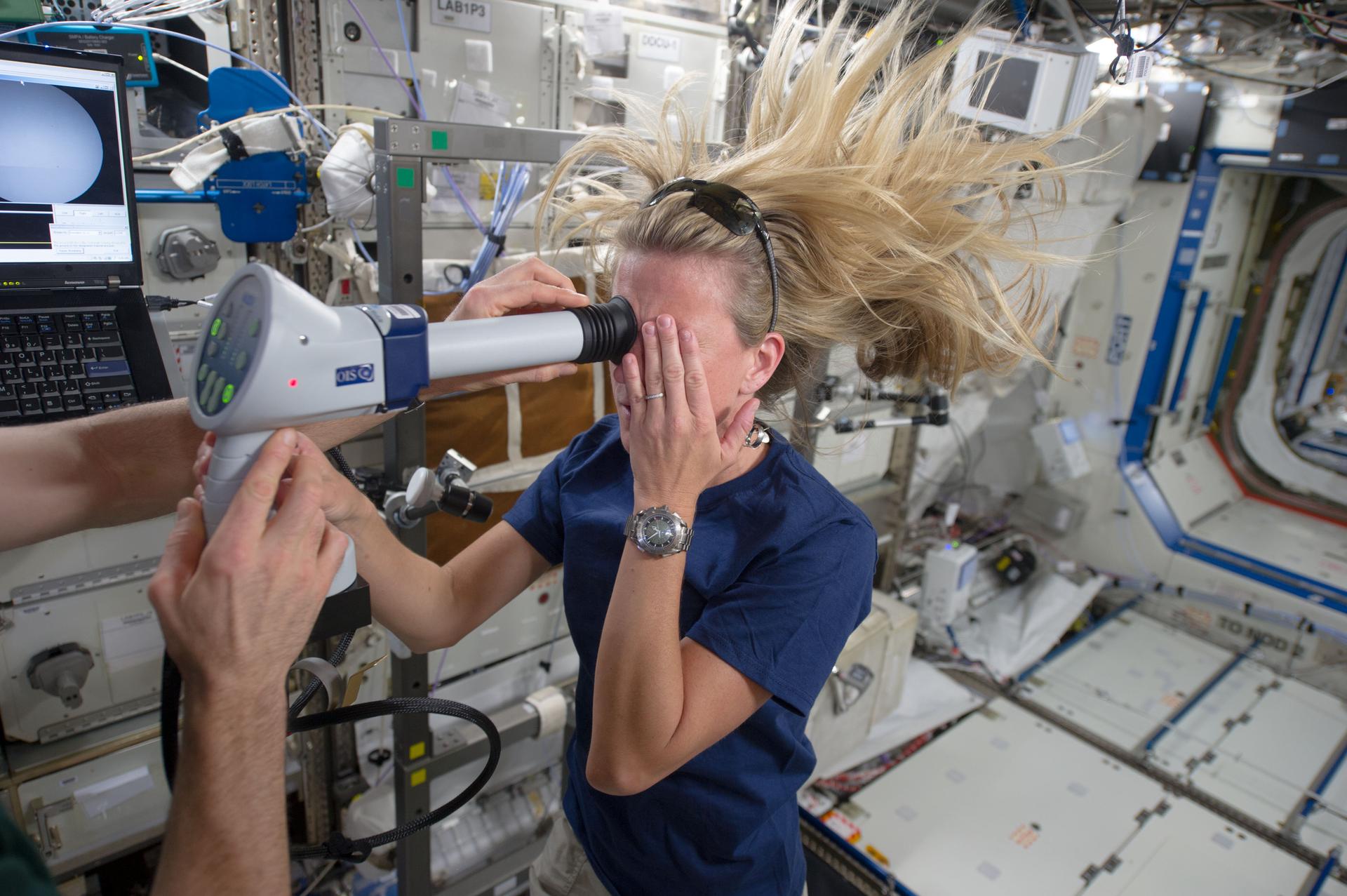
To view this video please enable JavaScript, and consider upgrading to a web browser that supports HTML5 video
Benefits to Science
The pursuit of discovery drives NASA to develop missions that teach us about Earth, the solar system, and the universe around us.
Science at NASA answers questions as practical as hurricane formation, as enticing as the prospect of lunar resources, as surprising as behavior in weightlessness, and as profound as the origin of the Universe.
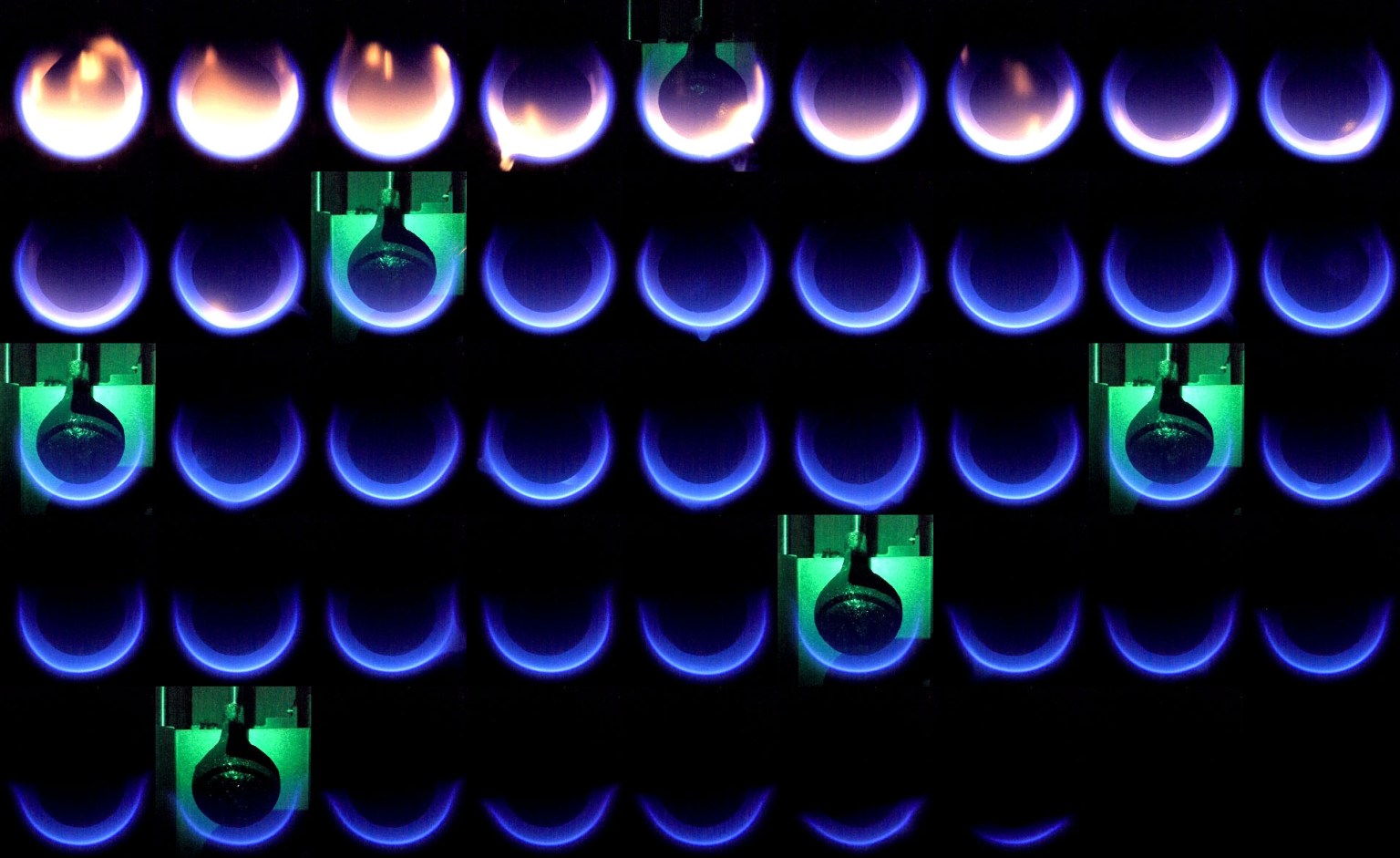
Unite with us on our journey to explore.

Discover More Topics From NASA
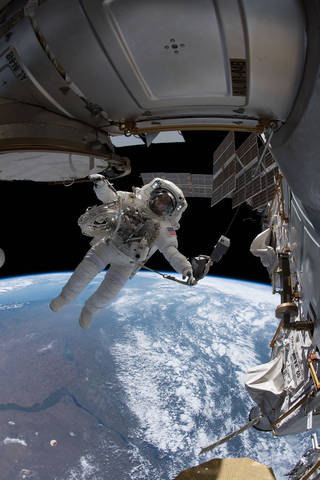

IMAGES
COMMENTS
With recent advances in commercial space exploration, we have curated a list of our best Research Topics on outer space. Explore collections edited by experts from NASA, The Goddard Space Flight Center, Space Science Institute, German Aerospace Center, Canadian Space Agency, National Space Science Center, European Space Agency, International Space University, and many more.
The space shuttle's formal name was the Space Transportation System (STS). It was used to orbit Earth, where its crew could do scientific work, place satellites in orbit, and visit space stations. Usually five to seven crew members rode the space shuttles, which were launched from Kennedy Space Center in Florida. Six shuttles were built.
Explore the latest questions and answers in Space Exploration, and find Space Exploration experts. ... I did some research in 2009/2010 for a star tracker that would have used COTS electronics and ...
The next phase of human space exploration includes the establishment and habitation of a lunar gateway, a permanent base on the Moon's surface, and exploratory crewed missions to Mars.
Research Topics List. JPL's charter is to conduct robotic space missions for NASA, to explore our own and neighboring planetary systems, understand the origin and evolution of the universe and make critical measurements to understand our home planet and help protect it. ... Astrophysics and space science research efforts at JPL are charged with ...
The research at the Center for Astrophysics | Harvard & Smithsonian centers on humanity's greatest unresolved questions about the nature of the universe. These questions have been pondered for generations, and our research is bringing us closer to answers than ever before ...
The use of space resources to provide propellant, habitation and materials critical to support human life (e.g. water, oxygen) will unlock the full potential of space exploration, enabling humans ...
Space exploration, investigation, by means of crewed and uncrewed spacecraft, of the reaches of the universe beyond Earth's atmosphere and the use of the information so gained to increase knowledge of the cosmos and benefit humanity. Learn more about space exploration in this article.
Why it matters: This colloid research can help to improve products used in our everyday lives and create entirely new products, including some that could enable exploration farther into space. Companies such as Procter & Gamble have used station research to study how to keep a product liquid enough to dispense easily and yet prevent ingredients ...
An international partnership of five space agencies from 15 countries operates the International Space Station, and two dozen countries have signed the Artemis Accords, signaling their commitment to shared values for long-term human exploration and research at the Moon. Through space exploration, we gain a new perspective to study Earth and the ...