
- ASSIGNMENTS
- Program Home Page
- LIBRARY RESOURCES

Case Study: The Amazon Rainforest

The Amazon in context
Tropical rainforests are often considered to be the “cradles of biodiversity.” Though they cover only about 6% of the Earth’s land surface, they are home to over 50% of global biodiversity. Rainforests also take in massive amounts of carbon dioxide and release oxygen through photosynthesis, which has also given them the nickname “lungs of the planet.” They also store very large amounts of carbon, and so cutting and burning their biomass contributes to global climate change. Many modern medicines are derived from rainforest plants, and several very important food crops originated in the rainforest, including bananas, mangos, chocolate, coffee, and sugar cane.
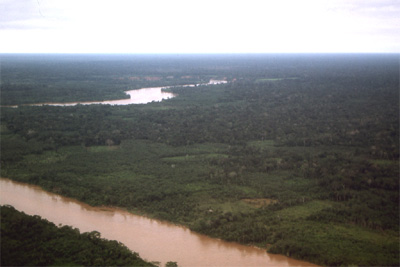
In order to qualify as a tropical rainforest, an area must receive over 250 centimeters of rainfall each year and have an average temperature above 24 degrees centigrade, as well as never experience frosts. The Amazon rainforest in South America is the largest in the world. The second largest is the Congo in central Africa, and other important rainforests can be found in Central America, the Caribbean, and Southeast Asia. Brazil contains about 40% of the world’s remaining tropical rainforest. Its rainforest covers an area of land about 2/3 the size of the continental United States.
There are countless reasons, both anthropocentric and ecocentric, to value rainforests. But they are one of the most threatened types of ecosystems in the world today. It’s somewhat difficult to estimate how quickly rainforests are being cut down, but estimates range from between 50,000 and 170,000 square kilometers per year. Even the most conservative estimates project that if we keep cutting down rainforests as we are today, within about 100 years there will be none left.
How does a rainforest work?
Rainforests are incredibly complex ecosystems, but understanding a few basics about their ecology will help us understand why clear-cutting and fragmentation are such destructive activities for rainforest biodiversity.
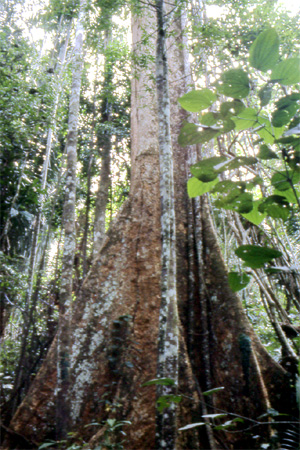
High biodiversity in tropical rainforests means that the interrelationships between organisms are very complex. A single tree may house more than 40 different ant species, each of which has a different ecological function and may alter the habitat in distinct and important ways. Ecologists debate about whether systems that have high biodiversity are stable and resilient, like a spider web composed of many strong individual strands, or fragile, like a house of cards. Both metaphors are likely appropriate in some cases. One thing we can be certain of is that it is very difficult in a rainforest system, as in most other ecosystems, to affect just one type of organism. Also, clear cutting one small area may damage hundreds or thousands of established species interactions that reach beyond the cleared area.
Pollination is a challenge for rainforest trees because there are so many different species, unlike forests in the temperate regions that are often dominated by less than a dozen tree species. One solution is for individual trees to grow close together, making pollination simpler, but this can make that species vulnerable to extinction if the one area where it lives is clear cut. Another strategy is to develop a mutualistic relationship with a long-distance pollinator, like a specific bee or hummingbird species. These pollinators develop mental maps of where each tree of a particular species is located and then travel between them on a sort of “trap-line” that allows trees to pollinate each other. One problem is that if a forest is fragmented then these trap-line connections can be disrupted, and so trees can fail to be pollinated and reproduce even if they haven’t been cut.
The quality of rainforest soils is perhaps the most surprising aspect of their ecology. We might expect a lush rainforest to grow from incredibly rich, fertile soils, but actually, the opposite is true. While some rainforest soils that are derived from volcanic ash or from river deposits can be quite fertile, generally rainforest soils are very poor in nutrients and organic matter. Rainforests hold most of their nutrients in their live vegetation, not in the soil. Their soils do not maintain nutrients very well either, which means that existing nutrients quickly “leech” out, being carried away by water as it percolates through the soil. Also, soils in rainforests tend to be acidic, which means that it’s difficult for plants to access even the few existing nutrients. The section on slash and burn agriculture in the previous module describes some of the challenges that farmers face when they attempt to grow crops on tropical rainforest soils, but perhaps the most important lesson is that once a rainforest is cut down and cleared away, very little fertility is left to help a forest regrow.
What is driving deforestation in the Amazon?
Many factors contribute to tropical deforestation, but consider this typical set of circumstances and processes that result in rapid and unsustainable rates of deforestation. This story fits well with the historical experience of Brazil and other countries with territory in the Amazon Basin.
Population growth and poverty encourage poor farmers to clear new areas of rainforest, and their efforts are further exacerbated by government policies that permit landless peasants to establish legal title to land that they have cleared.
At the same time, international lending institutions like the World Bank provide money to the national government for large-scale projects like mining, construction of dams, new roads, and other infrastructure that directly reduces the forest or makes it easier for farmers to access new areas to clear.
The activities most often encouraging new road development are timber harvesting and mining. Loggers cut out the best timber for domestic use or export, and in the process knock over many other less valuable trees. Those trees are eventually cleared and used for wood pulp, or burned, and the area is converted into cattle pastures. After a few years, the vegetation is sufficiently degraded to make it not profitable to raise cattle, and the land is sold to poor farmers seeking out a subsistence living.
Regardless of how poor farmers get their land, they often are only able to gain a few years of decent crop yields before the poor quality of the soil overwhelms their efforts, and then they are forced to move on to another plot of land. Small-scale farmers also hunt for meat in the remaining fragmented forest areas, which reduces the biodiversity in those areas as well.
Another important factor not mentioned in the scenario above is the clearing of rainforest for industrial agriculture plantations of bananas, pineapples, and sugar cane. These crops are primarily grown for export, and so an additional driver to consider is consumer demand for these crops in countries like the United States.
These cycles of land use, which are driven by poverty and population growth as well as government policies, have led to the rapid loss of tropical rainforests. What is lost in many cases is not simply biodiversity, but also valuable renewable resources that could sustain many generations of humans to come. Efforts to protect rainforests and other areas of high biodiversity is the topic of the next section.

- Deforestation
- Going Green
- Reforestation
- Privacy Policy

Deforestation: Case Studies
Deforestation is putting our planet at risk, as the following case studies exemplify. It is responsible for at least 10 per cent of global greenhouse gas emissions 1 and wipes out 137 species of plants, animals and insects every day 2 . The deplorable practice degenerates soil, losing half of the world’s topsoil over the past 150 years. 3 Deforestation also leads to drought by reducing the amount of water in the atmosphere. 4
Since the 1950s, deforestation has accelerated significantly, particularly in the tropics. 5 This is primarily due to rapid population growth and a resultant increase in demand for food and resources. 6 Agriculture drives about 80 per cent of deforestation today, as land is cleared for livestock, growing animal feed or other crops. 7 The below deforestation case studies of Brazil’s Amazon rainforest and the Congo Basin provide further insights into modern deforestation.
Deforestation case study: Brazil
Nearly two-thirds of the Amazon rainforest – the largest rainforest in the world – is within Brazil’s national borders. 8 Any examination of deforestation case studies would be incomplete without considering tree felling in Brazil.
History of deforestation in Brazil
Humans first discovered the Amazon rainforest about 13,000 years ago. But, it was the arrival of Europeans in the late 15th century that spurred the conversion of the forest into farmland. Nevertheless, the sheer size of the Amazon meant that the rainforest remained largely intact until the early 20th century. It was in the latter half of the 20th century that things began to change. 9

Industrial activities and large-scale agriculture began to eat away the southern and eastern fringes of the Amazon, from the 1950s onwards. 10 Deforestation in Brazil received a significant boost in 1964 when a military dictatorship took power and declared the jungle a security risk. 11 By the 1970s, the government was running television ads encouraging land conversion, provoking millions to migrate north into the forest. 12 Settlements replaced trees, and infrastructure began to develop. Wealthy tycoons subsequently bought the land for cattle ranches or vast fields of soy. 13
By the turn of the 21st century, more than 75 per cent of deforestation in the Amazon was for cattle ranching. But, environmentalists and Indigenous groups drew international attention to the devastation caused and succeeded in curtailing it by 2004. Between 2004 and the early 2010s, annual forest cover loss in Brazil reduced by about 80 per cent. The decline is attributed to “increased law enforcement, satellite monitoring, pressure from environmentalists, private and public sector initiatives, new protected areas, and macroeconomic trends”. 14
Brazil’s deforestation of the Amazon rainforest since 2010
Unfortunately, however, efforts to curtail deforestation in Brazil’s Amazon have stalled since 2012. 15 Tree felling and land conversion have been trending upwards ever since. The economic incentive for chopping the rainforest down has overcome the environmental benefits of leaving it standing. 16 Political movements and lax government legislation have leveraged this to their advantage. President Jair Bolsonaro won the 2018 election with a promise to open up the Amazon to business. 17 Since his inauguration, the rate of deforestation has leapt by as much as 92 per cent. 18
However, there is still hope for the Amazon rainforest. Bolsonaro’s principal international ally was US President Trump. Now that environmentally-conscious Joe Biden has replaced him in the White House, international pressure regarding deforestation will increase heavily. 19 Biden has made this clear with a promise of USD $20 billion to protect the Amazon. 20
The impact of continued deforestation in Brazil
For its three million plant and animal species and one million Indigenous inhabitants, it is imperative that Amazonian deforestation is massively and immediately reduced. 21 As much as 17 per cent of the Amazon has been lost already. 22 If this proportion increases to over 20 per cent, a tipping point will be reached. 23 This will irreversibly break the water cycle, and at least half of the remaining forest will become savannah. 24
Impact on climate change
Losing the Amazon would also mean losing the fight against climate change. Despite the rampant deforestation in recent years, the remaining Amazon rainforest still absorbs between 5 to 10 per cent of all human CO2 emissions. 25 Cutting trees down increases anthropogenic emissions. When felled, burned or left to rot, trees release sequestered carbon. 26 A combination of reducing greenhouse gas emissions and preserving existing forests is crucial to preventing dangerous levels of global warming. 27
Deforestation case study: The Congo Basin
The Congo Basin is the second-largest rainforest in the world. 28 It has been described as the ‘second lungs’ of the Earth because of how much carbon dioxide it absorbs and how much oxygen it produces. 29 But, just as the world’s first lungs – the Amazon – is being destroyed by humans, the Congo’s rainforest is also suffering heavy casualties. 30
60 per cent of the Congo Basin is located within the Democratic Republic of the Congo (DRC). 31 The DRC is one of the world’s largest and poorest countries, though it has immense economic resources. 32 Natural resources have fuelled an ongoing war that has affected all the neighbouring countries and claimed as many as six million lives. 33 The resultant instability combined with corruption and poor governance have led to an ever-increasing rate of deforestation within the DRC’s borders. 34
Deforestation in the Democratic Republic of the Congo (DRC)
Compared to the Amazon and Southeast Asia, deforestation in the Congo Basin has been low over the past few decades. 35 Nevertheless, great swathes of primary forest have been lost. Between 2000 and 2014, an area of forest larger than Bangladesh was destroyed. 36 From 2015 until 2019, 6.37 million hectares of tree cover was razed. 37 In 2019 alone, 475,000 hectares of primary forest disappeared, placing the DRC second only to Brazil for total deforestation that year. 38 Should the current rate of deforestation continue, all primary forest in the Congo Basin will be gone by the end of the century. 39
Drivers of deforestation in the DRC’s Congo Basin
Over the past 20 years, the biggest drivers of deforestation in the DRC has been small-scale subsistence agriculture. Clearing trees for charcoal and fuelwood, urban expansion and mining have also contributed to deforestation. Industrial logging is the most common cause of forest degradation. It opens up deeper areas of the forest to commercial hunting. There has been at least a 60 per cent drop in the region’s forest elephant populations over the past decade due to hunting and poaching. 40

Between 2000 and 2014, small-scale farming contributed to about 90 per cent of the DRC’s deforestation. This trend has not changed in recent years. The majority of small-scale forest clearing is conducted with simple axes by people with no other livelihood options. The region’s political instability and ongoing conflict are therefore inciting the unsustainable rate of deforestation within the Congo Basin. 41
In future, however, industrial logging and land conversion to large-scale agriculture will pose the greatest threats to the Congo rainforest. 42 There are fears that demand for palm oil, rubber and sugar production will promote a massive increase in deforestation. 43 The DRC’s population is also predicted to grow to almost 200 million people by 2050. 44 This increase will threaten the remaining rainforest further, as they try to earn a living in a country deprived of opportunities. 45
The impact of deforestation in the Congo Basin
80 million people depend upon the Congo Basin for their existence. It provides food, charcoal, firewood, medicinal plants, and materials for building and other purposes. But, this rainforest also indirectly supports people across the whole of sub-Saharan Africa. Like all forests, it is instrumental in regulating rainfall, which can affect precipitation hundreds of miles away. The Congo Basin is a primary source of rainfall for the Sahel region, doubling the amount of rainfall in the air that passes over it. 46
The importance of the Congo Basin’s ability to increase precipitation cannot be understated. Areas such as the Horn of Africa are becoming increasingly dry. Drought in Ethiopia and Somalia has put millions of people on emergency food and water rations in recent years. Destroying the DRC’s rainforest would create the largest humanitarian crisis on Earth. 47
It would also be devastating for biodiversity. The Congo Basin shelters some 10,000 animal species and more than 600 tree species. 48 They play a hugely important role in the forest, which has consequences for the entire planet. For instance, elephants, gorillas, and other large herbivores keep the density of small trees very low through predation. 49 This results in a high density of tall trees in the Congo rainforest. 50 Larger trees store more carbon and therefore help to prevent global warming by removing this greenhouse gas from the atmosphere. 51
Preserve our forests
Preserving the Amazon and Congo Basin rainforests is vital for tackling climate change, as these deforestation case studies demonstrate. We must prioritise protecting and enhancing our existing trees if we are to limit the global temperature increase to 1.5°C, as recommended by the IPCC. 52
- Rainforest Alliance. (2018). What is the Relationship Between Deforestation And Climate Change? [online] Available at: https://www.rainforest-alliance.org/articles/relationship-between-deforestation-climate-change.
- www.worldanimalfoundation.com. (n.d.). Deforestation: Clearing The Path For Wildlife Extinctions. [online] Available at: https://www.worldanimalfoundation.com/advocate/wild-earth/params/post/1278141/deforestation-clearing-the-path-for-wildlife-extinctions#:~:text=Seventy%20percent%20of%20the%20Earth.
- World Wildlife Fund. (2000). Soil Erosion and Degradation | Threats | WWF. [online] Available at: https://www.worldwildlife.org/threats/soil-erosion-and-degradation.
- Butler, R.A. (2001). The impact of deforestation. [online] Mongabay. Available at: https://rainforests.mongabay.com/09-consequences-of-deforestation.html.
- The Classroom | Empowering Students in Their College Journey. (2009). The History of Deforestation. [online] Available at: https://www.theclassroom.com/the-history-of-deforestation-13636286.html.
- Greenpeace USA. (n.d.). Agribusiness & Deforestation. [online] Available at: https://www.greenpeace.org/usa/forests/issues/agribusiness/.
- Yale.edu. (2015). The Amazon Basin Forest | Global Forest Atlas. [online] Available at: https://globalforestatlas.yale.edu/region/amazon.
- Time. (2019). The Amazon Rain Forest Is Nearly Gone. We Went to the Front Lines to See If It Could Be Saved. [online] Available at: https://time.com/amazon-rainforest-disappearing/.
- Butler, R. (2020). Amazon Destruction. [online] Mongabay.com. Available at: https://rainforests.mongabay.com/amazon/amazon_destruction.html.
- the Guardian. (2020). Amazon deforestation surges to 12-year high under Bolsonaro. [online] Available at: https://www.theguardian.com/environment/2020/dec/01/amazon-deforestation-surges-to-12-year-high-under-bolsonaro.
- Earth Innovation Institute. (2020). Joe Biden offers $20 billion to protect Amazon forests. [online] Available at: https://earthinnovation.org/2020/03/joe-biden-offers-20-billion-to-protect-amazon-forests/.
- Brazil’s Amazon: Deforestation “surges to 12-year high.” (2020). BBC News. [online] 30 Nov. Available at: https://www.bbc.co.uk/news/world-latin-america-55130304.
- Carbon Brief. (2020). Guest post: Could climate change and deforestation spark Amazon “dieback”? [online] Available at: https://www.carbonbrief.org/guest-post-could-climate-change-and-deforestation-spark-amazon-dieback.
- Union of Concerned Scientists (2012). Tropical Deforestation and Global Warming | Union of Concerned Scientists. [online] www.ucsusa.org. Available at: https://www.ucsusa.org/resources/tropical-deforestation-and-global-warming#:~:text=When%20trees%20are%20cut%20down.
- Milman, O. (2018). Scientists say halting deforestation “just as urgent” as reducing emissions. [online] the Guardian. Available at: https://www.theguardian.com/environment/2018/oct/04/climate-change-deforestation-global-warming-report.
- Bergen, M. (2019). Congo Basin Deforestation Threatens Food and Water Supplies Throughout Africa. [online] World Resources Institute. Available at: https://www.wri.org/blog/2019/07/congo-basin-deforestation-threatens-food-and-water-supplies-throughout-africa.
- www.esa.int. (n.d.). Earth from Space: “Second lungs of the Earth.” [online] Available at: https://www.esa.int/Applications/Observing_the_Earth/Earth_from_Space_Second_lungs_of_the_Earth [Accessed 26 Feb. 2021].
- Erickson-Davis, M. (2018). Congo Basin rainforest may be gone by 2100, study finds. [online] Mongabay Environmental News. Available at: https://news.mongabay.com/2018/11/congo-basin-rainforest-may-be-gone-by-2100-study-finds/.
- Mongabay Environmental News. (2020). Poor governance fuels “horrible dynamic” of deforestation in DRC. [online] Available at: https://news.mongabay.com/2020/12/poor-governance-fuels-horrible-dynamic-of-deforestation-in-drc/ [Accessed 26 Feb. 2021].
- DR Congo country profile. (2019). BBC News. [online] 10 Jan. Available at: https://www.bbc.co.uk/news/world-africa-13283212.
- Butler, R.A. (2001). Congo Deforestation. [online] Mongabay. Available at: https://rainforests.mongabay.com/congo/deforestation.html.
- Mongabay Environmental News. (2020). Poor governance fuels “horrible dynamic” of deforestation in DRC. [online] Available at: https://news.mongabay.com/2020/12/poor-governance-fuels-horrible-dynamic-of-deforestation-in-drc/.
- Butler, R. (2020). The Congo Rainforest. [online] Mongabay.com. Available at: https://rainforests.mongabay.com/congo/.
- Editor, B.W., Environment (n.d.). Large trees are carbon-storing giants. www.thetimes.co.uk. [online] Available at: https://www.thetimes.co.uk/article/large-trees-are-more-valuable-carbon-stores-than-was-thought-k8hnggzs8#:~:text=The%20world [Accessed 26 Feb. 2021].
- IPCC (2018). Summary for Policymakers — Global Warming of 1.5 oC. [online] Ipcc.ch. Available at: https://www.ipcc.ch/sr15/chapter/spm/.
Related posts:
- Top 10 Facts about Deforestation
- Deforestation Debate
- When a Tree Falls It Makes More Than a Sound
- What is Deforestation?
Related Posts

10 Facts About Deforestation in 2021
The following facts about deforestation in 2021 show that our planet is in a precarious position. We must leave our...

Where Is Deforestation Happening in 2021?
To combat climate change, it is important to know where deforestation is happening in 2021. Deforestation currently accounts for at...


The History of Deforestation: The Past and Origins
In many ways, the history of deforestation is the same as the history of agriculture. When humans began to farm...

The Economic Impact of Deforestation
Deforestation is the permanent removal of forests. Agriculture usually replaces the woodland.Youmatter (2020). Deforestation - What Is It? What Are...

Silviculture and Timber Production
Over the past decade, millions of trees have been dying at a frightening pace across the US and Canada. Some...

How Does Planting Trees Affect Climate Change?
While climate change can be a politically controversial topic, tree planting rarely is. Planting trees and climate change are directly...

10 Causes of Deforestation: The Roots of Forest Degradation

How Much CO2 Does a Tree Absorb?

The Effect of Oil Sands Tailings Ponds on Climate Change
- Privacy & Policy
© 2020 Climate Transform
Privacy Overview
Living World - Amazon Case Study
The Amazon is the largest tropical rainforest on Earth. It sits within the Amazon River basin, covers some 40% of the South American continent and as you can see on the map below includes parts of eight South American countries: Brazil, Bolivia, Peru, Ecuador, Colombia, Venezuela, Guyana, and Suriname. The actual word “Amazon” comes from river.

Amazing Amazon facts; • It is home to 1000 species of bird and 60,000 species of plants • 10 million species of insects live in the Amazon • It is home to 20 million people, who use the wood, cut down trees for farms and for cattle. • It covers 2.1 million square miles of land • The Amazon is home to almost 20% of species on Earth • The UK and Ireland would fit into the Amazon 17 times!
The Amazon caught the public’s attention in the 1980s when a series of shocking news reports said that an area of rainforest the size of Belgium was being cut down and subsequently burnt every year. This deforestation has continued to the present day according to the Sao Paulo Space Research Centre. In 2005 they had lost 17% of Amazon rainforest or 650000 square kilometres. Their satellite data is also showing increased deforestation in parts of the Amazon. The process of deforestation The Amazon helps a Newly Emerging Economy(NEE), Brazil, to make money. They build roads into the forest, logging firms then go in and take out valuable hard woods such as mahogany and cedar, worth thousands of pounds in richer economies like Europe. Then farmers, often cattle ranchers from big companies, burn the rest to make way for cattle pasture. 75% of cleared areas are used in this way. This is clearly shown on the map on figure 22 in red. Many of the deforested areas follow roads and branch off from there. Deforestation is also worse in the South and South East of the Amazon basin, closer to major centres of population in Brazil.

© WWF Source Used with permission.
The causes of deforestation 1. Subsistence and commercial farming – subsistence farming is where poor farmers occupy plots of the forest to grow food to feed themselves and their families. They clear forest and then burn it, hence the name slash and burn. They grow crops until the soil is exhausted and then move on. This contributes to deforestation but not as much as commercial farming (Farming to sell produce for a profit to retailers or food processing companies). The Brazilian region of Mato Grosso was affected by deforestation in the 1980s and 1990s. 43% of rainforest losses were in this region, and area almost ½ the size of France. It has been replaced by fields for grain and cattle. This has allowed Brazil to overtake Australia as the largest exporter of beef in the world. The land is also flat and easy to farm. It also has high temperatures and lots of rainfall.
2. Logging – This involves cutting down trees for sale as timber or pulp. The timber is used to build homes, furniture, etc. and the pulp is used to make paper and paper products. Logging can be either selective or clear cutting. Selective logging is selective because loggers choose only wood that is highly valued, such as mahogany. Clear-cutting is not selective. Loggers are interested in all types of wood and therefore cut all of the trees down, thus clearing the forest, hence the name- clear-cutting.
3. Road building – trees are also clear for roads. Roads are an essential way for the Brazilian government to allow development of the Amazon rainforest. However, unless they are paved many of the roads are unusable during the wettest periods of the year. The Trans Amazonian Highway has already opened up large parts of the forest and now a new road is going to be paved, the BR163 is a road that runs 1700km from Cuiaba to Santarem. The government planned to tarmac it making it a superhighway. This would make the untouched forest along the route more accessible and under threat from development.
4. Mineral extraction – forests are also cleared to make way for huge mines. The Brazilian part of the Amazon has mines that extract iron, manganese, nickel, tin, bauxite, beryllium, copper, lead, tungsten, zinc and gold!
The Belo Monte dam site under construction, copyright Used with the kind permission of Phil Clarke-Hill - His website is amazing, click here to see it.
5. Energy development – This has focussed mainly on using Hydro Electric Power, and there are 150 new dams planned for the Amazon alone. The dams create electricity as water is passed through huge pipes within them, where it turns a turbine which helps to generate the electricity. The power in the Amazon is often used for mining. Dams displace many people and the reservoirs they create flood large area of land, which would previously have been forest. They also alter the hydrological cycle and trap huge quantities of sediment behind them. The huge Belo Monte dam started operating in April 2016 and will generate over 11,000 Mw of power. A new scheme the 8,000-megawatt São Luiz do Tapajós dam has been held up because of the concerns over the impacts on the local Munduruku people.

6. Settlement & population growth – populations are growing within the Amazon forest and along with them settlements. Many people are migrating to the forest looking for work associated with the natural wealth of this environment. Settlements like Parauapebas, an iron ore mining town, have grown rapidly, destroying forest and replacing it with a swath of shanty towns. The population has grown from 154,000 in 2010 to 220,000 in 2012. The Brazilian Amazon’s population grew by a massive 23% between 2000 and 2010, 11% above the national average.
Impacts of deforestation – economic development, soil erosion, contribution to climate change. • Every time forest is cleared species are lost – so we lose BIODIVERSITY • Climate Change - Burning the forest releases greenhouse gasses like CO2. This contributes to the warming of our planet via climate change and global warming. In addition, the loss of trees prevents CO2 being absorbed, making the problem worse. The Amazon also helps to drive the global atmospheric system. There is a lot of rainfall there and changes to the Amazon could disrupt the global system. • Economic development – Brazil has used the forests as a way to develop their country. The forest has many natural riches that can be exploited. In addition, Brazil has huge foreign debt and lots of poor people to feed, so they want to develop the forest. May Brazilians see deforestation as a way to help develop their country and improve people’s standard of living. • Soil erosion - the soils of the Amazon forest are not fertile and are quickly exhausted once the forest is cleared. The farmers now artificially fertilise the soil when in the past the nutrient cycle would have done this naturally. In addition, the lack of forest cover means that soils are exposed to the rainfall. This washes huge amounts of soil into rivers in the process of soil erosion.
NEXT TOPIC - Living World - Sustainable Forest Management
©2015 Cool Geography
- Copyright Policy
- Privacy & Cookies
- Testimonials
- Feedback & support

Case Study: Deforestation in the Amazon Rainforest
Deforestation in the amazon rainforest.
The Amazon rainforest area spans about 8,200,000km 2 across 9 countries, making it the largest rainforest in the world. The tree coverage in 1970 was 4.1m km 2 . In 2018, it was 3.3m km 2 . Between 2001 and 2013, the causes of Amazonian deforestation were:
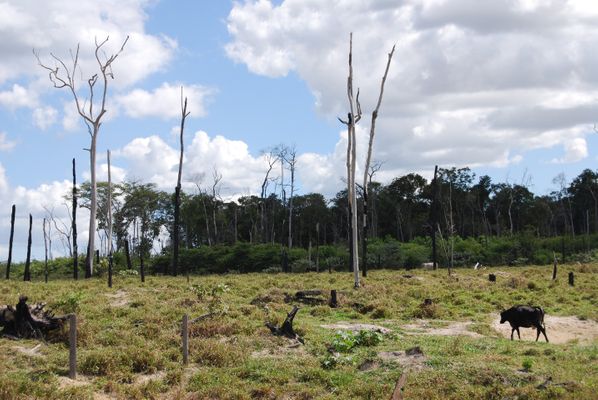
Pasture and cattle ranching = 63%
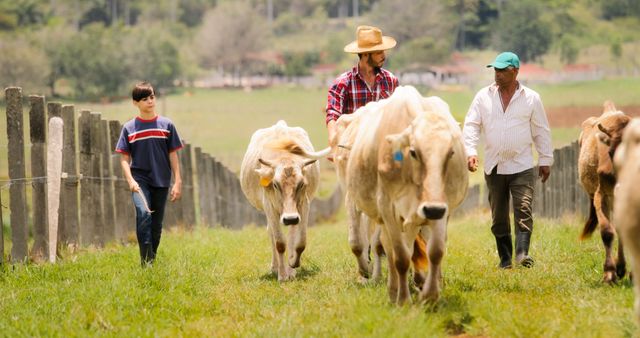
Small-scale, subsistence farmers = 12%
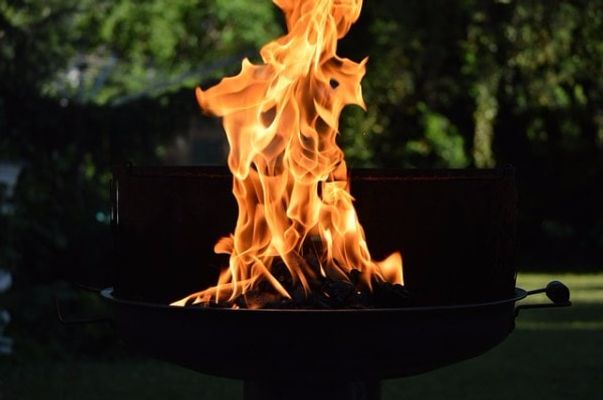
Commercial crop farming = 7%
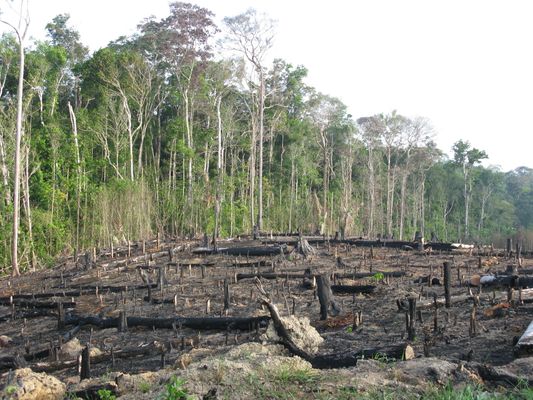
Tree felling and logging = 6%
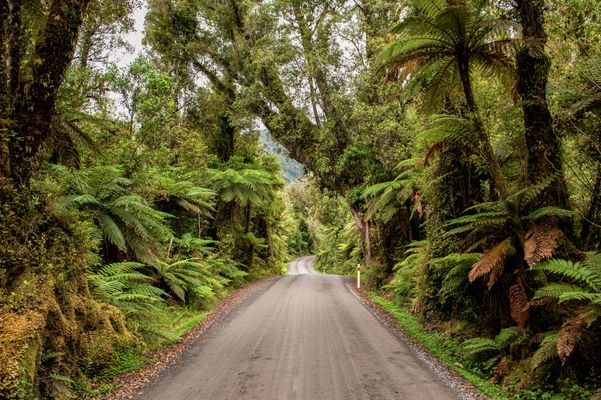
Other activities = 3%
- E.g. plantations, mining, road-building, and construction.
Impacts of Deforestation in the Amazon
Deforestation in the Amazon rainforest has the following environmental and economic impacts:
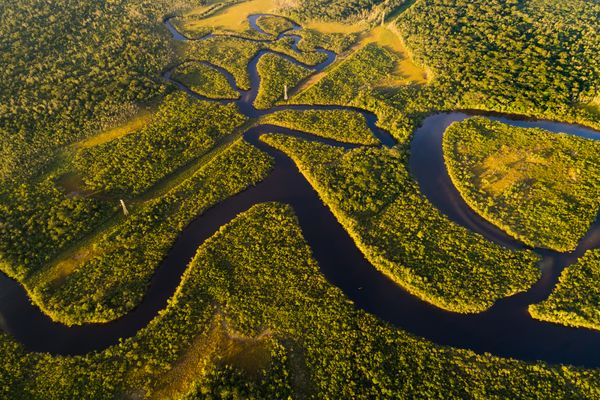
Environmental impact of Amazonian deforestation
- Photosynthesis by trees in the Amazon absorbs 5% of the world's carbon emissions each year (2bn tons of CO2).
- 100 billion tonnes of carbon are stored in the wood of the trees in the Amazon.
- If the Amazon were completely deforested, it would release the 100bn tonnes and also reduce the amount of carbon dioxide taken out of the atmosphere by 2bn tons each year.
- Trees anchor soil in the ground, bound to their roots. Deforestation damages the topsoil and once this has happened, the fertility of the ground is seriously damaged.
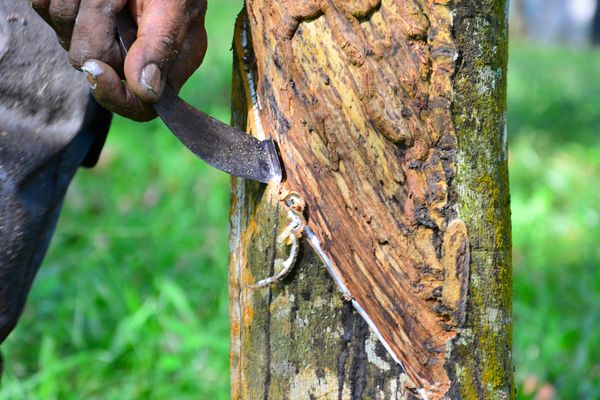
Economic impact of Amazonian deforestation
- Deforestation has fuelled the economic development of poor countries.
- In 2018, Brazil exported $28bn worth of metals. The mining industry creates jobs, exports and helps increase Brazilian people's standard of living.
- Similarly, hydroelectric power plants and cattle farms help to create jobs.
- In 2018, Brazil became the world's largest exporter of beef.
- Rio Tinto, an iron ore mining company employs 47,000 people globally and thousands of these are in Brazil.
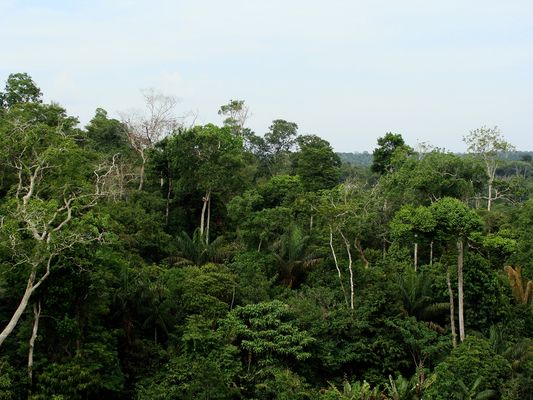
The rate of deforestation in the Amazon
- In 2015, the Brazilian President Dilma Rousseff claimed that the rate of deforestation had fallen by 83% and that actually Brazil was going to reforest the Amazon.
- However, the policies under President Temer and President Bolsonaro has reversed Rousseff's plan. In 2019, under Bolsonaro, the rate of deforestation was increasing again.
1 The Challenge of Natural Hazards
1.1 Natural Hazards
1.1.1 Natural Hazards
1.1.2 Types of Natural Hazards
1.1.3 Factors Affecting Risk
1.1.4 People Affecting Risk
1.1.5 Ability to Cope With Natural Hazards
1.1.6 How Serious Are Natural Hazards?
1.1.7 End of Topic Test - Natural Hazards
1.1.8 Exam-Style Questions - Natural Hazards
1.2 Tectonic Hazards
1.2.1 The Earth's Layers
1.2.2 Tectonic Plates
1.2.3 The Earth's Tectonic Plates
1.2.4 Convection Currents
1.2.5 Plate Margins
1.2.6 Volcanoes
1.2.7 Volcano Eruptions
1.2.8 Effects of Volcanoes
1.2.9 Primary Effects of Volcanoes
1.2.10 Secondary Effects of Volcanoes
1.2.11 Responses to Volcanic Eruptions
1.2.12 Immediate Responses to Volcanoes
1.2.13 Long-Term Responses to Volcanoes
1.2.14 Earthquakes
1.2.15 Earthquakes at Different Plate Margins
1.2.16 What is an Earthquake?
1.2.17 Measuring Earthquakes
1.2.18 Immediate Responses to Earthquakes
1.2.19 Long-Term Responses to Earthquakes
1.2.20 Case Studies: The L'Aquila Earthquake
1.2.21 Case Studies: The Kashmir Earthquake
1.2.22 Earthquake Case Study: Chile 2010
1.2.23 Earthquake Case Study: Nepal 2015
1.2.24 Reducing the Impact of Tectonic Hazards
1.2.25 Protecting & Planning
1.2.26 Living with Tectonic Hazards 2
1.2.27 End of Topic Test - Tectonic Hazards
1.2.28 Exam-Style Questions - Tectonic Hazards
1.2.29 Tectonic Hazards - Statistical Skills
1.3 Weather Hazards
1.3.1 Winds & Pressure
1.3.2 The Global Atmospheric Circulation Model
1.3.3 Surface Winds
1.3.4 UK Weather Hazards
1.3.5 Changing Weather in the UK
1.3.6 Tropical Storms
1.3.7 Tropical Storm Causes
1.3.8 Features of Tropical Storms
1.3.9 The Structure of Tropical Storms
1.3.10 The Effect of Climate Change on Tropical Storms
1.3.11 The Effects of Tropical Storms
1.3.12 Responses to Tropical Storms
1.3.13 Reducing the Effects of Tropical Storms
1.3.14 Tropical Storms Case Study: Katrina
1.3.15 Tropical Storms Case Study: Haiyan
1.3.16 UK Weather Hazards Case Study: Somerset 2014
1.3.17 End of Topic Test - Weather Hazards
1.3.18 Exam-Style Questions - Weather Hazards
1.3.19 Weather Hazards - Statistical Skills
1.4 Climate Change
1.4.1 Climate Change
1.4.2 Evidence for Climate Change
1.4.3 Natural Causes of Climate Change
1.4.4 Human Causes of Climate Change
1.4.5 Effects of Climate Change on the Environment
1.4.6 Effects of Climate Change on People
1.4.7 Climate Change Mitigation Strategies
1.4.8 Adaptation to Climate Change
1.4.9 End of Topic Test - Climate Change
1.4.10 Exam-Style Questions - Climate Change
1.4.11 Climate Change - Statistical Skills
2 The Living World
2.1 Ecosystems
2.1.1 Ecosystems
2.1.2 Food Chains & Webs
2.1.3 Ecosystem Cascades
2.1.4 Global Ecosystems
2.1.5 Ecosystem Case Study: Freshwater Ponds
2.2 Tropical Rainforests
2.2.1 Tropical Rainforests
2.2.2 Interdependence of Tropical Rainforests
2.2.3 Adaptations of Plants to Rainforests
2.2.4 Adaptations of Animals to Rainforests
2.2.5 Biodiversity of Tropical Rainforests
2.2.6 Deforestation
2.2.7 Impacts of Deforestation
2.2.8 Case Study: Deforestation in the Amazon Rainforest
2.2.9 Why Protect Rainforests?
2.2.10 Sustainable Management of Rainforests
2.2.11 Case Study: Malaysian Rainforest
2.2.12 End of Topic Test - Tropical Rainforests
2.2.13 Exam-Style Questions - Tropical Rainforests
2.2.14 Deforestation - Statistical Skills
2.3 Hot Deserts
2.3.1 Hot Deserts
2.3.2 Interdependence in Hot Deserts
2.3.3 Adaptation of Plants to Hot Deserts
2.3.4 Adaptation of Animals to Hot Deserts
2.3.5 Biodiversity in Hot Deserts
2.3.6 Case Study: Sahara Desert
2.3.7 Desertification
2.3.8 Reducing the Risk of Desertification
2.3.9 Case Study: Thar Desert
2.3.10 End of Topic Test - Hot Deserts
2.3.11 Exam-Style Questions - Hot Deserts
2.4 Tundra & Polar Environments
2.4.1 Overview of Cold Environments
2.4.2 Interdependence of Cold Environments
2.4.3 Adaptations of Plants to Cold Environments
2.4.4 Adaptations of Animals to Cold Environments
2.4.5 Biodiversity in Cold Environments
2.4.6 Case Study: Alaska
2.4.7 Sustainable Management
2.4.8 Case Study: Svalbard
2.4.9 End of Topic Test - Tundra & Polar Environments
2.4.10 Exam-Style Questions - Cold Environments
3 Physical Landscapes in the UK
3.1 The UK Physical Landscape
3.1.1 The UK Physical Landscape
3.1.2 Examples of the UK's Landscape
3.2 Coastal Landscapes in the UK
3.2.1 Types of Wave
3.2.2 Weathering
3.2.3 Mass Movement
3.2.4 Processes of Erosion
3.2.5 Wave-Cut Platforms
3.2.6 Headlands & Bays
3.2.7 Caves, Arches & Stacks
3.2.8 Longshore Drift
3.2.9 Sediment Transport
3.2.10 Deposition
3.2.11 Spits, Bars & Sand Dunes
3.2.12 Coastal Management - Hard Engineering
3.2.13 Coastal Management - Soft Engineering
3.2.14 Case Study: Landforms on the Dorset Coast
3.2.15 Coastal Management - Managed Retreat
3.2.16 Coastal Management Case Study - Holderness
3.2.17 Coastal Management Case Study: Swanage
3.2.18 Coastal Management Case Study - Lyme Regis
3.2.19 End of Topic Test - Coastal Landscapes in the UK
3.2.20 Exam-Style Questions - Coasts
3.3 River Landscapes in the UK
3.3.1 The Long Profile of a River
3.3.2 The Cross Profile of a River
3.3.3 Vertical & Lateral Erosion
3.3.4 River Valley Case Study - River Tees
3.3.5 Processes of Erosion
3.3.6 Sediment Transport
3.3.7 River Deposition
3.3.8 Waterfalls & Gorges
3.3.9 Interlocking Spurs
3.3.10 Meanders
3.3.11 Oxbow Lakes
3.3.12 Floodplains
3.3.13 Levees
3.3.14 Estuaries
3.3.15 Case Study: The River Clyde
3.3.16 River Management
3.3.17 Hydrographs
3.3.18 Flood Defences - Hard Engineering
3.3.19 Flood Defences - Soft Engineering
3.3.20 River Management Case Study - Boscastle
3.3.21 River Management Case Study - Banbury
3.3.22 End of Topic Test - River Landscapes in the UK
3.3.23 Exam-Style Questions - Rivers
3.4 Glacial Landscapes in the UK
3.4.1 The UK in the Last Ice Age
3.4.2 Glacial Processes
3.4.3 Glacial Landforms Caused by Erosion
3.4.4 Tarns, Corries, Glacial Troughs & Truncated Spurs
3.4.5 Types of Moraine
3.4.6 Drumlins & Erratics
3.4.7 Snowdonia
3.4.8 Land Use in Glaciated Areas
3.4.9 Conflicts in Glacial Landscapes
3.4.10 Tourism in Glacial Landscapes
3.4.11 Coping with Tourism Impacts in Glacial Landscapes
3.4.12 Case Study - Lake District
3.4.13 End of Topic Test - Glacial Landscapes in the UK
3.4.14 Exam-Style Questions - Glacial Landscapes
4 Urban Issues & Challenges
4.1 Urban Issues & Challenges
4.1.1 Urbanisation
4.1.2 Factors Causing Urbanisation
4.1.3 Megacities
4.1.4 Urbanisation Case Study: Lagos
4.1.5 Urbanisation Case Study: Rio de Janeiro
4.1.6 UK Cities
4.1.7 Case Study: Urban Regen Projects - Manchester
4.1.8 Case Study: Urban Change in Liverpool
4.1.9 Case Study: Urban Change in Bristol
4.1.10 Sustainable Urban Life
4.1.11 Reducing Traffic Congestion
4.1.12 End of Topic Test - Urban Issues & Challenges
4.1.13 Exam-Style Questions - Urban Issues & Challenges
4.1.14 Urban Issues -Statistical Skills
5 The Changing Economic World
5.1 The Changing Economic World
5.1.1 Measuring Development
5.1.2 Limitations of Developing Measures
5.1.3 Classifying Countries Based on Wealth
5.1.4 The Demographic Transition Model
5.1.5 Stages of the Demographic Transition Model
5.1.6 Physical Causes of Uneven Development
5.1.7 Historical Causes of Uneven Development
5.1.8 Economic Causes of Uneven Development
5.1.9 Consequences of Uneven Development
5.1.10 How Can We Reduce the Global Development Gap?
5.1.11 Case Study: Tourism in Kenya
5.1.12 Case Study: Tourism in Jamaica
5.1.13 Case Study: Economic Development in India
5.1.14 Case Study: Aid & Development in India
5.1.15 Case Study: Economic Development in Nigeria
5.1.16 Case Study: Aid & Development in Nigeria
5.1.17 End of Topic Test - The Changing Economic World
5.1.18 Exam-Style Questions - The Changing Economic World
5.1.19 Changing Economic World - Statistical Skills
5.2 Economic Development in the UK
5.2.1 Causes of Economic Change in the UK
5.2.2 The UK's Post-Industrial Economy
5.2.3 The Impacts of UK Industry on the Environment
5.2.4 Change in the UK's Rural Areas
5.2.5 Transport in the UK
5.2.6 The North-South Divide
5.2.7 Regional Differences in the UK
5.2.8 The UK's Links to the World
6 The Challenge of Resource Management
6.1 Resource Management
6.1.1 Global Distribution of Resources
6.1.2 Uneven Distribution of Resources
6.1.3 Food in the UK
6.1.4 Agribusiness
6.1.5 Demand for Water in the UK
6.1.6 Water Pollution in the UK
6.1.7 Matching Supply & Demand of Water in the UK
6.1.8 The UK's Energy Mix
6.1.9 Issues with Sources of Energy
6.1.10 Resource Management - Statistical Skills
6.2.1 Areas of Food Surplus & Food Deficit
6.2.2 Increasing Food Consumption
6.2.3 Food Supply & Food Insecurity
6.2.4 Impacts of Food Insecurity
6.2.5 Increasing Food Supply
6.2.6 Case Study: Thanet Earth
6.2.7 Creating a Sustainable Food Supply
6.2.8 Case Study: Agroforestry in Mali
6.2.9 End of Topic Test - Food
6.2.10 Exam-Style Questions - Food
6.2.11 Food - Statistical Skills
6.3.1 Water Surplus & Water Deficit
6.3.2 Increasing Water Consumption
6.3.3 What Affects the Availability of Water?
6.3.4 Impacts of Water Insecurity
6.3.5 Increasing Water Supplies
6.3.6 Case Study: Water Transfer in China
6.3.7 Sustainable Water Supply
6.3.8 Case Study: Kenya's Sand Dams
6.3.9 Case Study: Lesotho Highland Water Project
6.3.10 Case Study: Wakel River Basin Project
6.3.11 Exam-Style Questions - Water
6.3.12 Water - Statistical Skills
6.4.1 Global Demand for Energy
6.4.2 Increasing Energy Consumption
6.4.3 Factors Affecting Energy Supply
6.4.4 Impacts of Energy Insecurity
6.4.5 Increasing Energy Supply - Solar
6.4.6 Increasing Energy Supply - Water
6.4.7 Increasing Energy Supply - Wind
6.4.8 Increasing Energy Supply - Nuclear
6.4.9 Increasing Energy Supply - Fossil Fuels
6.4.10 Carbon Footprints
6.4.11 Energy Conservation
6.4.12 Case Study: Rice Husks in Bihar
6.4.13 Exam-Style Questions - Energy
6.4.14 Energy - Statistical Skills
Jump to other topics
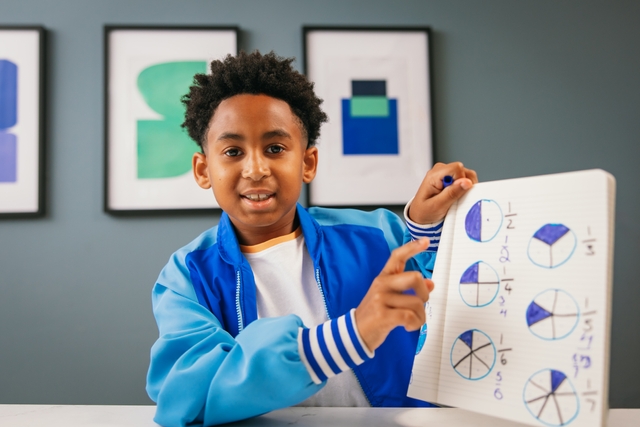
Unlock your full potential with GoStudent tutoring
Affordable 1:1 tutoring from the comfort of your home
Tutors are matched to your specific learning needs
30+ school subjects covered
Impacts of Deforestation
Why Protect Rainforests?

IMAGES
VIDEO
COMMENTS
The study examines the impact of deforestation on the health of the Amazon Rainforest in Rondônia by analysing land use changes from 1975 to 2024 using GIS software (LandSat Explorer, ArcGIS Online, ImageJ) and satellite images.
Many factors contribute to tropical deforestation, but consider this typical set of circumstances and processes that result in rapid and unsustainable rates of deforestation. This story fits well with the historical experience of Brazil and other countries with territory in the Amazon Basin.
Two deforestation case studies provide insights into the damage the practice is doing to our rainforests and to the climate.
GIS analysis of select strictly protected areas supported by the Amazon Region Protected Areas Program (ARPA)
Monitoring the Deforestation of Amazon’s Tropical Rainforest using Remote Sensing and Geographic Information Systems Tools: Case study, Mato Grosso, Brazil. One of the most serious...
Deforestation in the Amazon has numerous effects on the ecosystems surrounding the rainforest. Deforestation causes erosion, loss of nutrients, loss of biodiversity, and sediment build-up in the Amazon River.
Recent estimates of deforestation suggest that between 1 and 3 million hectares are being cleared annually in the Amazon Basin (Laurence 1997). Based on estimates of 1% annual tropical forest loss, the Amazon may be losing as many as 11 to 16 species per day (Wilson 1989), and the resulting ecosystems are often highly degraded (Buschbacher 1986).
Deforestation is a huge threat to the delicate tropical rainforest ecosystem. Subsistence farming is where farmers only produce enough food for themselves and their families to eat, rather than growing surplus food for profit. (areas are cut down and then burnt to remove the vegetation).
The Brazilian region of Mato Grosso was affected by deforestation in the 1980s and 1990s. 43% of rainforest losses were in this region, and area almost ½ the size of France. It has been replaced by fields for grain and cattle.
Deforestation in the Amazon rainforest has the following environmental and economic impacts: Photosynthesis by trees in the Amazon absorbs 5% of the world's carbon emissions each year (2bn tons of CO2). 100 billion tonnes of carbon are stored in the wood of the trees in the Amazon.