
- Table of Contents
- New in this Archive
- Chronological
- Editorial Information
- About the SEP
- Editorial Board
- How to Cite the SEP
- Special Characters
- Support the SEP
- PDFs for SEP Friends
- Make a Donation
- SEPIA for Libraries
- Entry Contents

Bibliography
Academic tools.
- Friends PDF Preview
- Author and Citation Info
- Back to Top
The Experience and Perception of Time
We see colours, hear sounds and feel textures. Some aspects of the world, it seems, are perceived through a particular sense. Others, like shape, are perceived through more than one sense. But what sense or senses do we use when perceiving time? It is certainly not associated with one particular sense. In fact, it seems odd to say that we see, hear or touch time passing. And indeed, even if all our senses were prevented from functioning for a while, we could still notice the passing of time through the changing pattern of our thought. Perhaps, then, we have a special faculty, distinct from the five senses, for detecting time. Or perhaps, as seems more likely, we notice time through perception of other things. But how?
Time perception raises a number of intriguing puzzles, including what it means to say we perceive time. In this article, we shall explore the various processes through which we are made aware of time, and which influence the way we think time really is. Inevitably, we shall be concerned with the psychology of time perception, but the purpose of the article is to draw out the philosophical issues, and in particular whether and how aspects of our experience can be accommodated within certain metaphysical theories concerning the nature of time and causation.
1. What is ‘the perception of time’?
2. kinds of temporal experience, 3. duration, 4. the specious present, 5. past, present and the passage of time, 6. time order, 7. the metaphysics of time perception, other internet resources, related entries.
The very expression ‘the perception of time’ invites objection. Insofar as time is something different from events, we do not perceive time as such, but changes or events in time. But, arguably, we do not perceive events only, but also their temporal relations. So, just as it is natural to say that we perceive spatial distances and other relations between objects (I see the dragonfly as hovering above the surface of the water), it seems natural to talk of perceiving one event following another (the thunderclap as following the flash of lightning), though even here there is a difficulty. For what we perceive, we perceive as present —as going on right now. Can we perceive a relation between two events without also perceiving the events themselves? If not, then it seems we perceive both events as present, in which case we must perceive them as simultaneous, and so not as successive after all. There is then a paradox in the notion of perceiving an event as occurring after another, though one that perhaps admits of a straightforward solution. When we perceive B as coming after A, we have, surely, ceased to perceive A. In which case, A is merely an item in our memory. Now if we wanted to construe ‘perceive’ narrowly, excluding any element of memory, then we would have to say that we do not, after all, perceive B as following A. But in this article, we shall construe ‘perceive’ more broadly, to include a wide range of experiences of time that essentially involve the senses. In this wide sense, we perceive a variety of temporal aspects of the world. We shall begin by enumerating these, and then consider accounts of how such perception is possible.
There are a number of what Ernst Pöppel (1978) calls ‘elementary time experiences’, or fundamental aspects of our experience of time. Among these we may list the experience of (i) duration; (ii) non-simultaneity; (iii) order; (iv) past and present; (v) change, including the passage of time. It might be thought that experience of non-simultaneity is the same as experience of time order, but it appears that, when two events occur very close together in time, we can be aware that they occur at different times without being able to say which one came first (see Hirsh and Sherrick 1961). We might also think that perception of order was itself explicable in terms of our experience of the distinction between past and present. There will certainly be links here, but it is a contentious question whether the experience of tense —that is, experiencing an event as past or present—is more fundamental than the experience of order, or vice versa, or whether indeed there is such a thing as the experience of tense at all. This issue is taken up below. Finally, we should expect to see links between the perception of time order and the perception of motion if the latter simply involves perception of the order of the different spatial positions of an object. This is another contentious issue that is taken up below.
One of the earliest, and most famous, discussions of the nature and experience of time occurs in the autobiographical Confessions of St Augustine. Augustine was born in Numidia (now Algeria) in 354 AD, held chairs in rhetoric at Carthage and Milan, and become Bishop of Hippo in 395. He died in 430. As a young adult, he had rejected Christianity, but was finally converted at the age of 32. Book XI of the Confessions contains a long and fascinating exploration of time, and its relation to God. During the course of it Augustine raises the following conundrum: when we say that an event or interval of time is short or long, what is it that is being described as of short or long duration? It cannot be what is past, since that has ceased to be, and what is non-existent cannot presently have any properties, such as being long. But neither can it be what is present, for the present has no duration. (For the reason why the present must be regarded as durationless, see the section on the specious present, below.) In any case, while an event is still going on, its duration cannot be assessed.
Augustine’s answer to this riddle is that what we are measuring, when we measure the duration of an event or interval of time, is in the memory. From this he derives the radical conclusion that past and future exist only in the mind. While not following Augustine all the way to the mind-dependence of other times, we can concede that the perception of temporal duration is crucially bound up with memory. It is some feature of our memory of the event (and perhaps specifically our memory of the beginning and end of the event) that allows us to form a belief about its duration. This process need not be described, as Augustine describes it, as a matter of measuring something wholly in the mind. Arguably, at least, we are measuring the event or interval itself, a mind-independent item, but doing so by means of some psychological process.
Whatever the process in question is, it seems likely that it is intimately connected with what William Friedman (1990) calls ‘time memory’: that is, memory of when some particular event occurred. That there is a close connection here is entailed by the plausible suggestion that we infer (albeit subconsciously) the duration of an event, once it has ceased, from information about how long ago the beginning of that event occurred. That is, information that is metrical in nature (e.g. ‘the burst of sound was very brief’) is derived from tensed information, concerning how far in the past something occurred. The question is how we acquire this tensed information. It may be direct or indirect, a contrast we can illustrate by two models of time memory described by Friedman. He calls the first the strength model of time memory. If there is such a thing as a memory trace that persists over time, then we could judge the age of a memory (and therefore how long ago the event remembered occurred) from the strength of the trace. The longer ago the event, the weaker the trace. This provides a simple and direct means of assessing the duration of an event. Unfortunately, the trace model comes into conflict with a very familiar feature of our experience: that some memories of recent events may fade more quickly than memories of more distant events, especially when those distant events were very salient ones (visiting a rarely seen and frightening relative when one was a child, for instance.) A contrasting account of time memory is the inference model . According to this, the time of an event is not simply read off from some aspect of the memory of it, but is inferred from information about relations between the event in question and other events whose date or time is known.
The inference model may be plausible enough when we are dealing with distant events, but rather less so for much more recent ones. In addition, the model posits a rather complex cognitive operation that is unlikely to occur in non-human animals, such as the rat. Rats, however, are rather good at measuring time over short intervals of up to a minute, as demonstrated by instrumental conditioning experiments involving the ‘free operant procedure’. In this, a given response (such as depressing a lever) will delay the occurrence of an electric shock by a fixed period of time, such as 40 seconds, described as the R-S (response-shock) interval. Eventually, rate of responding tracks the R-S interval, so that the probability of responding increases rapidly as the end of the interval approaches. (See Mackintosh 1983 for a discussion of this and related experiments.) It is hard to avoid the inference here that the mere passage of time itself is acting as a conditioned stimulus: that the rats, to put it in more anthropocentric terms, are successfully estimating intervals of time. In this case, the strength model seems more appropriate than the inference model.
The term ‘specious present’ was first introduced by the psychologist E.R. Clay, but the best known characterisation of it was due to William James, widely regarded as one of the founders of modern psychology. He lived from 1842 to 1910, and was professor both of psychology and of philosophy at Harvard. His definition of the specious present goes as follows: ‘the prototype of all conceived times is the specious present, the short duration of which we are immediately and incessantly sensible’ (James 1890). How long is this specious present? Elsewhere in the same work, James asserts ‘We are constantly aware of a certain duration—the specious present—varying from a few seconds to probably not more than a minute, and this duration (with its content perceived as having one part earlier and another part later) is the original intuition of time.’ This surprising variation in the length of the specious present makes one suspect that more than one definition is hidden in James’ rather vague characterisation.
There are two sources of ambiguity here. One is over whether ‘the specious present’ refers to the object of the experience, namely a duration in time, or the way in which that object is presented to us. The second is over how we should interpret ‘immediately sensible’. James’ words suggest that the specious present is the duration itself, picked out as the object of a certain kind of experience. But ‘ immediately sensible’admits of a number of disambiguations. So we could define the specious present as:
- the span of short-term memory;
- the duration which is perceived, not as duration, but as instantaneous;
- the duration which is directly perceived — i.e. not through the intermediary of a number of other, perhaps instantaneous, perceptions;
- the duration which is perceived both as present and as extended in time.
If James means the first of these, that would certainly explain his suggestion that it could last up to a minute. But this does not seem to have much to do specifically with the experience of presentness , since we can certainly hold something in the short-term memory and yet recognise it as past. James may be thinking of cases where we are listening to a sentence: if we did not somehow hold all the words in our conscious mind, we would not understand the sentence as a whole. But it is clear that the words are not experienced as simultaneous, for then the result would be an unintelligible jumble of sounds. (2) is illustrated by the familiar fact that some movements are so fast that we see them as a blur, such as when we look at a fan. What is in fact taking place at different times is presented as happening in an instant. But this is not standardly what is meant by the specious present. (3) is a construal that is found in the literature (see, e.g., Kelly 2005), but it is not obvious that that is what James had in mind, since James is concerned with the phenomenology of time perception, and whether or not an experience constitutes a direct or indirect perception of an interval does not seem to be a phenomenological matter. (Besides which, as Kelly points out, we might think it odd to suppose that past parts of the interval could be directly perceived.)
That leaves us with (4): a duration which is perceived both as present and as temporally extended. This present of experience is ‘specious’ in that, unlike the objective present (if there is such a thing — see The metaphysics of time perception below) it is an interval and not a durationless instant. The real or objective present must be durationless for, as Augustine argued, in an interval of any duration, there are earlier and later parts. So if any part of that interval is present, there will be another part that is past or future.
But is it possible to perceive something as extended and as present? If we hear a short phrase of music, we seem to hear the phrase as present, and yet — because it is a phrase rather than a single chord — we also hear the notes as successive, and therefore as extending over an interval. If this does not seem entirely convincing, consider the perception of motion. As Broad (1923) puts it, ‘to see a second-hand moving is quite a different thing from "seeing" that a hour-hand has moved.’ It is not that we see the current position of the second hand and remember where it was a second ago: we just see the motion. That leads to the following argument:
Still, there is more than an air of paradox about this. If successive parts of the motion (or musical phrase, or whatever change we perceive) are perceived as present, then surely they are perceived as simultaneous. But if they are perceived as simultaneous, then the motion will simply be a blur, as it is in cases where it is too fast to perceive as motion. The fact that we do not see it as motion suggests that we do not see the successive parts of it as simultaneous, and so do not see them as present. But then how do we explain the distinction to which Broad directs our attention?
One way out of this impasse is to suggest that two quite distinct processes are going on in the perception of motion (and other kinds of change). One is the perception of successive states as successive, for example the different positions of the second hand. The other is the perception of pure movement. This second perception, which may involve a more primitive system than the first, does not contain as part the recognition of earlier and later elements. (Le Poidevin 2007, Chapter 5.) Alternatively, we might attempt to explain the phenomena of temporal experience without appeal to the notion of the specious present at all (see Arstila, 2018).
The previous section indicated the importance of distinguishing between perceiving the present and perceiving something as present. We may perceive as present items that are past. Indeed, given the finite speed of the transmission of both light and sound (and the finite speed of transmission of information from receptors to brain), it seems that we only ever perceive what is past. However, this does not by itself tell us what it is to perceive something as present, rather than as past. Nor does it explain the most striking feature of our experience as-of the present: that it is constantly changing. The passage (or apparent passage) of time is its most striking feature, and any account of our perception of time must account for this aspect of our experience.
Here is one attempt to do so. The first problem is to explain why our temporal experience is limited in a way in which our spatial experience is not. We can perceive objects that stand in a variety of spatial relations to us: near, far, to the left or right, up or down, etc. Our experience is not limited to the immediate vicinity (although of course our experience is spatially limited to the extent that sufficiently distant objects are invisible to us). But, although we perceive the past, we do not perceive it as past, but as present. Moreover, our experience does not only appear to be temporally limited, it is so: we do not perceive the future, and we do not continue to perceive transient events long after information from them reached our senses. Now, there is a very simple answer to the question why we do not perceive the future, and it is a causal one. Briefly, causes always precede their effects; perception is a causal process, in that to perceive something is to be causally affected by it; therefore we can only perceive earlier events, never later ones. So one temporal boundary of our experience is explained; what of the other?
There seems no logical reason why we should not directly experience the distant past. We could appeal to the principle that there can be no action at a temporal distance, so that something distantly past can only causally affect us via more proximate events. But this is inadequate justification. We can only perceive a spatially distant tree by virtue of its effects on items in our vicinity (light reflected off the tree impinging on our retinas), but this is not seen by those who espouse a direct realist theory of perception as incompatible with their position. We still see the tree , they say, not some more immediate object. Perhaps then we should look for a different strategy, such as the following one, which appeals to biological considerations. To be effective agents in the world, we must represent accurately what is currently going on: to be constantly out of date in our beliefs while going about our activities would be to face pretty immediate extinction. Now we are fortunate in that, although we only perceive the past it is, in most cases, the very recent past, since the transmission of light and sound, though finite, is extremely rapid. Moreover, although things change, they do so, again in most cases, at a rate that is vastly slower than the rate at which information from external objects travels to us. So when we form beliefs about what is going on in the world, they are largely accurate ones. (See Butterfield 1984 for a more detailed account along these lines.) But, incoming information having been registered, it needs to move into the memory to make way for more up to date information. For, although things may change slowly relative to the speed of light or of sound, they do change, and we cannot afford to be simultaneously processing conflicting information. So our effectiveness as agents depends on our not continuing to experience a transient state of affairs (rather in the manner of a slow motion film) once information from it has been absorbed. Evolution has ensured that we do not experience anything other than the very recent past (except when we are looking at the heavens).
To perceive something as present is simply to perceive it: we do not need to postulate some extra item in our experience that is ‘the experience of presentness.’ It follows that there can be no ‘perception of pastness’. In addition, if pastness were something we could perceive, then we would perceive everything in this way, since every event is past by the time we perceive it. But even if we never perceive anything as past (at the same time as perceiving the event in question) we could intelligibly talk more widely of the experience of pastness: the experience we get when something comes to an end. And it has been suggested that memories—more specifically, episodic memories , those of our experiences of past events—are accompanied by a feeling of pastness (see Russell 1921). The problem that this suggestion is supposed to solve is that an episodic memory is simply a memory of an event: it represents the event simpliciter, rather than the fact that the event is past. So we need to postulate something else which alerts us to the fact that the event remembered is past. An alternative account, and one which does not appeal to any phenomenological aspects of memory, is that memories dispose us to form past-tensed beliefs, and is by virtue of this that they represent an event as past.
We have, then, a candidate explanation for our experience of being located at a particular moment in time, the (specious) present. And as the content of that experience is constantly changing, so that position in time shifts. But there is still a further puzzle. Change in our experience is not the same thing as experience of change. We want to know, not just what it is to perceive one event after another, but also what it is to perceive an event as occurring after another. Only then will we understand our experience of the passage of time. We turn, then, to the perception of time order.
How do we perceive precedence amongst events? A temptingly simple answer is that the perception of precedence is just a sensation caused by instances of precedence, just as a sensation of red is caused by instances of redness. Hugh Mellor (1998), who considers this line, rejects it for the following reason. If this were the correct explanation, then we could not distinguish between x being earlier than y , and x being later than y , for whenever there is an instance of one relation, there is also an instance of the other. But plainly we are able to distinguish the two cases, so it cannot simply be a matter of perceiving a relation, but something to do with our perception of the relata. But mere perception of the relata cannot be all there is to perceiving precedence. Consider again Broad’s point about the second hand and the hour hand. We first perceive the hour hand in one position, say pointing to 3 o’clock, and later we perceive it in a different position, pointing to half-past 3. So I have two perceptions, one later than the other. I may also be aware of the temporal relationship of the two positions of the hand. Nevertheless, I do not perceive that relationship, in that I do not see the hand moving. In contrast, I do see the second hand move from one position to another: I see the successive positions as successive.
Mellor’s proposal is that I perceive x precede y by virtue of the fact that my perception of x causally affects my perception of y . As I see the second hand in one position, I have in my short-term memory an image (or information in some form) of its immediately previous position, and this image affects my current perception. The result is a perception of movement. The perceived order of different positions need not necessarily be the same as the actual temporal order of those positions, but it will be the same as the causal order of the perceptions of them. Since causes always precede their effects, the temporal order perceived entails a corresponding temporal order in the perceptions. Dainton (2001) has objected to this that, if the account were right, we should not be able to remember perceiving precedence, since we only remember what we can genuinely perceive. But there seems no reason to deny that, just because perception of precedence may involve short-term memory, it does not thereby count as genuine perception.
There is a further disanalogy between perception of colour and perception of time order. What is perceived in the case of colour is something that has a definite spatio-temporal location. The relation of precedence, in contrast, is not something that has any obvious location. But causes do have locations, so the perception of precedence is rather harder to reconcile with the causal theory of perception than the perception of colour (Le Poidevin 2004, 2007).
In effect, Mellor’s idea is that the brain represents time by means of time: that temporally ordered events are represented by similarly temporally ordered experiences. This would make the representation of time unique. (For example, the brain does not represent spatially separated objects by means of spatially separated perceptions, or orange things by orange perceptions.) But why should time be unique in this respect? In other media, time can be represented spatially (as in cartoons, graphs, and analogue clocks) or numerically (as in calendars and digital clocks). So perhaps the brain can represent time by other means. One reason to suppose that it must have other means at its disposal is that time needs to be represented in memory (I recall, both that a was earlier than b , and also the experience of seeing a occur before b) and intention (I intend to F after I G ), but there is no obvious way in which Mellor’s ‘representation of time by time’ account can be extended to these.
On Mellor’s model, the mechanism by which time-order is perceived is sensitive to the time at which perceptions occur, but indifferent to their content (what the perceptions are of). Daniel Dennett (1991) proposes a different model, on which the process is time-independent, but content-sensitive. For example, the brain may infer the temporal order of events by seeing which sequence makes sense of the causal order of those events. One of the advantages of Dennett’s model is that it can account for the rather puzzling cases of ‘backwards time referral’, where perceived order does not follow the order of perceptions. (See Dennett 1991 for a discussion of these cases, and also Roache 1999 for an attempt to reconcile them with Mellor’s account.)
In giving an account of the various aspects of time perception, we inevitably make use of concepts that we take to have an objective counterpart in the world: the past, temporal order, causation, change, the passage of time and so on. But one of the most important lessons of philosophy, for many writers, is that there may be a gap, perhaps even a gulf, between our representation of the world and the world itself, even on a quite abstract level. (It would be fair to add that, for other writers, this is precisely not the lesson philosophy teaches.) Philosophy of time is no exception to this. Indeed, it is interesting to note how many philosophers have taken the view that, despite appearances, time, or some aspect of time, is unreal. In this final section, we will take a look at how three metaphysical debates concerning the nature of the world interact with accounts of time perception.
The first debate concerns the reality of tense, that is, our division of time into past, present and future. Is time really divided in this way? Does what is present slip further and further into the past? Or does this picture merely reflect our perspective on a reality in which there is no uniquely privileged moment, the present, but simply an ordered series of moments? A-theorists say that our ordinary picture of the world as tensed reflects the world as it really is: the passage of time is an objective fact. B-theorists deny this. (The terms A-theory and B-theory derive from McTaggart’s (1908) distinction between two ways in which events can be ordered in time, either as an A-series—that is in terms of whether they are past, present or future — or as a B-series—that is according to whether they are earlier than, later than, or simultaneous with other events.)
For B-theorists, the only objective temporal facts concern relations of precedence and simultaneity between events. (I ignore here the complications introduced by the Special Theory of Relativity, since B-theory—and perhaps A-theory also—can be reformulated in terms which are compatible with the Special Theory.) B-theorists do not deny that our tensed beliefs, such as the belief that a cold front is now passing, or that Sally’s wedding was two years ago , may be true, but they assert that what makes such beliefs true are not facts about the pastness, presentness or futurity of events, but tenseless facts concerning precedence and simultaneity (see Mellor 1998, Oaklander and Smith 1994). On one version of the B-theory, for example, my belief that there is a cold front now passing is true because the passing of the front is simultaneous with my forming the belief. Now one very serious challenge to the tenseless theorist is to explain why, if time does not pass in reality, it appears to do so. What, in B-theoretic terms, is the basis for our experience as-of the passage of time?
The accounts we considered above, first of the temporal restrictions on our experience, and secondly of our experience of time order, did not explicitly appeal to tensed, or A-theoretic notions. The facts we did appeal to look like purely B-theoretic ones: that causes are always earlier than their effects, that things typically change slowly in relation to the speed of transmission of light and sound, that our information-processing capacities are limited, and that there can be causal connections between memories and experiences. So it may be that the tenseless theorist can discharge the obligation to explain why time seems to pass. But two doubts remain. First, perhaps the A- theorist can produce a simpler explanation of our experience. Second, it may turn out that supposedly B-series facts are dependent upon A-series ones, so that, for example, a and b are simultaneous by virtue of the fact that both are present .
What is clear, though, is that there is no direct argument from experience to the A-theory, since the present of experience, being temporally extended and concerning the past, is very different from the objective present postulated by the A-theory. Further, it cannot be taken for granted that the objective passage of time would explain whatever it is that the experience as-of time’s passage is supposed to amount to. (See Prosser 2005, 2007, 2012, 2016, 2018.)
The second metaphysical issue that has a crucial bearing on time perception is connected with the A/B-theory dispute, and that is the debate between presentists and eternalists. Presentists hold that only the present exists (for an articulation of various kinds of presentism, and the challenges they face, see Bourne 2006), whereas eternalists grant equal reality to all times. the two debates, A- versus B-theory and presentism versus eternalism, do not map precisely onto each other. Arguably, B-theory is committed to eternalism, but A-theorists may not necessarily endorse presentism (though Bourne argues that they should).
How might his be connected to perception? According to the indirect (or, as it is sometimes called, representative) theory of perception, we perceive external objects only by perceiving some intermediate object, a sense datum. According to the direct theory, in contrast, perception of external objects involves no such intermediary. Now, external objects are at varying distances from us, and, as noted above, since light and sound travel at finite speeds, that means that the state of objects that we perceive will necessarily lie in the past. In the case of stars, where the distances are very considerable, the time gap between light leaving the star and our perceiving it may be one of many years. The presentist holds that past states, events and objects are no longer real. But if all that we perceive in the external world is past, then it seems that the objects of our perception (or at least the states of those objects that we perceive) are unreal. It is hard to reconcile this with the direct theory of perception. It looks on the face of it, therefore, that presentists are committed to the indirect theory of perception. (See Power 2010a, 2010b, 2018, Le Poidevin 2015b.)
The third and final metaphysical issue that we will discuss in the context of time perception concerns causal asymmetry. The account of our sense of being located at a time which we considered under Past, present and the passage of time rested on the assumption that causation is asymmetric. Later events, it was suggested, cannot affect earlier ones, as a matter of mind-independent fact, and this is why we do not perceive the future, only the past. But attempts to explain the basis of causal asymmetry, in terms for example of counterfactual dependence, or in probabilistic terms, are notoriously problematic. One moral we might draw from the difficulties of reducing causal asymmetry to other asymmetries is that causal asymmetry is primitive, and so irreducible. Another is that that the search for a mind-independent account is mistaken. Perhaps causation in intrinsically symmetric, but some feature of our psychological constitution and relation to the world makes causation appear asymmetric. This causal perspectivalism is the line taken by Huw Price (1996). That causal asymmetry should be explained in part by our psychological constitution, in a way analogous to our understanding of secondary qualities such as colour, is a radical reversal of our ordinary assumptions, but then our ordinary understanding of a number of apparently objective features of the world—tense, absolute simultaneity—have met with similarly radical challenges. Now, if causal asymmetry is mind-dependent in this way, then we cannot appeal to it in accounting for our experience of temporal asymmetry—the difference between past and future.
Further, it is not at all clear that perspectivalism can account for the perception of time order. The mechanism suggested by Mellor (see Time Order ) exploited the asymmetry of causation: it is the fact that the perception of A causally influences the perception of B, but not vice versa, that gives rise to the perception of A’s being followed by B. We can represent this schematically as follows (where the arrow stands for an asymmetric causal relation):
P(A)→P(B)→P(A<B)
But if there is no objective asymmetry, then what is the explanation? Of course, we can still define causal order in terms of a causal betweenness relation, and we can say that the perceived order follows the objective causal order of the perceptions, in this sense: on the one hand, where A is perceived as being followed by B, then the perception of B is always causally between the perception of A and the perception of A’s being followed by B (the dash represents a symmetric causal relation):
P(A) – P(B) – P(A<B)
On the other hand, where B is perceived as being followed by A, the perception of A is always causally between the perception of B and the perception of B’s being followed by A:
P(B) – P(A)) – P(B<A)
But what, on the causal perspectivalist view, would rule out the following case?
P(B<A) – P(A) – P(B) – P(A<B)
For such a case would satisfy the above constraints. But it is a case in which A is perceived by an observer both as following, and as being followed by, B, and we know that such a case never occurs in experience. ‘Is perceived by x as followed by’ is an asymmetric relation (assuming we are dealing with a single sense modality), and so one that can be grounded in the causal relation only if the causal relation is itself asymmetric. Now if perspectivalism cannot meet the challenge to explain why, when B is perceived as following A, A is never perceived by the same observer as following B, it seems that our experience of time order, insofar as it has a causal explanation, requires causation to be objectively asymmetric.
One strategy the causal perspectivalist could adopt (indeed, the only one available) is to explain the asymmetric principle above in terms of some objective non-causal asymmetry. Price, for example, allows an objective thermodynamic asymmetry, in that an ordered series of states of the universe will exhibit what he calls a thermodynamic gradient: entropy will be lower at one end of the series than at the end. We should resist the temptation to say that entropy increases, for that would be like asserting that a road goes uphill rather than downhill without conceding the perspectival nature of descriptions like ‘uphill’. Could such a thermodynamic asymmetry explain the perception of time order? That is a question for the reader to ponder.
- Arstila, Valtteri, 2018, ‘Temporal Experience without the Specious Present’, Australasian Journal of Philosophy , 92(2): 287–302.
- Augustine, St., Confessions , R.S. Pinecoffin (ed.), Harmondsworth: Penguin, 1961.
- Benovsky, Jiri, 2013, ‘The Present vs The Specious Present’, Review of Philosophy and Psychology , 4(2): 193–203.
- Bourne, Craig, 2006, A Future for Presentism , Oxford: Clarendon Press.
- Broad, C.D., 1923, Scientific Thought , London: Routledge and Kegan Paul.
- Butterfield, Jeremy, 1984, ‘Seeing the Present’, Mind , 93: 161–76; reprinted with corrections in R. Le Poidevin (ed.), Questions of Time and Tense , Oxford: Clarendon Press, 61–75.
- Callender, Craig, 2008, ‘The Common Now’, Philosophical Issues , 18: 339–61.
- Campbell, John, 1994, Past, Space and Self , Cambridge, Mass.: MIT Press.
- Clark, Andy, 1998, ‘Time and Mind’, Journal of Philosophy , 95: 354–76.
- Dainton, Barry, 2000, Stream of Consciousness: unity and continuity in conscious experience , London: Routledge.
- –––, 2001, Time and Space , Chesham: Acumen.
- –––, 2013, ‘The Perception of Time’, in Heather Dyke and Adrian Bardon (eds.), A Companion to the Philosophy of Time , Oxford: Wiley-Blackwell, 389–409.
- Deng, Natalja, 2013a, ‘Our Experience of Time on the B-theory’, Erkenntnis , 78(4): 367–82.
- –––, 2013b, ‘On Explaining Why Time Seems to Pass’, Southern Journal of Philosophy , 51(3): 713–26.
- –––, 2018, ‘On ’Experiencing Time’: A Response to Simon Prosser’, Inquiry , 61 (3): 281–301.
- Dennett, Daniel, 1991, Consciousness Explained , London: Allen Lane.
- Falk, Arthur, 2003, ‘Time plus the Whoosh and Whiz’, in A. Jokic and Q. Smith (eds.), Time, Tense and Reference , Cambridge, Mass.: MIT Press.
- Fotheringham, Heather, 1999, ‘How Long is the Present?’, Stoa , 1(2): 56–65.
- Friedman, William J., 1990, About Time: Inventing the Fourth Dimension , Cambridge, MA: MIT Press.
- Gombrich, Ernst, 1964, ‘Moment and Movement in Art’, Journal of the Warburg and Courtauld Institutes , XXVII: 293–306.
- Grush, Rick, 2003, ‘Brain Time and Phenomenal Time’, in Andrew Brook and Kathleen Akins (eds.), Cognition and the Brain: the philosophy and neuroscience movement , Cambridge: Cambridge University Press.
- Hestevold, H. Scott, 1990, ‘Passage and the Presence of Experience’, Philosophy and Phenomenological Research , 50: 537–52; reprinted in Oaklander and Smith 1994, 328–43
- Hirsh, I.J. and Sherrick, J.E., 1961, ‘Perceived Order in Different Sense Modalities’, Journal of Experimental Psychology , 62: 423–32.
- Hoerl, Christoph, 1998, ‘The Perception of Time and the Notion of a Point of View’, European Journal of Philosophy , 6: 156–71.
- Hoerl, Christoph and McCormack, Teresa (eds.), 2001, Time and Memory: Issues in Philosophy and Psychology , Oxford: Clarendon Press.
- James, William, 1890, The Principles of Psychology , New York: Henry Holt.
- Kelly, Sean D., 2005, ‘The Puzzle of Temporal Experience’, in Andrew Brook and Kathleen Akins (eds.), Cognition and the Brain: the philosophy and neuroscience movement , Cambridge: Cambridge University Press, 208–38.
- Lee, Geoffrey, 2018, ‘Explaining Away Temporal Flow – Thoughts on Prosser’s ’Experiencing Time’’, Inquiry , 61(3): 315–27.
- Le Poidevin, Robin, 1997, ‘Time and the Static Image’, Philosophy , 72: 175–88.
- –––, 1999, ‘Egocentric and Objective Time’, Proceedings of the Aristotelian Society , XCIX: 19–36.
- –––, 2004, ‘ A Puzzle Concerning Time Perception ’, Synthese , 142: 109–42.
- –––, 2007, The Images of Time: An Essay on Temporal Representation , Oxford: Oxford University Press
- –––, 2011, ‘ The Temporal Prison ’, Analysis , 71(3): 456–65.
- –––, 2015a, ‘ Stopped Clocks, Silent Telephones and Sense Data: some problems of time perception’, Topoi , 34: 241–8.
- –––, 2015b, ‘Perception and Time’, in Mohan Matthen (ed.), Oxford Handbook of the Philosophy of Perception , Oxford: Wiley-Blackwell.
- –––, 2017, ‘ The Arrow of Mind ’, Journal of Consciousness Studies , 24(3-4): 112–26.
- Mabbott, J.D., 1951, ‘Our Direct Experience of Time’, Mind , 60: 153–67.
- Mackintosh, N.J., 1983, Conditioning and Associative Learning , Oxford: Clarendon Press.
- Martin, M.G.F., 2001, ‘Out of the Past: episodic recall as retained acquaintance’, in Hoerl and McCormack 2001, 257–84.
- Mayo, Bernard, 1950, ‘Is There a Sense of Duration?’, Mind , 59: 71–8.
- Mellor, D.H., 1998, Real Time II , London: Routledge.
- Mundle, C.W.K., 1966, ‘Augustine’s Pervasive Error Concerning Time’, Philosophy , 41: 165–8.
- Myers, Gerald, 1971, ‘James on Time Perception’, Philosophy of Science , 38: 353–60
- Oaklander, L. Nathan, 1993, ‘On the Experience of Tenseless Time’, Journal of Philosophical Research , 18: 159–66; reprinted in Oaklander and Smith 1994, 344–50.
- –––, 2002, ‘Presentism, Ontology and Temporal Experience’, in C. Callender (ed.), Time, Reality and Experience , Cambridge: Cambridge University Press.
- Oaklander, L. Nathan, and Smith, Quentin (eds.), 1994, The New Theory of Time , New Haven: Yale University Press.
- Odegard, D., 1978, ‘Phenomenal Time’, Ratio , 20: 116–22.
- Ornstein, R.E., 1969, On the Experience of Time , Harmondsworth: Penguin.
- Paul, L.A., 2010, ‘Temporal Experience’, Journal of Philosophy , 107(2): 333–59.
- Phillips, Ian, 2008, ‘Perceiving Temporal Properties’, European Journal of Philosophy , 18(2): 176–202.
- –––, 2012, ‘Attention to the Passage of Time’, Philosophical Perspectives , 26(2): 176–202.
- –––, 2014, ‘Experience Of and In Time’, Philosophy Compass , 9(2): 131–144.
- Plumer, Gilbert, 1985, ‘The Myth of the Specious Present’, Mind , 94: 277–308.
- –––, 1987, ‘Detecting Temporalities’, Philosophy and Phenomenological Research , 47: 451–60.
- Pöppel, Ernst, 1978, ‘Time Perception’, in Richard Held et al . (eds.), Handbook of Sensory Physiology , Vol. VIII: Perception, Berlin: Springer-Verlag.
- Power, Sean Enda, 2010a, ‘Complex Experience, Relativity and Abandoning Simultaneity’, Journal of Consciousness Studies , 17: 231–56.
- –––, 2010b, ‘Perceiving External Things and the Time-Lag Argument’, European Journal of Philosophy , 21(1): 94–117.
- –––, 2012, ‘The Metaphysics of the ‘Specious’ Present’, Erkenntnis , 17(1): 121–32.
- –––, 2018, Philosophy of Time and Perceptual Experience , London: Routledge.
- Price, Huw, 1996, Time’s Arrow and Archimedes’ Point: New Directions in the Physics of Time , Oxford: Oxford University Press.
- Prosser, Simon, 2005, ‘Cognitive Dynamics and Indexicals’, Mind and Language , 20: 369–91.
- –––, 2007, ‘Could We Experience the Passage of Time?’, Ratio , 20(1): 75–90.
- –––, 2011, ‘Why Does Time Seem to Pass?’, Philosophy and Phenomenological Research , 85 (1): 92–116.
- –––, 2016, Experiencing Time , Oxford: Oxford University Press.
- –––, 2018, ‘Replies to Deng, Lee and Skow’, Inquiry , 61(3): 328–50.
- Roache, Rebecca, 1999, ‘Mellor and Dennett on the Perception of Temporal Order’, Philosophical Quarterly , 49: 231–38.
- Russell, Bertrand, 1915, ‘On the Experience of Time’, Monist , 25: 212–33.
- –––, 1921, The Analysis of Mind , London: George Allen and Unwin.
- Skow, Bradford, 2011 ‘Experience and the Passage of Time’, Philosophical Perspectives , 25(1): 359–87.
- –––, 2015, Objective Becoming , Oxford: Oxford University Press.
- –––, 2018, ‘Some Thoughts On Experiencing Time ’, Inquiry , 61(3): 302–14.
- Smith, Quentin, 1988, ‘The Phenomenology of A-Time’, Diálogos , 52: 143–53; reprinted in Oaklander and Smith 1994, 351–9.
- Walsh, W.H., 1967, ‘Kant on the Perception of Time’, Monist , 51: 376–96.
- Williams, Clifford, 1992, ‘The Phenomenology of B-Time’, Southern Journal of Philosophy , 30: 123-37; reprinted in Oaklander and Smith 1994, 360–72.
How to cite this entry . Preview the PDF version of this entry at the Friends of the SEP Society . Look up topics and thinkers related to this entry at the Internet Philosophy Ontology Project (InPhO). Enhanced bibliography for this entry at PhilPapers , with links to its database.
[Please contact the author with suggestions.]
Augustine, Saint | consciousness: temporal | memory | perception: the problem of | presentism | space and time: being and becoming in modern physics | time
Copyright © 2019 by Robin Le Poidevin < r . d . lepoidevin @ leeds . ac . uk >
Support SEP
Mirror sites.
View this site from another server:
- Info about mirror sites
The Stanford Encyclopedia of Philosophy is copyright © 2021 by The Metaphysics Research Lab , Department of Philosophy, Stanford University
Library of Congress Catalog Data: ISSN 1095-5054
- Subject List
- Take a Tour
- For Authors
- Subscriber Services
- Publications
- African American Studies
- African Studies
- American Literature
- Anthropology
- Architecture Planning and Preservation
- Art History
- Atlantic History
- Biblical Studies
- British and Irish Literature
- Childhood Studies
- Chinese Studies
- Cinema and Media Studies
- Communication
- Criminology
- Environmental Science
- Evolutionary Biology
- International Law
- International Relations
- Islamic Studies
- Jewish Studies
- Latin American Studies
- Latino Studies
- Linguistics
- Literary and Critical Theory
- Medieval Studies
- Military History
- Political Science
- Public Health
- Renaissance and Reformation
- Social Work
- Urban Studies
- Victorian Literature
- Browse All Subjects
How to Subscribe
- Free Trials
In This Article Expand or collapse the "in this article" section Time Perception
Introduction, general overviews.
- Reference Resources
- Bibliographies
- Early Articles on Time Perception, 315 bce –1891 ce
- Subsequent Research on Time Perception, 1892–1956
- Origins of Modern Research on Time Perception, 1957–1964
- Late 20th and Early 21st Century, 1965–Present
- Time Perception Methods
- Psychophysics of Time Perception
- Scalar Expectancy Theory (SET)
- The Influence of Attention on Time Perception
- Time Present (Experienced Duration)
- Time Past (Remembered Duration)
- Past Time: Autobiographical Memory and Recency Judgments
- Future Time: Prospective Memory
- Theoretical Views
- Circadian Timing and Related Duration Experience
- Neurophysiological and Neuropsychological Findings
- Neurotransmitter and Psychopharmacological Findings
- Electroencephalographic and Neuroimaging Findings
- Developmental, Gender, Social, and Cross-Cultural Differences
- Aging and Time Perception
- Applied Psychology of Time Perception
Related Articles Expand or collapse the "related articles" section about
About related articles close popup.
Lorem Ipsum Sit Dolor Amet
Vestibulum ante ipsum primis in faucibus orci luctus et ultrices posuere cubilia Curae; Aliquam ligula odio, euismod ut aliquam et, vestibulum nec risus. Nulla viverra, arcu et iaculis consequat, justo diam ornare tellus, semper ultrices tellus nunc eu tellus.
- Autobiographical Memories
- Event Perception
- Research Methods
Other Subject Areas
Forthcoming articles expand or collapse the "forthcoming articles" section.
- Data Visualization
- Executive Functions in Childhood
- Remote Work
- Find more forthcoming articles...
- Export Citations
- Share This Facebook LinkedIn Twitter
Time Perception by Richard A. Block , Peter A. Hancock LAST REVIEWED: 13 January 2014 LAST MODIFIED: 13 January 2014 DOI: 10.1093/obo/9780199828340-0123
The term time perception refers to a large subfield within the more general study of the psychology of time. It is an old and venerable topic in psychology. When psychology emerged from philosophy and medicine in the late 1800s, time perception became a major topic of interest. Researchers investigated many aspects of the psychology of time, especially the relationships between psychological and “real” (physical) time. Later, in about 1920, the tide turned: in the United States, behavioral psychologists asserted that psychologists should not investigate such topics. European psychologists did not agree, and they continued to investigate time perception, as they still do. Beginning in the 1960s, however, time psychologists started to be influential in the mainstream, even in the United States. They investigated how time perception involves many other processes. They began to integrate time perception along with attention, memory, and other cognitive psychological topics. After about the 1960s, and continuing to the present, time perception has seen a resurgence of interest. Now even an American time researcher can hold her or his head up and be proud to say, “I’m back.” This article focuses on the history and resurgence of time perception instead of the much more diverse topics of the psychology of time. Thus, we are excluding many references to clinical, pathology, personality, social, and other aspects of the psychology of time. This article includes only a few citations in those other subareas of psychology, mostly those that relate directly to time perception. The article presents a selective list of citations, undoubtedly omitting many important ones from hundreds of researchers, for anyone to get started on this fascinating topic. Given the selective nature of this article, there is only a limited number of publications can be included out of more than 13,000 of the journal articles, book chapters, and books published from the 1860s through the 2010s—more than 150 years of time perception research.
General overviews have been published in many edited books and chapters. Some of the more recent and influential are noted here. In chronological order: the edited volume Michon and Jackson 1985 , from a conference in The Netherlands, is still of interest. McGrath 1986 is a mostly social psychological book. Block 1990 is a book on cognitive models, which is still relevant. Friedman 1990 revealed how time experience relies on different processes and that it is important to understand temporal experience as involving separate components. Macar, et al. 1992 contains edited chapters based on presentations at a conference in France, and these are still worth reading. Helfrich 2003 is an important book, along with an earlier one, from two conferences in Germany. Meck 2003 focused on scalar timing and neural mechanisms, and it is also important to time researchers. More recently, Grondin 2008 contains many important chapters. Contemporary researchers of time psychophysics, time perception, and time cognition will want to read most of the chapters in it.
Block, R. A., ed. 1990. Cognitive models of psychological time . Hillsdale, NJ: Erlbaum.
Eleven diverse chapters relating to time perception from a cognitive perspective. Widely cited.
Friedman, W. J. 1990. About time: Inventing the fourth dimension . Cambridge, MA: MIT Press.
An interesting book by a leading researcher, who mainly (but not only) focused on developmental psychology and time.
Grondin, S., ed. 2008. Psychology of time . Bingley, UK: Emerald.
Intentionally titled after the famous French psychologist’s single-authored book ( Fraisse 1963 , cited under Origins of Modern Research on Time Perception, 1957–1964 ), this is an important edited volume with thirteen chapters to read.
Helfrich, H., ed. 2003. Time and mind II: Information processing perspectives . Papers presented at the International Symposium on Time and Mind II, University of Hildesheim, 2–4 September 2002. Göttingen, Germany: Hogrefe & Huber.
Chapters resulting from the second of two conferences in Germany. Arguably the better volume, containing articles on time perception and other related issues. For articles from the first conference, see H. Helfrich (ed.), Time and Mind (Seattle, WA: Hogrefe & Huber, 1996).
Macar, F., V. Pouthas, and W. J. Friedman, eds. 1992. Time, action and cognition: Towards bridging the gap . Papers presented at the NATO Advanced Research Workshop on Time, Action and Cognition, Saint-Malo, France, 22–25 October 1991. NATO ASI series. Dordrecht, The Netherlands: Kluwer Academic.
DOI: 10.1007/978-94-017-3536-0
Many articles inspired by a conference in Saint-Malo, France, which was organized in honor of Paul Fraisse, appeared in this important volume.
McGrath, J. E., ed. 1986. Research toward a psychology of time . Beverly Hills, CA: SAGE.
Mostly social psychologically oriented.
Meck, W. H., ed. 2003. Functional and neural mechanisms of interval timing . Methods and New Frontiers in Neuroscience. Boca Raton, FL: CRC.
DOI: 10.1201/9780203009574
A very important edited collection of articles on neural processes and timing behavior. Many of the articles concern interval timing. Any researcher interested in the Scalar Expectancy Theory (SET) —and how brains time intervals—will want to read this book.
Michon, J. A., and J. L. Jackson, eds. 1985. Time, mind, and behavior . Papers presented at the International Workshop on Time, Mind, and Behavior, University of Groningen, September 1984. Berlin: Springer-Verlag.
DOI: 10.1007/978-3-642-70491-8
Chapters arising from an important early conference in Groningen, The Netherlands. Michon’s chapter provided a nice overview of temporal experience: “The Compleat Time Experiencer” (pp. 20–52).
back to top
Users without a subscription are not able to see the full content on this page. Please subscribe or login .
Oxford Bibliographies Online is available by subscription and perpetual access to institutions. For more information or to contact an Oxford Sales Representative click here .
- About Psychology »
- Meet the Editorial Board »
- Abnormal Psychology
- Academic Assessment
- Acculturation and Health
- Action Regulation Theory
- Action Research
- Addictive Behavior
- Adolescence
- Adoption, Social, Psychological, and Evolutionary Perspect...
- Advanced Theory of Mind
- Affective Forecasting
- Affirmative Action
- Ageism at Work
- Allport, Gordon
- Alzheimer’s Disease
- Ambulatory Assessment in Behavioral Science
- Analysis of Covariance (ANCOVA)
- Animal Behavior
- Animal Learning
- Anxiety Disorders
- Art and Aesthetics, Psychology of
- Artificial Intelligence, Machine Learning, and Psychology
- Assessment and Clinical Applications of Individual Differe...
- Attachment in Social and Emotional Development across the ...
- Attention-Deficit/Hyperactivity Disorder (ADHD) in Adults
- Attention-Deficit/Hyperactivity Disorder (ADHD) in Childre...
- Attitudinal Ambivalence
- Attraction in Close Relationships
- Attribution Theory
- Authoritarian Personality
- Bayesian Statistical Methods in Psychology
- Behavior Therapy, Rational Emotive
- Behavioral Economics
- Behavioral Genetics
- Belief Perseverance
- Bereavement and Grief
- Biological Psychology
- Birth Order
- Body Image in Men and Women
- Bystander Effect
- Categorical Data Analysis in Psychology
- Childhood and Adolescence, Peer Victimization and Bullying...
- Clark, Mamie Phipps
- Clinical Neuropsychology
- Clinical Psychology
- Cognitive Consistency Theories
- Cognitive Dissonance Theory
- Cognitive Neuroscience
- Communication, Nonverbal Cues and
- Comparative Psychology
- Competence to Stand Trial: Restoration Services
- Competency to Stand Trial
- Computational Psychology
- Conflict Management in the Workplace
- Conformity, Compliance, and Obedience
- Consciousness
- Coping Processes
- Correspondence Analysis in Psychology
- Counseling Psychology
- Creativity at Work
- Critical Thinking
- Cross-Cultural Psychology
- Cultural Psychology
- Daily Life, Research Methods for Studying
- Data Science Methods for Psychology
- Data Sharing in Psychology
- Death and Dying
- Deceiving and Detecting Deceit
- Defensive Processes
- Depressive Disorders
- Development, Prenatal
- Developmental Psychology (Cognitive)
- Developmental Psychology (Social)
- Diagnostic and Statistical Manual of Mental Disorders (DSM...
- Discrimination
- Dissociative Disorders
- Drugs and Behavior
- Eating Disorders
- Ecological Psychology
- Educational Settings, Assessment of Thinking in
- Effect Size
- Embodiment and Embodied Cognition
- Emerging Adulthood
- Emotional Intelligence
- Empathy and Altruism
- Employee Stress and Well-Being
- Environmental Neuroscience and Environmental Psychology
- Ethics in Psychological Practice
- Evolutionary Psychology
- Expansive Posture
- Experimental Existential Psychology
- Exploratory Data Analysis
- Eyewitness Testimony
- Eysenck, Hans
- Factor Analysis
- Festinger, Leon
- Five-Factor Model of Personality
- Flynn Effect, The
- Forensic Psychology
- Forgiveness
- Friendships, Children's
- Fundamental Attribution Error/Correspondence Bias
- Gambler's Fallacy
- Game Theory and Psychology
- Geropsychology, Clinical
- Global Mental Health
- Habit Formation and Behavior Change
- Health Psychology
- Health Psychology Research and Practice, Measurement in
- Heider, Fritz
- Heuristics and Biases
- History of Psychology
- Human Factors
- Humanistic Psychology
- Implicit Association Test (IAT)
- Industrial and Organizational Psychology
- Inferential Statistics in Psychology
- Insanity Defense, The
- Intelligence
- Intelligence, Crystallized and Fluid
- Intercultural Psychology
- Intergroup Conflict
- International Classification of Diseases and Related Healt...
- International Psychology
- Interviewing in Forensic Settings
- Intimate Partner Violence, Psychological Perspectives on
- Introversion–Extraversion
- Item Response Theory
- Law, Psychology and
- Lazarus, Richard
- Learned Helplessness
- Learning Theory
- Learning versus Performance
- LGBTQ+ Romantic Relationships
- Lie Detection in a Forensic Context
- Life-Span Development
- Locus of Control
- Loneliness and Health
- Mathematical Psychology
- Meaning in Life
- Mechanisms and Processes of Peer Contagion
- Media Violence, Psychological Perspectives on
- Mediation Analysis
- Memories, Autobiographical
- Memories, Flashbulb
- Memories, Repressed and Recovered
- Memory, False
- Memory, Human
- Memory, Implicit versus Explicit
- Memory in Educational Settings
- Memory, Semantic
- Meta-Analysis
- Metacognition
- Metaphor, Psychological Perspectives on
- Microaggressions
- Military Psychology
- Mindfulness
- Mindfulness and Education
- Minnesota Multiphasic Personality Inventory (MMPI)
- Money, Psychology of
- Moral Conviction
- Moral Development
- Moral Psychology
- Moral Reasoning
- Nature versus Nurture Debate in Psychology
- Neuroscience of Associative Learning
- Nonergodicity in Psychology and Neuroscience
- Nonparametric Statistical Analysis in Psychology
- Observational (Non-Randomized) Studies
- Obsessive-Complusive Disorder (OCD)
- Occupational Health Psychology
- Older Workers
- Olfaction, Human
- Operant Conditioning
- Optimism and Pessimism
- Organizational Justice
- Parenting Stress
- Parenting Styles
- Parents' Beliefs about Children
- Path Models
- Peace Psychology
- Perception, Person
- Performance Appraisal
- Personality and Health
- Personality Disorders
- Personality Psychology
- Person-Centered and Experiential Psychotherapies: From Car...
- Phenomenological Psychology
- Placebo Effects in Psychology
- Play Behavior
- Positive Psychological Capital (PsyCap)
- Positive Psychology
- Posttraumatic Stress Disorder (PTSD)
- Prejudice and Stereotyping
- Pretrial Publicity
- Prisoner's Dilemma
- Problem Solving and Decision Making
- Procrastination
- Prosocial Behavior
- Prosocial Spending and Well-Being
- Protocol Analysis
- Psycholinguistics
- Psychological Literacy
- Psychological Perspectives on Food and Eating
- Psychology, Political
- Psychoneuroimmunology
- Psychophysics, Visual
- Psychotherapy
- Psychotic Disorders
- Publication Bias in Psychology
- Reasoning, Counterfactual
- Rehabilitation Psychology
- Relationships
- Reliability–Contemporary Psychometric Conceptions
- Religion, Psychology and
- Replication Initiatives in Psychology
- Risk Taking
- Role of the Expert Witness in Forensic Psychology, The
- Sample Size Planning for Statistical Power and Accurate Es...
- Schizophrenic Disorders
- School Psychology
- School Psychology, Counseling Services in
- Self, Gender and
- Self, Psychology of the
- Self-Construal
- Self-Control
- Self-Deception
- Self-Determination Theory
- Self-Efficacy
- Self-Esteem
- Self-Monitoring
- Self-Regulation in Educational Settings
- Self-Report Tests, Measures, and Inventories in Clinical P...
- Sensation Seeking
- Sex and Gender
- Sexual Minority Parenting
- Sexual Orientation
- Signal Detection Theory and its Applications
- Simpson's Paradox in Psychology
- Single People
- Single-Case Experimental Designs
- Situational Strength
- Skinner, B.F.
- Sleep and Dreaming
- Small Groups
- Social Class and Social Status
- Social Cognition
- Social Neuroscience
- Social Support
- Social Touch and Massage Therapy Research
- Somatoform Disorders
- Spatial Attention
- Sports Psychology
- Stanford Prison Experiment (SPE): Icon and Controversy
- Stereotype Threat
- Stereotypes
- Stress and Coping, Psychology of
- Student Success in College
- Subjective Wellbeing Homeostasis
- Taste, Psychological Perspectives on
- Teaching of Psychology
- Terror Management Theory
- Testing and Assessment
- The Concept of Validity in Psychological Assessment
- The Neuroscience of Emotion Regulation
- The Reasoned Action Approach and the Theories of Reasoned ...
- The Weapon Focus Effect in Eyewitness Memory
- Theory of Mind
- Therapy, Cognitive-Behavioral
- Thinking Skills in Educational Settings
- Time Perception
- Trait Perspective
- Trauma Psychology
- Twin Studies
- Type A Behavior Pattern (Coronary Prone Personality)
- Unconscious Processes
- Video Games and Violent Content
- Virtues and Character Strengths
- Women and Science, Technology, Engineering, and Math (STEM...
- Women, Psychology of
- Work Well-Being
- Workforce Training Evaluation
- Wundt, Wilhelm
- Privacy Policy
- Cookie Policy
- Legal Notice
- Accessibility
Powered by:
- [66.249.64.20|195.190.12.77]
- 195.190.12.77
An official website of the United States government
Official websites use .gov A .gov website belongs to an official government organization in the United States.
Secure .gov websites use HTTPS A lock ( Lock Locked padlock icon ) or https:// means you've safely connected to the .gov website. Share sensitive information only on official, secure websites.
- Publications
- Account settings
- Advanced Search
- Journal List

Flow of Time: Perceiving the passage of time: neural possibilities
Timothy muller, anna c nobre.
- Author information
- Article notes
- Copyright and License information
Address for correspondence: Anna C. Nobre, Department of Experimental Psychology, University of Oxford, South Parks Road, Oxford Oxfordshire OX1 3UD, United Kingdom. [email protected]
Issue date 2014 Oct.
This is an open access article under the terms of the Creative Commons Attribution License, which permits use, distribution and reproduction in any medium, provided the original work is properly cited.
Although the study of time has been central to physics and philosophy for millennia, questions of how time is represented in the brain and how this representation is related to time perception have only recently started to be addressed. Emerging evidence subtly yet profoundly challenges our intuitive notions of time over short scales, offering insight into the nature of the brain's representation of time. Numerous different models, specified at the neural level, of how the brain may keep track of time have been proposed. These models differ in various ways, such as whether time is represented by a centralized or distributed neural system, or whether there are neural systems dedicated to the problem of timing. This paper reviews the insight offered by behavioral experiments and how these experiments refute and guide some of the various models of the brain's representation of time.
Keywords: brain, time, causality, temporal illusion
Introduction
Whether time exists or not, it cannot be denied that we subjectively perceive a passage of time. This raises the question of how the brain generates the subjective sense of time. We have no “time receptors,” as we do for other features of perception, such as shape, color, and pitch—so how does the brain keep track of time? This issue is of great importance, since timing is essential to all aspects of behavior: from structuring actions to planning the events in our lives. It is perhaps, therefore, surprising that the neural underpinnings of timing have remained relatively unexplored, for example, in comparison to the investigation of spatial cognition. As neuroscience begins to grapple with the fourth dimension, different possible mechanisms for neural timekeeping are beginning to be proposed and investigated. These models, along with psychophysical experiments that inform them, will be discussed in this review.
Conceptions of time in physics and philosophy
Time is arguably one of most difficult entities to define. Indeed, even labelling it as an “entity” is loaded, since it implies it has an existence per se. Its elusive nature can be gleaned from a brief sketch of some notions of time offered by philosophy and physics.
Perhaps most appealing to our intuitions is the notion held by Newton stating that time exists: time is “absolute, true, and mathematical, in and of itself and of its own nature, without reference to anything external, flows uniformly and by another name is called duration.” 1 This view states that time flows in a predictable manner, as we believe we perceive it.
A contrasting view with origins dating back to the sophists, then articulated by Leibniz, states that time has no independent existence, but rather it is “merely relative… an order of successions.” 2 This view of time, as the structured relation among entities, rather than an entity itself, was taken further by Kant, who proposed that time, like space, had no external existence at all, but was instead one of the core organizing principles through which we organize sense data into a coherent perception. 3 Yet stranger notions of time are captured in Einstein's theories of special and general relatively, positing that time is deeply meshed with space into a dynamic and curving space-time fabric. 4
Concepts of time in the brain
A few key concepts frame the literature on how time is represented in the brain. The first issue is whether time is represented in a centralized system 5 – 7 or is distributed throughout the brain. The second issue is whether there are specialized neural systems exclusively dedicated to the problem of timing. 8 , 9 Such a system could be defined as a dedicated “clock” within the brain. Dedicated timing mechanisms are contrasted with the alternative view that the circuits responsible for timing primarily process other information, such as other stimulus attributes 10 or motor programs, 11 but also intrinsically represent time. In this case, neural processes occur in time, without necessarily coding time per se. As is discussed in more detail below, the time dependency of these processes can give the illusion that the process is the coding of time per se. Therefore, apparently dedicated timing mechanisms may in fact be incidental to the processing of other attributes. This distinction between dedicated versus intrinsic timing somewhat echoes the distinction between absolute, Newtonian time and relational, Leibnizian or Kantian time, respectively: time that is not extracted from its context is by definition not “in and of itself…without reference to anything external,” but rather defined by the process performing the function in which time is intrinsically processed.
It is noteworthy that, while it may appear intuitive for centralized timing mechanisms to be dedicated and for distributed timing mechanisms to be intrinsic, these are orthogonal principles and could theoretically exist in any combination.
Finally, different time scales may be mediated by different mechanisms. 7 In this review, we will focus on the time scales associated with sensing the passage of time, as measured through tasks requiring judgments of durations or temporal relations among events, in the millisecond-to-second range. Neural timing over this “interval” scale is not well understood, in contrast to circadian rhythms thought to be timed by the suprachiasmatic nucleus using transcriptional feedback loops to generate an approximate 24-h cycle of activity. 11
Psychological challenges for a neural clock
There are a few strong motivations for dedicated, centralized timing mechanisms. First, the sense of the passage of time transcends modalities, evidenced by our ability to measure duration across the senses: 9 , 12 , 13 for example, our ability to compare the duration of a tone to a light, or to reproduce the duration of a visual stimulus with a motor response. 12 This is suggestive of a common underlying mechanism. Second, individual differences in temporal acuity correlate across perception and action, 14 , 15 similarly suggesting a common underlying mechanism. Finally, such a dedicated timing mechanism appeals to our intuitions of a consistent, linear passage of time.
However, numerous illusions highlighting distortions in our perception of the passage of time challenge such intuitions. Surprising experimental evidence comes from the study of rapid eye movements (saccades), which we use to foveate objects of interest in the environment. When two bars are flashed sequentially onto a screen around the time that individuals saccade horizontally from one visual target to another, the individuals perceive the duration of separation of the two bars to be significantly shorter than it actually is. 16 Importantly, perception of auditory clicks presented similarly around saccadic onset suffers no distortion of time. Such evidence is not compatible with a common, unitary sense of linear time, since that would predict that the perception of time across sensory modalities should be unperturbed and equivalent.
In the same vein, it has been demonstrated that, even within the visual modality, temporal perception of different events can be differentially distorted. 17 – 19 For example, maintaining gaze on a moving stimulus in one portion of the visual field reduces the apparent duration of stimuli subsequently presented to that location, but not to other locations. 17 Therefore, temporal perception in any given spatial region of the visual field is at least partly independent of temporal perception in other regions––again incompatible with a common, centralized representation of time in the brain.
Additional profound illusions come from studies investigating the perceived duration between one's action and its inferred effect. When individuals are asked to press a button to “cause” a tone, the estimated perceived time between the button press and the tone is reliably shorter than the actual time. 20 This temporal-compression effect does not occur when the button press and tone occur with identical temporal parameters, but individuals do not believe that their action caused the tone (i.e., when the tones are stated to be randomly generated through a computer program). One prevalent interpretation is that the anticipated, intended consequence of the action is brought closer in time, leading to the labeling of this phenomenon “intentional binding.” 20 However, some dispute remains over whether perceived intentionality is required or whether the mere perception of causality is sufficient. 21 , 22
Perceptual distortions between action and inferred effect are also subject to contextual effects of adaptation, which allows the power of these illusions to be revealed. Stetson et al . 23 designed an experiment whereby participants adapted to the delay between pressing a button and perceiving a flash caused by that button press. As before, temporal binding was observed between action and consequence. However, when the adapted delay was suddenly removed, such that the flash occurred almost immediately after the button press, participants perceived the flash as occurring before their action that caused it. Such an observation is clearly incompatible with perception being a representation of linearly flowing time, since time becomes inverted to the observer.
Adaptation to temporal delays is further demonstrated in experiments manipulating the timing of information from different sensory modalities. The perception of an external event through more than one sensory channel raises an interesting problem: different sensory channels receive and process information at different speeds, and therefore our perception of these events through different channels should be asynchronous. Indeed, when individuals are asked to judge the order or simultaneity of separate brief events perceived through different sensory modalities (for example, a burst of sound and a flash of light), it becomes possible to observe that different sensory channels have different lags to reach perceptual awareness. 24 However, when we click our fingers or watch someone speaking, we perceive the visual (movement of the fingers, movement of the lips) and auditory input (“snap,” “hello”) to be synchronous. They are correctly perceived to be caused by the same external “event,” despite the lags in the different sensory channels. How has the brain solved this problem? It has been suggested that the perceived timing of different sources is dynamically calibrated, such that the multisensory perception of an external event comes to be unitary.
There is empirical evidence for such a calibration process. When participants are exposed to a fixed time lag between auditory and visual stimulation for 3 min, they subsequently show shifts in the time required between auditory and visual stimuli in order to perceive them as simultaneous, known as the “point of subjective simultaneity.” 25 In other words, participants have adapted to the delay. These adaptation effects also transfer onto other perceptual tasks, indicating that it is a genuine perceptual change. Analogous results have been demonstrated in the context of speech: the point at which participants judge the audio and visual components of audiovisual speech to be simultaneous shifts depending on whether they were previously exposed to asynchronous or synchronous audiovisual components of speech. 26 Therefore, temporal calibration occurs in the context of simple audiovisual stimuli as well as in more complex and dynamic speech perception in order to generate a unitary perception of stimuli arising from a common external event. Although this does help our perception of external events appear veridical, it requires adaptation processes that distort temporal processing. Again, this is incompatible with a constant mechanism of linear time supporting perception.
Causation and probability
A common factor that may govern motor–sensory and sensory–sensory temporal distortions is the interpretation of causal relations among events. As alluded to above, the interpretation of causality, and not necessarily intentional action, may give rise to the temporal compression observed between actions and their inferred effects. A string of experiments supports this. 21 , 22 , 27 For example, by manipulating the belief of causality while controlling for all other variables, Buehner and Humphreys 21 demonstrated that perceived causality between action and effect is sufficient for temporal compression. Further, when the actor is a mechanical device (an arm of a small machine), instead of self-generated actions, temporal compression still occurs when a causal relation is assumed. 22 Similarly, the belief that two percepts of different modalities are caused by the same external event may be responsible for driving temporal calibration of sensory channels. There is evidence to support this: if participants believe that two sensory percepts originate from a common event, they are more likely to be perceived as simultaneous. 28 – 31
The role played by “causality” in temporal compression may have its roots in our prior experience with the probable temporal relations between events. Hume proposed that events that are close in space and time are more likely to be perceived as causally related than events further apart. 32 Drawing insight captured by Bayes’ theorem, events believed to be causally related are more likely to be spatiotemporally close than those that are not. Given some uncertainty in the temporal estimate of an event, the updated temporal estimate will be biased in time toward the other, causally related event. 33 This Bayesian causal-binding explanation predicts that causal beliefs can lead to temporal compression, but also that temporal compression should be stronger for action–effect pairings that are closer in time, as observed by Haggard et al . 20
The possibility that temporal calibrations are Bayesian in nature has been formally investigated in the context of prior experience. When participants are asked to judge the temporal order of tactile stimuli delivered one to each hand, such that one hand is more likely to receive stimulation before the other, the perceived timing of stimulation follows a shift predicted by a Bayesian integrator. 34 In other words, learning one hand is more likely to be stimulated before the other biases observers, making them more likely to select stimulation of that hand as occurring before the other in future trials. Analogous mechanisms have been found for audiovisual recalibration. 35 Importantly, the belief biasing temporal estimates is prior experience of delay, without any necessary mediating role by causal beliefs.
Another important factor influencing calibration is our interaction with the world. When an event is far away, audiovisual asynchrony occurs because of the slower velocity of sound (e.g., fireworks). Parsons et al . 28 demonstrated that active control over a distant audiovisual event renders the auditory and visual percepts more likely to be perceived as simultaneous: we can send out a motor action and analyze the returning multimodal sensations in order to calibrate the relative timing of different modalities. This proposal is rooted in spatial cognition: when individuals wear left–right inverting prism glasses, such that objects on the left appear on the right and vice versa, their vision is highly distorted. However, if allowed to interact with the world by reaching out and touching objects, adaptation occurs and objects on the left appear on the left again. 36 , 37 Therefore, in both temporal and spatial recalibration, motor interactions with the world, and the feedback received from them, allow dynamic recalibration of our percepts.
It is therefore clear that our perception of time can be tuned dynamically, and can often be highly and locally distorted, at least over a timescale of under a second. This can be observed in the context of foveating events with saccadic eye movements, temporal compression of action consequences, or calibration of different sensory modalities. Therefore, instead of time perception following a constant linear mechanism, it is flexible, dynamic, and continually retuned.
Neural substrates
Numerous different models of how the brain may keep track of time have been proposed. As noted above, these can be broadly classified as either centralized or distributed, and by the type of timing they address.
Centralized timing mechanisms
A single, dedicated, centralized, and supramodal clock is the closest model of timing in the brain to keep track of a Newtonian-like “absolute” time. By far the most influential such model is the pacemaker–accumulator model based on an information-processing model of scalar timing theory, consisting of three key components: clock, memory, and decision stages. 38 A stimulus triggers the accumulator to start counting “ticks,” emitted from the pacemaker based on some sort of periodic neural process, until the end of the timed duration (clock component). The number of counted ticks is then compared in working memory to those previously stored in a reference memory to establish a match (memory component), and thereby make a decision about the duration of the interval (decision component). Timing in this model is therefore achieved by reading out from a timeline generated by the pacemaker.
The pacemaker–accumulator model satisfies the phenomenological motivations discussed above by serving as a centralized, supramodal clock dedicated to timing across all domains. It appeals to our intuitions of a linear passage of time. However, on its own, it is unable to account for the common illusions of temporal perception described above, and support for a direct neural implementation of this model is diminishing.
The pacemaker–accumulator model does have some capacity for flexibility or distortion. For example, it can offer explanation of how states of hyperarousal (e.g., road traffic accidents) are associated with time dilation, since arousal could cause an increased rate of ticks emitted from the pacemaker, accumulating and causing an overestimation of passed time. It can additionally account for how paying attention to the passage of time can influence its apparent rate of passage: attention directed away from the passage of time could cause ticks to be missed by the accumulator, and hence time is underestimated, accounting for how “time flies when you're having fun.” Similarly, focusing on the passage of time could account for how “a watched pot never boils.” 39 – 41
More problematic for the pacemaker–accumulator model are local distortions in time perception or the temporal alignment between different modalities, including reversals of temporal perception. It allows only for a global speeding or slowing of time perception. It is therefore not possible that a single, centralized timing mechanism is responsible for time perception. Minimally, if a centralized timing mechanism exists, it must interact with other, more localized mechanisms before the perception of timing occurs. 42 , 43
However, that such a clock cannot account for all mechanisms of time perception in the brain does not rule out a centralized timing system altogether. 43 , 44 A centralized system could have multiple timing mechanisms running in parallel, allowing modality or spatially specific timing, such as those described in the illusions above. The cerebellum 5 , 6 and basal ganglia 7 have been proposed as candidate brain structures for timing in the millisecond to the hundreds-of-milliseconds ranges, respectively. These brain areas have structural architectures compatible with multiple, parallel timing mechanisms: the regular repeating architecture of the cerebellum and the repeating loops involving the basal ganglia and cortical structures (corticostriatal loops). In a centralized system with plural mechanisms, the timing of any given event could be independent of another: for example, timing of a visual stimulus in a given spatial location compared to one in another.
The cerebellum as a centralized timer
There is evidence indicating that the cerebellum may play a key role in timing within the millisecond to hundreds-of-milliseconds ranges. 5 A recent meta-analysis of multiple neuroimaging studies 45 revealed that the cerebellum, among other areas, is active for both perceptual and motor timing tasks of subsecond duration. This observation is supported by deficits when cerebellar function is impaired. Both patients with lesions in the cerebellum and normal subjects who receive transient magnetic pulses to disrupt ongoing neural activity in the cerebellum exhibit impaired timing behaviors in both motor 46 – 48 and perceptual 47 , 49 , 50 subsecond timing tasks. The consistent activation and necessity of the cerebellum in the timing of subsecond durations across a range of tasks has led to the suggestion that it performs common computations dedicated to temporal processing of these durations. 6 , 8
An example of the specific neural mechanisms that may underlie timing in the cerebellum proposes that time is encoded in a large population of active neurons. 51 A stimulus causes the activity of a large population of extremely abundant neurons, known as granule cells, to change dynamically, owing to negative feedback loops between them and interneurons. In this way, time is encoded in the activity of these neurons, and can be read out by neurons on which granule cells converge, known as Purkinje cells. Purkinje cells will fire when they recognize certain patterns of activity across the neurons converging upon them, and the pattern of activity they recognize is determined by the weights between the Purkinje cell and granule cell synapses. 51 , 52 In this way, time is decoded from the time-varying activity of a large population of neurons.
However, despite being a localized brain area, apparently performing common computations for temporal processing across different tasks, the cerebellum is unlikely to be a dedicated clock. The cerebellum has also been strongly implicated in motor control and associative learning. 53 Dissociating whether timing or other functions are the primary computational purpose of the cerebellum is difficult. Indeed, there is debate in the cerebellum literature as to whether the cerebellar cortex is responsible for storing memory traces of temporal 54 or conditioned–unconditioned stimulus 55 relationships.
The basal ganglia as a centralized timer
Converging evidence suggests that the subcortical structures known as the basal ganglia may play an important role in timing. For example, damage to the striatum—a part of the basal ganglia—in Parkinson's disease is associated with robust and replicable deficits in temporal tasks. 56 Dopamine is one of the key neurotransmitters stimulating the striatum, and its dysregulation similarly causes temporal distortion in this disease, as well as in schizophrenia. 56 Corresponding pharmacological and lesion studies in animals further implicate the striatum and dopamine system in timing. 7 Functional neuroimaging data in humans reveal activity in the basal ganglia and connected cortical areas during timing tasks. 57 , 58 For example, when participants pay attention to the duration versus color of a stimulus, there is an increase in activity in a corticostriatal network. 59 Based on these converging findings, a model of how the basal ganglia and associated neural circuitry may be able to tell time has been proposed.
The striatal beat frequency (SBF) model proposes that duration estimation is based on the detection of coincidental oscillatory processes in corticostriatal circuits. 7 The model suggests that, at the beginning of an event to be timed, dopaminergic output from the ventral tegmental area “resets” and synchronizes corticostriatal oscillatory activity. Neurons in the striatum, onto which cortical neurons converge, then track the oscillatory activity until a reinforcement signal indicates the end of the timed duration. This reinforcement signal, originating from the substantia nigra, releases dopamine into the striatum, which modulates corticostriatal synaptic weights. With experience, the striatal neurons learn to recognize a specific “snapshot” of coincidental oscillatory activity, representing a specific duration. This model predicts, for example, that a peak in neuronal activity in the striatum should be observed around the end of a timed duration—a finding paralleled by ensemble recordings of striatal neurons. 60
Some have proposed the SBF model to be a dedicated timer. 57 However, it could be argued that frontostriatal systems are far from exclusively dedicated to timing functions, as they may instead/also be important in action selection and in sensorimotor decision making. 6
Importantly, the SBF model and the cerebellar timing model outlined above do not require any temporal accumulator. Instead, they rely on coincidence detection—a neurophysiologically more feasible computation. 44 The models bear striking similarities. In both, massive convergence onto a set of neurons (Purkinje cells in the cerebellum, striatal neurons in the basal ganglia) allows these neurons to recognize snapshots of neural activity that evolves over time. Further, in both, reinforcement signals (transmitted by climbing fibers in the cerebellum and dopaminergic nigrostriatal neurons in the basal ganglia) teach Purkinje (cerebellum) and striatal (basal ganglia) neurons to recognize “stamps” of large-scale, time-dependent neural activity by adjusting synaptic weights.
To summarize, it is possible that the basal ganglia and cerebellum serve as centralized clocks, despite the evidence of localized timing mechanisms. However, it is not clear whether these timing mechanisms are dedicated to the problem of timing, or whether timing is a process inextricably wrapped up in other computational analyses being performed: for example, associative learning in the cerebellum or selection in the basal ganglia.
Distributed, intrinsic timing mechanisms
Some scholars have disposed of centralized timing mechanisms altogether in favor of distributed timing mechanisms. In these models, temporal processing is an intrinsic property of information processing and distributed throughout the brain. One may ask, why dispose of a centralized clock? Perhaps, simply because we can: centralized clocks may not be necessary for coding temporal information. Additionally, neuronal activity that covaries with the timing of events has been observed in diverse areas of the brain, suggesting that it may be a ubiquitous aspect of cortical processing. In the proposed distributed timing mechanisms outlined below, time is intrinsically represented: neural networks inherently encode time while processing other information, such as stimulus features or motor commands.
State-dependent networks (SDN)
If there is no centralized clock, how do we process the temporal dimension of events? An intriguing possibility is that a neural network processing a sensory event inherently encodes its temporal component as a result of time-dependent changes in network state. 10 , 61
This SDN model proposes that local neural circuits are inherently capable of processing both temporal and spatial information and that the processing of both is inextricably linked. 61 As a result, temporal information is inherently encoded in evolving neural trajectories—analogous to the spatiotemporal pattern of ripples in a pond 61 (one could determine how long ago the stone was dropped by looking at the trajectory of the ripples). It is noteworthy that the SDN model likely accounts for temporal processing only up to durations of a few hundred miliiseconds. 10
A crucial aspect of the SDN model is that spatiotemporal information is encoded in local neural circuits, 10 and timing is therefore distributed throughout the brain. This type of mechanism would be compatible with the findings discussed above demonstrating spatially localized temporal distortions, 17 – 19 since if time is encoded in local neural networks, then distortions of time in one location can be independent of those in another. The SDN model therefore suggests that temporal processing over short time scales occurs in local sensory or motor cortices, consistent with functional neuroimaging data. 62
Climbing neural activity
Another influential distributed account for how the brain may keep track of time is through climbing neural activity. 63 This model suggests that neural activity increases as a function of time, ramping up until the end of a timed duration. The existence of climbing neural activity has been documented through recordings from individual neurons in numerous brain areas of monkeys. These include the anterior cingulate cortex, premotor cortex, posterior parietal cortex, supplementary motor area (SMA), and pre-SMA (for review, see Ref. 63). Such activity is generally elicited by a stimulus signaling the onset of a fixed temporal interval preceding a response or another task-relevant stimulus.
The ramping up of neural activity until the end of a timed duration is suggestive of such neurons generating an internal representation of duration. 63 , 64 Interestingly, while this may be true, it cannot be determined that such activity is performing an exclusively temporal function, representing duration per se. Since the end of the timed duration is invariably marked by a response or another stimulus, the ramping up of neural activity could represent signals related to motor preparation or expectancy, and not necessarily timing per se.
For example, it has been shown that neurons in a region of the parietal cortex of the monkey, which has been implicated in attention and decision making, increase their firing rate as a function of elapsed time in anticipation of a salient event. In this case, the neuron's firing rate, as well as representing elapsed time, also represents the probability of the event occurring given that it has not already occurred, 65 also known as the hazard function. The neuron, therefore, may represent mounting expectation instead of or in addition to timing of the interval. Similarly, in another experiment, the apparent coding of time by neurons in this parietal region may represent decision variables. Monkeys were trained to judge the duration of a stimulus as longer or shorter than another by making a saccade to targets representing “shorter” or “longer.” 66 Neurons with a receptive field containing the “shorter” target maintained their activity until the duration of the stimulus exceeded that of the stimulus to be judged against, after which activity dropped off. Activity in neurons with the “longer” target in their receptive field increased over time. Therefore, although representing time, these neurons also represent the monkey's choices. These two examples demonstrate how apparent coding of duration can be inextricably tied to other variables.
Human studies have similarly suggested that neural activity apparently representing duration may reflect other processes. For example, a slowly developing negative potential recorded from the scalp of participants using electroencephalography—the contingent negative variation—is present during the timing of intervals. 67 It develops when a response is required at a predictable point in time, thereby requiring temporal estimation. Accordingly, some have interpreted it to reflect a neural representation of the accumulator in the pacemaker–accumulator model. 68 However, critical evaluation of the evidence on which this proposition is based suggests that the contingent negative variation does not index interval timing mechanisms, but rather expectation, response preparation, or decision-making processes that are time dependent. 63 , 69 , 70
Further, Cui et al . 71 asked participants to react as quickly as possible to a cue following a variable waiting period, and investigated neural correlates of this waiting period using functional magnetic resonance imaging (fMRI). It was found that the amplitude of the fMRI signal in the SMA after the cue presentation depended on the wait time, resembling the cumulative hazard rate (i.e., the cumulative probability of cue presentation given it has not already been presented). Importantly, when participants were informed of the cue arrival time, the fMRI signal no longer depended on the wait time, suggesting that the signal does not simply reflect elapsed time, but rather temporal probabilities. The authors suggested that this signal may be produced by the integration of hazard signals from the parietal cortex. 65 Therefore, the apparent representation of duration by climbing neural activity is likely inextricably and intrinsically linked to other variables.
One may wonder whether, in order for neurons to ramp their activity as a linear function of time, they require input from a centralized system. While this is possible, an alternative explanation is that such activity could be intrinsically generated, 9 thereby negating necessity for a centralized system at any stage of processing. Indeed, it has been proposed that self-sustaining, time-varying neural activity can be generated by the internal dynamics of recurrently connected networks. 9 A model based on these principles is, of course, entirely intrinsic in nature, and has recently been applied to account for motor timing. 9
Models of simultaneity and recalibration
In addition to interval timing, neural models have been proposed to account for the temporal recalibration effects. Cai et al . 72 have developed a model for recalibration of temporal order between motor and sensory events. In their model, different neuronal populations encode different motor–sensory delays, and the output of these neurons reaches opponent neuronal populations encoding the sensory event as “before” or “after” the motor event. The difference in activity between these “before” and “after” populations determines the perceptual judgment. The input weights to the “before”/“after” neuronal populations are scaled by adaptation to temporal delays. For example, if repeatedly exposed to a delay between motor actions and their consequences, the input weights of the “after” population will decrease, while those of the “before” population will increase. Therefore, the system becomes biased toward making “before” decisions. This would lead to a shortening of the perceived time between action and effect, as observed in the motor–sensory recalibration experiments described above. If the delay is then suddenly removed, the participant will still be biased to making a “before” decision, leading to the illusory reversal of action and sensation, as is observed experimentally. 23 An analogous model has been proposed for sensory–sensory audiovisual recalibration. 73
It is noteworthy that, in these models, temporal recalibrations do not result from changes in signal transmission or processing times, which have been proposed as alternative mechanisms of recalibration. 74 – 76 Rather, the temporal properties of events themselves are represented. In this way, temporal perception is the brain's interpretation of external events, and not simply the order in which events occur in time, as our intuition would suggest (which is why we find the illusions so striking). For example, audiovisual recalibration is attributable to adjusting the weights of neurons tuned to different delays onto output populations, as opposed to altering the signal transmission or processing time of auditory or visual signals. This moves away from the time-encodes-time notion, whereby the “external” timing of events encodes the perceptual timing by the relative signal arrival times. Consistent with the hypothesis that temporal perception is not a simple reflection of simultaneity of neural signals, but rather the brain's interpretation of external events, Nishida and Johnston 77 have proposed “time markers,” which refers to the representation of temporal patterns; it is the relationship between these representations that gives rise to the perception of the relative timing of events.
These ideas echo those of Dennett and Kinsbourne, 78 who draw the distinction between the representation of temporal properties and the temporal properties of representations. According to their scheme, for the brain to represent “A before B,” a representing of A before a representing of B is not required, 78 as is observed in the recalibration experiments. The authors point out that this accounts for the well-known “rabbit illusion”—whereby a rapid sequence of taps delivered near the wrist and then the elbow creates the illusory sensation of sequential taps hopping up the arm toward the elbow, before the elbow stimulation is perceived. That time does not necessarily encode time allows the “backdating” in time required for the perception of taps between the wrist and elbow before the perception of taps at the elbow.
However, there appears to be an upper bound on the durations susceptible to temporal compression or calibration. 20 , 23 This may reflect a maximum separation before two events are no longer labeled as causally related. Alternatively, it could reflect a temporal window in which the temporal realignments could still be useful for the brain to control action. 78 Once the perceptual processes within the observer have begun to perform their function (e.g., initiating action), there is no point in altering the representations.
Beyond the “interval” time scale, the story may become very different. Longer durations—above the hundreds-of-milliseconds range probed in the above experiments—do appear to follow the arrow of time more linearly: you know you read this paragraph after the previous, and, so far, we have discovered no illusion that could shift this.
Other considerations
Is timing ever truly dedicated.
A deep issue regarding timing in the brain is whether neural processing can ever be said to be dedicated to the problem of timing, or whether temporal processing is always, by necessity, wrapped up with the coding of other attributes in the brain. As discussed above, even in apparently dedicated models such as in cerebellar timing and the SBF model, timing may actually be a by-product of coding other processes, such as associating stimuli to actions or selecting motor commands.
Other models, such as those directly coding temporal delay to determine the order of events, may appear to be dedicated. However, it is interesting to speculate whether such models really necessitate a pure representation of time. Could time-related activity in these neurons coding “before”/“after” also be inextricable from the coding of other, task-relevant properties? For example, we have indicated that climbing neural activity could also reflect decision variables rather than interval timings. Analogously, neural activity in these models could encode the decision variable “before” or “after” as part of the choice-selection process. In other words, neural activity linked to timing could reflect a process of evidence accumulation for action selection, and not the extracted representation of time per se.
The question remains whether the coding of temporal processing in the brain can ever truly be separated from its context. What purpose could it have, since perhaps only timing in the context of something else could bear on our perception and action toward external events, as well as on the memories and musings of our internal mental life? Indeed, it is difficult to conceive of a completely abstracted and disembodied neural representation of time, although this on its own may be insufficient ground for denying its possible existence.
Time and space
At multiple levels of analysis, parallels can be drawn between temporal and spatial processing. For example, in addition to the saccade-induced temporal distortions discussed above, saccades also distort spatial perception, 79 with dynamics that are tightly coupled to the temporal distortions. 80 Accordingly, models such as the SDN model explicitly acknowledge and propose the inextricable bundling of temporal and spatial information. 61
The model by Cai et al . 72 using delay lines to account for temporal recalibration also proposes the overlap of temporal and spatial processing. Recalibration of temporal order judgments is proposed to be a temporal analogue to the motion after effect, with identical neural mechanisms underlying judgments of both time and space. 72 In other words, the neural computations performed are the same, suggesting an evolutionarily conservative genetic program coding for a module capable of dealing with either time or space depending only on the inputs. 72 Further, as discussed above, there are apparent similarities in the way the brain uses motor interactions with the world and the feedback received from them to calibrate temporal and spatial percepts. Finally, parallels are increasingly proposed between “place” and “time” cells identified in the rodent hippocampus, which are thought to contribute to the spatial and temporal integration and organization of events in memory. 81 – 83
An interesting question that can be raised about space, given the debates about timing, is whether neural mechanisms are ever dedicated to spatial processing. Or do similar arguments against dedicated timing hold for space? For example, place cells, which code the location of an animal in space, 84 may not code the abstract representation of space per se, but rather the structured relation among things in space. This is supported by the fact that spatial coding in place cells is highly context dependent: cells code different locations in different environments, and their mapping changes when the environment changes. 85 Furthermore, spatial properties of neurons in parietal and premotor regions within sensorimotor circuits are often linked to locations relative to motor effectors, as opposed to absolute space. 86 , 87
Taken together, it is interesting to speculate whether there is a commonality in the way that the brain deals with temporal and spatial information to create a meaningful and coherent perception of the world around us. The similarities in the way that the brain deals with these dimensions raise the possibility that the same neural architectures could process analogous aspects of both. Furthermore, if time and space are not coded in isolation in the brain, but instead can only be explained as the relation between things, could this contribute to philosophical debates about the reality and nature of these dimensions? Kant, as mentioned above, questions whether both time and space are constructs of the mind, neither of which may have a real external existence—a stance somewhat echoed by Einstein, who states: “Time and space are modes by which we think and not conditions in which we live.” One suggestion is that they are both constructs that help organize our perception of events, so that we can better understand our world and the relations of events within it.
Experimental psychology and neuroscience have, like physics, challenged some of our intuitive notions of a constant and linear passage of time, and have begun to elucidate some of the mechanisms by which the brain may represent time. At least over a short time frame, our temporal perception of events is far from veridical, and multiple timelines are capable of dynamic recalibration. This is incompatible with the notion of a unitary centralized and dedicated clock, from which all timing is performed. Alternative accounts for multiple centralized clocks and distributed timing mechanisms have been proposed. These models are not mutually exclusive, and timing may also be achieved through a combination of centralized and distributed processing. 42 , 43 Regardless of the exact timing structures and mechanisms, the fundamental question remains whether neural processing is ever exclusively dedicated to the problem of timing. Neural activity that may appear as such can always be reframed to be coding some other process that occurs in time, rather than time itself.
Acknowledgments
This work was supported by a Wellcome Trust Senior Investigator Award to ACN (104571/Z/14/Z) and by the National Institute for Health Research (NIHR) Oxford Biomedical Research Centre based at Oxford University Hospitals Trust, Oxford University.
Conflicts of interest
The authors declare no conflicts of interest.
- Newton I. Mathematical Principles of Natural Philosophy. Berkeley, California: University of California Press; 1999. [ Google Scholar ]
- Alexander H. The Leibniz-Clarke Correspondence. Manchester, United Kingdom: Manchester University Press; 1956. [ Google Scholar ]
- Parsons C. Cambridge Companion to Kant. Cambridge, United Kingdom: Cambridge University Press; 1992. The Transcendental Aesthetic; pp. 62–100. [ Google Scholar ]
- Forsee A. Albert Einstein: Theoretical Physicist. New York: Macmillan; 1963. [ Google Scholar ]
- Ivry RB. The representation of temporal information in perception and motor control. Curr. Opin. Neurobiol. 1996;6:851–857. doi: 10.1016/s0959-4388(96)80037-7. [ DOI ] [ PubMed ] [ Google Scholar ]
- Ivry RB. Spencer RMC. The neural representation of time. Curr. Opin. Neurobiol. 2004;14:225–232. doi: 10.1016/j.conb.2004.03.013. [ DOI ] [ PubMed ] [ Google Scholar ]
- Buhusi CV. Meck WH. What makes us tick? Functional and neural mechanisms of interval timing. Nat. Rev. Neurosci. 2005;6:755–765. doi: 10.1038/nrn1764. [ DOI ] [ PubMed ] [ Google Scholar ]
- Ivry RB. Schlerf JE. Dedicated and intrinsic models of time perception. Trends Cogn. Sci. 2008;12:273–280. doi: 10.1016/j.tics.2008.04.002. [ DOI ] [ PMC free article ] [ PubMed ] [ Google Scholar ]
- Buonomano D. Laje R. Population clocks: motor timing with neural dynamics. Trends Cogn. Sci. 2010;14:520–527. doi: 10.1016/j.tics.2010.09.002. [ DOI ] [ PMC free article ] [ PubMed ] [ Google Scholar ]
- Karmarkar UR. Buonomano DV. Timing in the absence of clocks: encoding time in neural network states. Neuron. 2007;53:427–438. doi: 10.1016/j.neuron.2007.01.006. [ DOI ] [ PMC free article ] [ PubMed ] [ Google Scholar ]
- Darlington TK. Closing the circadian loop: CLOCK-induced transcription of its own inhibitors per and tim. Science. 1998;280:1599–1603. doi: 10.1126/science.280.5369.1599. [ DOI ] [ PubMed ] [ Google Scholar ]
- Meegan D, Aslin R. Jacobs R. Motor timing learned without motor training. Nat. Neurosci. 2000;3:860–862. doi: 10.1038/78757. [ DOI ] [ PubMed ] [ Google Scholar ]
- Warm JS, Stutz RM. Vassolo PA. Intermodal transfer in temporal discrimination. Percept. Psychophys. 1975;18:281–286. [ Google Scholar ]
- Ivry RB. Hazeltine RE. Perception and production of temporal intervals across a range of durations: evidence for a common timing mechanism. J. Exp. Psychol. Hum. Percept. Perform. 1995;21:3–18. doi: 10.1037//0096-1523.21.1.3. [ DOI ] [ PubMed ] [ Google Scholar ]
- Keele SW, Jennings P, Jones S, et al. On the modularity of sequence representation. J. Mot. Behav. 1995;27:17–30. [ Google Scholar ]
- Morrone MC, Ross J. Burr D. Saccadic eye movements cause compression of time as well as space. Nat. Neurosci. 2005;8:950–954. doi: 10.1038/nn1488. [ DOI ] [ PubMed ] [ Google Scholar ]
- Johnston A, Arnold DH. Nishida S. Spatially localized distortions of event time. Curr. Biol. 2006;16:472–479. doi: 10.1016/j.cub.2006.01.032. [ DOI ] [ PubMed ] [ Google Scholar ]
- Burr D, Tozzi A. Morrone MC. Neural mechanisms for timing visual events are spatially selective in real-world coordinates. Nat. Neurosci. 2007;10:423–425. doi: 10.1038/nn1874. [ DOI ] [ PubMed ] [ Google Scholar ]
- Cicchini G. Morrone M. Shifts in spatial attention affect the perceived duration of events. J. Vis. 2009;9:1–13. doi: 10.1167/9.1.9. [ DOI ] [ PubMed ] [ Google Scholar ]
- Haggard P, Clark S. Kalogeras J. Voluntary action and conscious awareness. Nat. Neurosci. 2002;5:382–385. doi: 10.1038/nn827. [ DOI ] [ PubMed ] [ Google Scholar ]
- Buehner MJ. Humphreys GR. Causal binding of actions to their effects. Psychol. Sci. 2009;20:1221–1228. doi: 10.1111/j.1467-9280.2009.02435.x. [ DOI ] [ PubMed ] [ Google Scholar ]
- Buehner MJ. Understanding the past, predicting the future: causation, not intentional action, is the root of temporal binding. Psychol. Sci. 2012;23:1490–1497. doi: 10.1177/0956797612444612. [ DOI ] [ PubMed ] [ Google Scholar ]
- Stetson C, Cui X, Montague PR. Eagleman DM. Motor-sensory recalibration leads to an illusory reversal of action and sensation. Neuron. 2006;51:651–659. doi: 10.1016/j.neuron.2006.08.006. [ DOI ] [ PubMed ] [ Google Scholar ]
- Calvert G, Spence C. Stein B. The Handbook of Multisensory Processes. Cambridge, Massachusetts: MIT Press; 2004. [ Google Scholar ]
- Fujisaki W, Shimojo S, Kashino M. Nishida S. Recalibration of audiovisual simultaneity. Nat. Neurosci. 2004;7:773–778. doi: 10.1038/nn1268. [ DOI ] [ PubMed ] [ Google Scholar ]
- Vatakis A, Navarra J, Soto-Faraco S. Spence C. Temporal recalibration during asynchronous audiovisual speech perception. Exp. Brain Res. 2007;181:173–181. doi: 10.1007/s00221-007-0918-z. [ DOI ] [ PubMed ] [ Google Scholar ]
- Haering C. Kiesel A. Mine is earlier than yours: causal beliefs influence the perceived time of action effects. Front. Psychol. 2012;3:393. doi: 10.3389/fpsyg.2012.00393. [ DOI ] [ PMC free article ] [ PubMed ] [ Google Scholar ]
- Parsons BD, Novich SD. Eagleman DM. Motor-sensory recalibration modulates perceived simultaneity of cross-modal events at different distances. Front. Psychol. 2013;4:46. doi: 10.3389/fpsyg.2013.00046. [ DOI ] [ PMC free article ] [ PubMed ] [ Google Scholar ]
- Zampini M, Guest S, Shore DI. Spence C. Audio-visual simultaneity judgments. Percept. Psychophys. 2005;67:531–544. doi: 10.3758/BF03193329. [ DOI ] [ PubMed ] [ Google Scholar ]
- Van Wassenhove V. Grant KW. Poeppel D. Temporal window of integration in auditory-visual speech perception. Neuropsychologia. 2007;45:598–607. doi: 10.1016/j.neuropsychologia.2006.01.001. [ DOI ] [ PubMed ] [ Google Scholar ]
- Stevenson RA, Fister JK, Barnett ZP, Nidiffer AR. Wallace MT. Interactions between the spatial and temporal stimulus factors that influence multisensory integration in human performance. Exp. Brain Res. 2012;219:121–137. doi: 10.1007/s00221-012-3072-1. [ DOI ] [ PMC free article ] [ PubMed ] [ Google Scholar ]
- Hume D. Enquiry Concerning Human Understanding. New York: PF Collier & Son; 1748. [ Google Scholar ]
- Eagleman DM. Holcombe AO. Causality and the perception of time. Trends Cogn. Sci. 2002;6:323–325. doi: 10.1016/s1364-6613(02)01945-9. [ DOI ] [ PubMed ] [ Google Scholar ]
- Miyazaki M, Yamamoto S, Uchida S. Kitazawa S. Bayesian calibration of simultaneity in tactile temporal order judgment. Nat. Neurosci. 2006;9:875–877. doi: 10.1038/nn1712. [ DOI ] [ PubMed ] [ Google Scholar ]
- Yamamoto S, Miyazaki M, Iwano T. Kitazawa S. Bayesian calibration of simultaneity in audiovisual temporal order judgments. PLoS One. 2012;7:e40379. doi: 10.1371/journal.pone.0040379. [ DOI ] [ PMC free article ] [ PubMed ] [ Google Scholar ]
- Welch R. Perceptual Modification: Adapting to Altered Sensory Environments. New York: Academic Press; 1978. [ Google Scholar ]
- Redding G. Wallace B. Strategic calibration and spatial alignment: a model from prism adaptation. J. Mot. Behav. 2002;34:126–138. doi: 10.1080/00222890209601935. [ DOI ] [ PubMed ] [ Google Scholar ]
- Treisman M. Temporal discrimination and the indifference interval: implications for a model of the “internal clock.”. Psychol. Monogr. Gen. Appl. 1963;77:1–31. doi: 10.1037/h0093864. [ DOI ] [ PubMed ] [ Google Scholar ]
- Hicks RE, Miller GW. Kinsbourne M. Prospective and retrospective judgments of time as a function of amount of information processed. Am. J. Psychol. 1976;89:719–730. [ PubMed ] [ Google Scholar ]
- Macar F, Grondin S. Casini L. Controlled attention sharing influences time estimation. Mem. Cogn. 1994;22:673–686. doi: 10.3758/bf03209252. [ DOI ] [ PubMed ] [ Google Scholar ]
- Brown SW. Attentional resources in timing: interference effects in concurrent temporal and nontemporal working memory tasks. Percept. Psychophys. 1997;59:1118–1140. doi: 10.3758/bf03205526. [ DOI ] [ PubMed ] [ Google Scholar ]
- Merchant H, Harrington DL. Meck WH. Neural basis of the perception and estimation of time. Annu. Rev. Neurosci. 2013;36:313–336. doi: 10.1146/annurev-neuro-062012-170349. [ DOI ] [ PubMed ] [ Google Scholar ]
- Allman MJ, Teki S, Griffiths TD. Meck WH. Properties of the internal clock: first-and second-order principles of subjective time. Ann. Rev. Psychol. 2014;65:743–771. doi: 10.1146/annurev-psych-010213-115117. [ DOI ] [ PubMed ] [ Google Scholar ]
- Wiener M, Turkeltaub P. Coslett HB. The image of time: a voxel-wise meta-analysis. Neuroimage. 2010;49:1728–1740. doi: 10.1016/j.neuroimage.2009.09.064. [ DOI ] [ PubMed ] [ Google Scholar ]
- Bhattacharjee Y. A timely debate about the brain. Science. 2006;311:596–598. doi: 10.1126/science.311.5761.596. [ DOI ] [ PubMed ] [ Google Scholar ]
- Perrett S, Ruiz B. Mauk M. Cerebellar cortex lesions disrupt learning-dependent timing of conditioned eyelid responses. J. Neurosci. 1993;13:1708–1718. doi: 10.1523/JNEUROSCI.13-04-01708.1993. [ DOI ] [ PMC free article ] [ PubMed ] [ Google Scholar ]
- Ivry R. Keele S. Timing functions of the cerebellum. J. Cogn. Neurosci. 1989;1:136–152. doi: 10.1162/jocn.1989.1.2.136. [ DOI ] [ PubMed ] [ Google Scholar ]
- Koch G, Oliveri M. Torriero S. Repetitive TMS of cerebellum interferes with millisecond time processing. Exp. Brain Res. 2007;179:291–299. doi: 10.1007/s00221-006-0791-1. [ DOI ] [ PubMed ] [ Google Scholar ]
- Mangels J, Ivry R. Shimizu N. Dissociable contributions of the prefrontal and neocerebellar cortex to time perception. Cogn. Brain Res. 1998;7:15–39. doi: 10.1016/s0926-6410(98)00005-6. [ DOI ] [ PubMed ] [ Google Scholar ]
- Lee K, Egleston P. Brown W. The role of the cerebellum in subsecond time perception: evidence from repetitive transcranial magnetic stimulation. J. Cogn. Neurosci. 2007;19:147–157. doi: 10.1162/jocn.2007.19.1.147. [ DOI ] [ PubMed ] [ Google Scholar ]
- Buonomano D. Karmarkar U. Book review: how do we tell time? Neuroscience. 2002;8:42–51. doi: 10.1177/107385840200800109. [ DOI ] [ PubMed ] [ Google Scholar ]
- Buonomano D. Mauk M. Neural network model of the cerebellum: temporal discrimination and the timing of motor responses. Neural Comput. 1994;6:38–55. [ Google Scholar ]
- McCormick DA. Thompson RF. Cerebellum: essential involvement in the classically conditioned eyelid response. Science. 1984;223:296–299. doi: 10.1126/science.6701513. [ DOI ] [ PubMed ] [ Google Scholar ]
- Koekkoek S, et al. Cerebellar LTD and learning-dependent timing of conditioned eyelid responses. Science. 2003;301:1736–1739. doi: 10.1126/science.1088383. [ DOI ] [ PubMed ] [ Google Scholar ]
- Kellett D, Fukunaga I, Chen-Kubota E, Dean P. Yeo C. Memory consolidation in the cerebellar cortex. PLoS One. 2010;5:e11737. doi: 10.1371/journal.pone.0011737. [ DOI ] [ PMC free article ] [ PubMed ] [ Google Scholar ]
- Allman MJ. Meck WH. Pathophysiological distortions in time perception and timed performance. Brain. 2012;135:656–677. doi: 10.1093/brain/awr210. [ DOI ] [ PMC free article ] [ PubMed ] [ Google Scholar ]
- Coull JT, Cheng RK. Meck WH. Neuroanatomical and neurochemical substrates of timing. Neuropsychopharmacology. 2011;36:3–25. doi: 10.1038/npp.2010.113. [ DOI ] [ PMC free article ] [ PubMed ] [ Google Scholar ]
- Coull J. Nobre A. Dissociating explicit timing from temporal expectation with fMRI. Curr. Opin. Neurobiol. 2008;18:137–144. doi: 10.1016/j.conb.2008.07.011. [ DOI ] [ PubMed ] [ Google Scholar ]
- Coull JT, Vidal F, Nazarian B. Macar F. Functional anatomy of the attentional modulation of time estimation. Science. 2004;303:1506–1508. doi: 10.1126/science.1091573. [ DOI ] [ PubMed ] [ Google Scholar ]
- Matell M, Meck W. Nicolelis M. Interval timing and the encoding of signal duration by ensembles of cortical and striatal neurons. Behav. Neurosci. 2003;117:760–773. doi: 10.1037/0735-7044.117.4.760. [ DOI ] [ PubMed ] [ Google Scholar ]
- Buonomano D. Maass W. State-dependent computations: spatiotemporal processing in cortical networks. Nat. Rev. Neurosci. 2009;10:113–125. doi: 10.1038/nrn2558. [ DOI ] [ PubMed ] [ Google Scholar ]
- Lewis PA. Miall RC. Distinct systems for automatic and cognitively controlled time measurement: evidence from neuroimaging. Curr. Opin. Neurobiol. 2003;13:250–255. doi: 10.1016/s0959-4388(03)00036-9. [ DOI ] [ PubMed ] [ Google Scholar ]
- Wittmann M. The inner sense of time: how the brain creates a sense of duration. Nat. Rev. Neurosci. 2013;14:217–223. doi: 10.1038/nrn3452. [ DOI ] [ PubMed ] [ Google Scholar ]
- Mita A, Mushiake H, Shima K, et al. Interval time coding by neurons in the presupplementary and supplementary motor areas. Nat. Neurosci. 2009;12:502–507. doi: 10.1038/nn.2272. [ DOI ] [ PubMed ] [ Google Scholar ]
- Janssen P. Shadlen MN. A representation of the hazard rate of elapsed time in macaque area LIP. Nat. Neurosci. 2005;8:234–241. doi: 10.1038/nn1386. [ DOI ] [ PubMed ] [ Google Scholar ]
- Leon MI. Shadlen MN. Representation of time by neurons in the posterior parietal cortex of the macaque. Neuron. 2003;38:317–327. doi: 10.1016/s0896-6273(03)00185-5. [ DOI ] [ PubMed ] [ Google Scholar ]
- Pfeuty M, Ragot R. Pouthas V. Relationship between CNV and timing of an upcoming event. Neurosci. Lett. 2005;382:106–111. doi: 10.1016/j.neulet.2005.02.067. [ DOI ] [ PubMed ] [ Google Scholar ]
- Macar F. Vidal F. Timing processes: an outline of behavioural and neural indices not systematically considered in timing models. Can. J. Exp. Psychol. 2009;63:227–239. doi: 10.1037/a0014457. [ DOI ] [ PubMed ] [ Google Scholar ]
- Van Rijn H. Kononowicz TW. Meck WH. Contingent negative variation and its relation to time estimation: a theoretical evaluation. Front. Integr. Neurosci. 2011;5:91. doi: 10.3389/fnint.2011.00091. [ DOI ] [ PMC free article ] [ PubMed ] [ Google Scholar ]
- Miniussi C, Wilding EL, Coull JT. Nobre AC. Orienting attention in time. Modulation of brain potentials. Brain. 1999;122:1507–1518. doi: 10.1093/brain/122.8.1507. [ DOI ] [ PubMed ] [ Google Scholar ]
- Cui X, Stetson C, Montague PR. Eagleman DM. Ready…go: amplitude of the FMRI signal encodes expectation of cue arrival time. PLoS Biol. 2009;7:e1000167. doi: 10.1371/journal.pbio.1000167. [ DOI ] [ PMC free article ] [ PubMed ] [ Google Scholar ]
- Cai M, Stetson C. Eagleman DM. A neural model for temporal order judgments and their active recalibration: a common mechanism for space and time? Front. Psychol. 2012;3:470. doi: 10.3389/fpsyg.2012.00470. [ DOI ] [ PMC free article ] [ PubMed ] [ Google Scholar ]
- Roach NW, Heron J, Whitaker D. McGraw PV. Asynchrony adaptation reveals neural population code for audio-visual timing. Proc. Biol. Sci. 2011;278:1314–1322. doi: 10.1098/rspb.2010.1737. [ DOI ] [ PMC free article ] [ PubMed ] [ Google Scholar ]
- Kösem A, Gramfort A. van Wassenhove V. Encoding of event timing in the phase of neural oscillations. NeuroImage. 2014;92:274–284. doi: 10.1016/j.neuroimage.2014.02.010. [ DOI ] [ PubMed ] [ Google Scholar ]
- Navarra J. Adaptation to audiovisual asynchrony modulates the speeded detection of sound. Proc. Natl. Acad. Sci. U. S. A. 2009;106:9169–9173. doi: 10.1073/pnas.0810486106. [ DOI ] [ PMC free article ] [ PubMed ] [ Google Scholar ]
- Di Luca M, Machulla TK. Ernst MO. Recalibration of multisensory simultaneity: cross-modal transfer coincides with a change in perceptual latency. J. Vis. 2009;9:7. doi: 10.1167/9.12.7. [ DOI ] [ PubMed ] [ Google Scholar ]
- Nishida S. Johnston A. Marker correspondence, not processing latency, determines temporal binding of visual attributes. Curr. Biol. 2002;12:359–368. doi: 10.1016/s0960-9822(02)00698-x. [ DOI ] [ PubMed ] [ Google Scholar ]
- Dennett D. Kinsbourne M. Time and the observer: the where and when of consciousness in the brain. Behav. Brain Sci. 1992;15:183–247. [ Google Scholar ]
- Ross J, Morrone MC. Burr DC. Compression of visual space before saccades. Nature. 1997;386:598–601. doi: 10.1038/386598a0. [ DOI ] [ PubMed ] [ Google Scholar ]
- Binda P, Cicchini GM, Burr DC. Morrone MC. Spatiotemporal distortions of visual perception at the time of saccades. J. Neurosci. 2009;29:13147–13157. doi: 10.1523/JNEUROSCI.3723-09.2009. [ DOI ] [ PMC free article ] [ PubMed ] [ Google Scholar ]
- MacDonald CJ, Lepage KQ, Eden UT. Eichenbaum H. Hippocampal “time cells” bridge the gap in memory for discontiguous events. Neuron. 2011;71:737–749. doi: 10.1016/j.neuron.2011.07.012. [ DOI ] [ PMC free article ] [ PubMed ] [ Google Scholar ]
- MacDonald CJ, Carrow S, Place R. Eichenbaum H. Distinct hippocampal time cell sequences represent odor memories in immobilized rats. J. Neurosci. 2013;33:14607–14616. doi: 10.1523/JNEUROSCI.1537-13.2013. [ DOI ] [ PMC free article ] [ PubMed ] [ Google Scholar ]
- Kraus BJ, Robinson II RJ, White JA, Eichenbaum H. Hasselmo ME. Hippocampal “time cells”: time versus path integration. Neuron. 2013;78:1090–1101. doi: 10.1016/j.neuron.2013.04.015. [ DOI ] [ PMC free article ] [ PubMed ] [ Google Scholar ]
- O'Keefe J. Dostrovsky J. The hippocampus as a spatial map. Preliminary evidence from unit activity in the freely-moving rat. Brain Res. 1971;34:171–175. doi: 10.1016/0006-8993(71)90358-1. [ DOI ] [ PubMed ] [ Google Scholar ]
- Moser E, Kropff E. Moser M. Place cells, grid cells, and the brain's spatial representation system. Annu. Rev. Neurosci. 2008;31:69–89. doi: 10.1146/annurev.neuro.31.061307.090723. [ DOI ] [ PubMed ] [ Google Scholar ]
- Colby CL. Goldberg ME. Space and attention in parietal cortex. Ann. Rev. Neurosci. 1999;22:319–349. doi: 10.1146/annurev.neuro.22.1.319. [ DOI ] [ PubMed ] [ Google Scholar ]
- Mattingley J, Husain M, Rorden C, Kennard C. Driver J. Motor role of human inferior parietal lobe revealed in unilateral neglect patients. Nature. 1998;392:179–182. doi: 10.1038/32413. [ DOI ] [ PubMed ] [ Google Scholar ]
- View on publisher site
- PDF (154.7 KB)
- Collections
Similar articles
Cited by other articles, links to ncbi databases.
- Download .nbib .nbib
- Format: AMA APA MLA NLM
Add to Collections
Thank you for visiting nature.com. You are using a browser version with limited support for CSS. To obtain the best experience, we recommend you use a more up to date browser (or turn off compatibility mode in Internet Explorer). In the meantime, to ensure continued support, we are displaying the site without styles and JavaScript.
- View all journals
- My Account Login
- Explore content
- About the journal
- Publish with us
- Sign up for alerts
- Open access
- Published: 29 May 2024
Malleability and fluidity of time perception
- Hirohito M. Kondo ORCID: orcid.org/0000-0002-7444-4996 1 ,
- Elena Gheorghiu 2 &
- Ana P. Pinheiro ORCID: orcid.org/0000-0002-7981-3682 3
Scientific Reports volume 14 , Article number: 12244 ( 2024 ) Cite this article
3890 Accesses
15 Altmetric
Metrics details
- Human behaviour
Time perception is inherently subjective and malleable. We experience a wide range of time scales, from less than a second to decades. In addition, our perception of time can be affected by our attentional and emotional states. Previous psychological and neuroimaging studies have used several paradigms and methods to probe factors that influence time perception. Considering these factors facilitates approaches to improve time management and to enhance sensory experiences. This Collection of time perception studies includes reports that focus on stimulus property, physiological state, cross-modal interaction, attention, learning, age, and environment. These findings help to illuminate the complex mechanisms of time perception.
Humans have no absolute sense of time. Time perception is fundamentally subjective and depends on one's experiences and circumstances. Moments of excitement and joy can seem dizzyingly faster, whereas moments of boredom and stress can feel interminable 1 , illustrating how attentional and emotional states affect time perception. Also, time perception has critical effects on many cognitive abilities and motor skills. For instance, we can play the piano with quick movements. Accurate temporal and rhythmic performance are important not only for playing music, but also for multisensory perception, language, and motor planning 2 , 3 . In addition, we may vividly recall memories from years ago. We have a broad range of time scales 4 . These timings appear to be underpinned by different neural mechanisms 5 . The brain navigates and processes time ranges from subsecond to year, highlighting its remarkable adaptability and complexity.
The more often we pay attention to the passage of time, the longer we perceive time to be 6 . Our perception of the passage of time may vary as a function of age and education 7 or mood state 8 . This is probably consistent with the contextual-change hypothesis that the perceived duration of an event is affected by the number of contextual changes 9 . Extending this idea may explain how different age groups perceive time differently. For boys and girls, holiday adventures are hard to come by. Adults have many routine activities and time seems to pass at an accelerated pace. Relative to adults, children may use heuristic methods for duration estimation 10 . However, it should be noted that feeling the passage of time and estimating duration may employ different mechanisms of time perception 11 .
A simple explanation for the perceived compression and expansion of time is the event-density hypothesis. This postulates that the number of events occurring during a certain period affects perception of time intervals 12 , assuming that the “internal clock” counts at a constant rhythm 13 . Directing attention to salient stimuli or engaging in complex tasks increases internal pulses, i.e., the density of events, resulting in the perception that time is passing quickly 6 . This hypothesis is consistent with the idea that cellular metabolism and the internal clock are intimately interconnected. An early study argued that as body temperature increases, the internal clock seems to advance faster, leading to the perception of shorter durations 14 . Cognitive components, such as working memory and attention, were incorporated into the pulse-generating pacemaker and developed into the scalar expectancy model 15 and the attentional-gate model 16 .
Time perception depends not only on endogenous factors, such as attentional, motivational, and physiological levels 17 , 18 , but also on exogenous factors, such as speed of motion, stimulus complexity 19 , salience of visual stimulus features 20 , and spatial, temporal, social context 21 or environment 22 . Previous studies have frequently employed experimental paradigms such as temporal order or duration judgements to assess time perception of short intervals. In such paradigms, a novel or “oddball” stimulus is perceived as longer in duration than repeated or “standard” stimuli 23 . The first visual stimulus in a train appears to be perceived as longer than successive stimuli 24 . However, such a phenomenon does not occur in relation to auditory stimuli. There is a consensus that timing of subsecond intervals is supported by distributed sensory-specific mechanisms 25 , 26 . An event-related potential (ERP) study demonstrated that people with normal hearing, but not deaf individuals, show a strong ERP response to visual stimuli in temporal areas during a time-bisection task, whereas the same response is not elicited during a space-bisection task 27 (see also a study of developmental viewpoint 28 ). Gamma-aminobutyric acid (GABA) levels in the human visual cortex measured using magnetic resonance spectroscopy appear to correlate with perceived durations of visual intervals, suggesting that the GABAergic system contributes to individual differences in time perception 29 . However, time perception studies in this Collection have found that learning of temporal interval discrimination transfers between auditory and visual modalities 30 and that cross-modal correspondence between auditory pitch and visual elevation selectively affects temporal recalibration 31 .
There is no single sensory organ responsible for time perception. Different brain regions are involved in temporal processing depending on time scales. Subsecond time intervals are mainly processed in the cerebellum 32 , whereas temporal processing in the range of seconds and minutes is supported by the prefrontal cortex and striatum 33 , 34 . In addition, time perception is impaired in disorders of the precuneus/posterior cingulate gyrus 35 and supramarginal gyrus 36 . In particular, the precuneus may contribute to our sense of “presentness”, providing the “now” in the passage of time 37 , 38 .
The advent of digital technology has had an unprecedented impact on time perception. Ubiquitous access to the Internet facilitates instantaneous information retrieval and synchronous communication. A consequence of this persistent connectivity is the potential for information overload, such that the sense of time tends to become ambiguous. The widespread prevalence of social media notifications may contribute to the perceived acceleration of time. However, through flow and meditation states, alternative perceptions of time can be experienced. Specifically, a flow experience is a symbolic phenomenon of time distortion, in which one forgets the passage of time by immersing oneself in a certain activity. People in a flow state often report this state as being “in the zone” 39 . Although there are anecdotal reports of flow experiences by athletes, few studies have captured quantitative aspects of flow states 40 . However, some studies have identified flow states in terms of attentional fluctuations 41 , 42 . Using such methods, it may be possible to overcome methodological difficulties and to measure altered time perception.
Articles in this Collection show that the interplay of stimulus property, physiological state, attention, age, and environment fundamentally shapes individual temporal experiences. A deep understanding of these factors is undoubtedly crucial to the ongoing field of time perception research.
James, W. The Principles of Psychology (Henry Holt and Company, 1890).
Google Scholar
Kaya, E. & Henry, M. J. Reliable estimation of internal oscillator properties from a novel, fast-paced tapping paradigm. Sci. Rep. 12 , 20466. https://doi.org/10.1038/s41598-022-24453-6 (2022).
Article ADS CAS PubMed PubMed Central Google Scholar
Li, L., Yotsumoto, Y. & Hayashi, M. J. Temporal perceptual learning distinguishes between empty and filled intervals. Sci. Rep. 12 , 9824. https://doi.org/10.1038/s41598-022-13814-w (2022).
Eagleman, D. M. Human time perception and its illusions. Curr. Opin. Neurobiol. 18 , 131–136. https://doi.org/10.1016/j.conb.2008.06.002 (2008).
Article CAS PubMed PubMed Central Google Scholar
Wittmann, M. & Paulus, M. P. Decision making, impulsivity and time perception. Trends Cogn. Sci. 12 , 7–12. https://doi.org/10.1016/j.tics.2007.10.004 (2008).
Article PubMed Google Scholar
Martinelli, N. & Droit-Volet, S. What factors underlie our experience of the passage of time? Theoretical consequences. Psychol. Res. 86 , 522–530. https://doi.org/10.1007/s00426-021-01486-6 (2022).
Wittmann, M. & Lehnhoff, S. Age effects in perception of time. Psychol. Rep. 97 , 921–935. https://doi.org/10.2466/pr0.97.3.921-935 (2005).
Buzi, G., Eustache, F., D’Argembeau, A. & Hinault, T. The role of depressive symptoms in the interplay between aging and temporal processing. Sci. Rep. 13 , 11375. https://doi.org/10.1038/s41598-023-38500-3 (2023).
Block, R. A. & Reed, M. A. Remembered duration: Evidence for a contextual-change hypothesis. J. Exp. Psychol. Hum. Learn. 4 , 656–665. https://doi.org/10.1037/0278-7393.4.6.656 (1978).
Article Google Scholar
Stojić, S., Topić, V. & Nadasdy, Z. Children and adults rely on different heuristics for estimation of durations. Sci. Rep. 13 , 1077. https://doi.org/10.1038/s41598-023-27419-4 (2023).
Lamprou-Kokolaki, M., Nédélec, Y., Lhuillier, S. & van Wassenhove, V. Distinctive features of experiential time: Duration, speed and event density. Conscious. Cogn. 118 , 103635. https://doi.org/10.1016/j.concog.2024.103635 (2024).
Poynter, D. In [Adavances in Psychology] Time and Human Cognition: A Life-Span Perspective Vol. 59 (eds Levin, I. & Zakay, D.) Ch. 8, 305–331 (Elsevier, 1989).
Treisman, M. Temporal discrimination and the indifference interval. Implications for a model of the “internal clock”. Psychol. Monogr. 77 , 1–31. https://doi.org/10.1037/h0093864 (1963).
Article CAS PubMed Google Scholar
Hoagland, H. The physiological control of judgments of duration: Evidence for a chemical clock. J. Gen. Psychol. 9 , 267–287. https://doi.org/10.1080/00221309.1933.9920937 (1933).
Gibbon, J., Church, R. M. & Meck, W. H. Scalar timing in memory. Ann. N. Y. Acad. Sci. 423 , 52–77. https://doi.org/10.1111/j.1749-6632.1984.tb23417.x (1984).
Article ADS CAS PubMed Google Scholar
Zakay, D. & Block, R. A. Temporal cognition. Curr. Dir. Psychol. Sci. 6 , 12–16. https://doi.org/10.1111/1467-8721.ep11512604 (1997).
Sabat, M., Haładus, B., Klincewicz, M. & Nalepa, G. J. Cognitive load, fatigue and aversive simulator symptoms but not manipulated zeitgebers affect duration perception in virtual reality. Sci. Rep. 12 , 15689. https://doi.org/10.1038/s41598-022-18520-1 (2022).
Polgári, P., Jovanovic, L., van Wassenhove, V. & Giersch, A. The processing of subthreshold visual temporal order is transitory and motivation-dependent. Sci. Rep. 13 , 7699. https://doi.org/10.1038/s41598-023-34392-5 (2023).
Kovarski, K. et al. Movie editing influences spectators’ time perception. Sci. Rep. 12 , 20084. https://doi.org/10.1038/s41598-022-23992-2 (2022).
Ziat, M., Saoud, W., Prychitko, S., Servos, P. & Grondin, S. Malleability of time through progress bars and throbbers. Sci. Rep. 12 , 10400. https://doi.org/10.1038/s41598-022-14649-1 (2022).
Boned, J. & López-Moliner, J. Duration judgments are mediated by the similarity with the temporal context. Sci. Rep. 12 , 22575. https://doi.org/10.1038/s41598-022-27168-w (2022).
Jording, M., Vogel, D. H. V., Viswanathan, S. & Vogeley, K. Dissociating passage and duration of time experiences through the intensity of ongoing visual change. Sci. Rep. 12 , 8226. https://doi.org/10.1038/s41598-022-12063-1 (2022).
Eagleman, D. M. & Pariyadath, V. Is subjective duration a signature of coding efficiency? Philos. Trans. R. Soc. B. 364 , 1841–1851. https://doi.org/10.1098/rstb.2009.0026 (2009).
Rose, D. & Summers, J. Duration illusions in a train of visual stimuli. Perception 24 , 1177–1187. https://doi.org/10.1068/p241177 (1995).
Grondin, S. Timing and time perception: A review of recent behavioral and neuroscience findings and theoretical directions. Atten. Percept. Psychophys. 72 , 561–582. https://doi.org/10.3758/app.72.3.561 (2010).
Mauk, M. D. & Buonomano, D. V. The neural basis of temporal processing. Annu. Rev. Neurosci. 27 , 307–340 (2004).
Gori, M., Amadeo, M. B., Pavani, F., Valzolgher, C. & Campus, C. Temporal visual representation elicits early auditory-like responses in hearing but not in deaf individuals. Sci. Rep. 12 , 19036. https://doi.org/10.1038/s41598-022-22224-x (2022).
Polver, S., Háden, G. P., Bulf, H., Winkler, I. & Tóth, B. Early maturation of sound duration processing in the infant’s brain. Sci. Rep. 13 , 10287. https://doi.org/10.1038/s41598-023-36794-x (2023).
Terhune, D. B., Russo, S., Near, J., Stagg, C. J. & Kadosh, R. C. GABA predicts time perception. J. Neurosci. 34 , 4364–4370. https://doi.org/10.1523/jneurosci.3972-13.2014 (2014).
Article PubMed PubMed Central Google Scholar
Xiong, Y. Z., Guan, S. C. & Yu, C. A supramodal and conceptual representation of subsecond time revealed with perceptual learning of temporal interval discrimination. Sci. Rep. 12 , 10668. https://doi.org/10.1038/s41598-022-14698-6 (2022).
Uno, K. & Yokosawa, K. Cross-modal correspondence between auditory pitch and visual elevation modulates audiovisual temporal recalibration. Sci. Rep. 12 , 21308. https://doi.org/10.1038/s41598-022-25614-3 (2022).
Koch, G., Oliveri, M. & Caltagirone, C. Neural networks engaged in milliseconds and seconds time processing: Evidence from transcranial magnetic stimulation and patients with cortical or subcortical dysfunction. Philos. Trans. R. Soc. B. 364 , 1907–1918. https://doi.org/10.1098/rstb.2009.0018 (2009).
Lalonde, R. & Hannequin, D. The neurobiological basis of time estimation and temporal order. Rev. Neurosci. 10 , 151–173. https://doi.org/10.1515/revneuro.1999.10.2.151 (1999).
Allman, M. J. & Meck, W. H. Pathophysiological distortions in time perception and timed performance. Brain 135 , 656–677. https://doi.org/10.1093/brain/awr210 (2012).
Critchley, M. The Parietal Lobes (Hafner Press, 1953).
Pacella, V. et al. Temporal judgments of actions following unilateral brain damage. Sci. Rep. 12 , 21668. https://doi.org/10.1038/s41598-022-26070-9 (2022).
Article ADS PubMed PubMed Central Google Scholar
Peer, M., Salomon, R., Goldberg, I., Blanke, O. & Arzy, S. Brain system for mental orientation in space, time, and person. Proc. Natl. Acad. Sci. USA 112 , 11072–11077. https://doi.org/10.1073/pnas.1504242112 (2015).
Tang, L. et al. Neural correlates of temporal presentness in the precuneus: A cross-linguistic fMRI study based on speech stimuli. Cereb. Cortex 31 , 1538–1552. https://doi.org/10.1093/cercor/bhaa307 (2021).
Csikszentmihalyi, M. Flow: The Psychology of Optimal Experience (HarperCollins, 1990).
Hancock, P. A. et al. A meta-analysis of flow effects and the perception of time. Acta Psychol. 198 , 102836. https://doi.org/10.1016/j.actpsy.2019.04.007 (2019).
Article CAS Google Scholar
Esterman, M., Noonan, S. K., Rosenberg, M. & Degutis, J. In the zone or zoning out? Tracking behavioral and neural fluctuations during sustained attention. Cereb. Cortex 23 , 2712–2723. https://doi.org/10.1093/cercor/bhs261 (2013).
Terashima, H., Kihara, K., Kawahara, J. I. & Kondo, H. M. Common principles underlie the fluctuation of auditory and visual sustained attention. Q. J. Exp. Psychol. 74 , 705–715. https://doi.org/10.1177/1747021820972255 (2021).
Download references
HMK was supported by the Japan Society for the Promotion of Science KAKENHI grants (nos. 20H01789 and 22K18659).
Author information
Authors and affiliations.
School of Psychology, Chukyo University, 101-2 Yagoto Honmachi, Showa, Nagoya, Aichi, 466-8666, Japan
Hirohito M. Kondo
Department of Psychology, Faculty of Natural Sciences, University of Stirling, Stirling, UK
Elena Gheorghiu
Faculty of Psychology, University of Lisbon, Lisbon, Portugal
Ana P. Pinheiro
You can also search for this author in PubMed Google Scholar
Contributions
HMK: writing–original draft, writing–review and editing; EG: writing–review and editing; APP: writing–review and editing. All authors gave final approval for publication.
Corresponding author
Correspondence to Hirohito M. Kondo .
Ethics declarations
Competing interests.
The authors declare no competing interests.
Additional information
Publisher's note.
Springer Nature remains neutral with regard to jurisdictional claims in published maps and institutional affiliations.
Rights and permissions
Open Access This article is licensed under a Creative Commons Attribution 4.0 International License, which permits use, sharing, adaptation, distribution and reproduction in any medium or format, as long as you give appropriate credit to the original author(s) and the source, provide a link to the Creative Commons licence, and indicate if changes were made. The images or other third party material in this article are included in the article's Creative Commons licence, unless indicated otherwise in a credit line to the material. If material is not included in the article's Creative Commons licence and your intended use is not permitted by statutory regulation or exceeds the permitted use, you will need to obtain permission directly from the copyright holder. To view a copy of this licence, visit http://creativecommons.org/licenses/by/4.0/ .
Reprints and permissions
About this article
Cite this article.
Kondo, H.M., Gheorghiu, E. & Pinheiro, A.P. Malleability and fluidity of time perception. Sci Rep 14 , 12244 (2024). https://doi.org/10.1038/s41598-024-62189-7
Download citation
Published : 29 May 2024
DOI : https://doi.org/10.1038/s41598-024-62189-7
Share this article
Anyone you share the following link with will be able to read this content:
Sorry, a shareable link is not currently available for this article.
Provided by the Springer Nature SharedIt content-sharing initiative
Quick links
- Explore articles by subject
- Guide to authors
- Editorial policies
Sign up for the Nature Briefing newsletter — what matters in science, free to your inbox daily.

ORIGINAL RESEARCH article
Feel the time. time perception as a function of interoceptive processing.
- 1 Department of Psychology, Università Cattolica del Sacro Cuore, Milan, Italy
- 2 Applied Technology for Neuro-Psychology Lab, IRCCS Istituto Auxologico Italiano, Milan, Italy
- 3 Institute of Cognitive Sciences and Technologies, National Research Council, Rome, Italy
The nature of time is rooted in our body. Constellations of impulses arising from the flesh constantly create our interoceptive perception and, in turn, the unfolding of these perceptions defines human awareness of time. This study explored the connection between time perception and interoception and proposes the Interoceptive Buffer saturation (IBs) index. IBs evaluates subjects’ ability to process salient stimuli from the body by measuring subjective distortions of interoceptive time perception, i.e., the estimated duration of tactile interoceptive stimulations. Thirty female healthy subjects were recruited through consecutive sampling and assessed for common variables related to interoceptive alterations: depressive symptoms (Beck Depression Inventory, BDI-II), eating disorders (EDI-3) risk, and anxiety levels (State Trait Anxiety Inventory, STAI). Interoceptive cardiac accuracy (IAc) was assessed as well. Subjects performed verbal time estimation of interoceptive stimuli (IBs) delivered using a specifically designed interoceptive tactile stimulator, as well as verbal time estimation of visual and auditory stimuli. Results showed that IBs index positively correlated with IAc, and negatively with EDI-3 Drive for Thinness (DT) risk subscale. Moreover, IBs index was positively predicted by IAc, and negatively predicted by DT and somatic factors of depression. Our results suggest that underestimations in interoceptive time perception are connected to different psychological conditions characterized by a diminished processing of high salience stimuli from the body. Conversely, overestimations of the duration of interoceptive stimuli appear to be function of subjects’ ability to correctly perceive their own bodily information. Evidence supported IBs index, fostering the concept of interoceptive treatments for clinical purposes.
Introduction
Time perception is a fundamental element of human awareness. Our consciousness, our ability to perceive the world around us and, ultimately, our very sense of self are shaped upon our perception of time in loop connecting memories of the past, present sensations and expectations about the future. Yet, the way we perceive time is widely debated.
Scalar Expectancy Theory ( Gibbon et al., 1984 ) is one of the most accepted frameworks of time perception ( Church, 1984 ; Treisman et al., 1990 ). A core tenet of SET is an internal clock with a pacemaker-accumulator component. Pulses emitted by the pacemaker are stored in the accumulator, and the amount of units stored in a finite span influences sample frequency and our time perception. A high pulse rate will store more units in the accumulator, therefore leading to overestimation in subjective perception, whereas a low pulse rate will produce opposite effects. Recent developments of SET included memory and decision making components along with the pacemaker-accumulator unit, providing a more efficient neurocognitive framework for time awareness ( Gibbon et al., 1984 ; Lui et al., 2011 ). Moreover, the Attentional Gate Model ( Zakay and Block, 1996 ) introduced attention as mediator of the mode switch between the pacemaker and the accumulator. Specifically, attention can control the mode switch ( Wearden and Penton-Voak, 1995 ; Droit-Volet and Meck, 2007 ; Wearden et al., 2010 ; Ogden et al., 2015a ) in such a way that, if the switch is open, some emitted pulses can be lost therefore contracting our perception of time ( Burle and Casini, 2001 ; Wittmann, 2009 ; Ogden, 2013 ).
The pacemaker-accumulator framework has been connected to an embodied model of time perception ( Wittmann and van Wassenhove, 2009 ; Wittmann et al., 2010 ) by several authors. In this perspective, bodily states and emotions represent central elements whereas high physiological arousal ( Gil and Droit-Volet, 2012 ; Grecucci et al., 2014 ; Ogden et al., 2015a ; Yoo and Lee, 2015 ; Mioni et al., 2016 ) can increase the pulse frequency of the pacemaker, creating overestimation of subjective time perception ( Wittmann, 2009 ). Numerous other authors also suggested that arousal and bodily signals are deeply connected with subjective time awareness ( Droit-Volet and Gil, 2009 ; Gil and Droit-Volet, 2012 ; Pollatos et al., 2014 ; Ogden et al., 2015a , b ), leading to the conclusion that perception of time is intimately rooted in our body.
An embodied perspective of time is also supported by various computational neuroscientific models that highlight the importance of the body and of bodily movements. Evidence from Tomassini and Morrone (2016) suggested that subjective perception of time is connected to the motor cortex whereas sensory-motor conflicts integration contributes to subjective distortion of time across different modalities. In a similar way, Orgs et al. (2013) linked the perception of time to the processing of human movements that subsides visual and motor cortical areas. Moreover, complementary evidence identified a direct link between temporal evaluation and visuo-motor representation of motor actions, highlighting the connection between time perception and body movements ( Gavazzi et al., 2013 ) also on representative level. Perception of time appears therefore as a fundamental requirement of several functions related to the body. To this account, Buonomano and Laje (2010) proposed the concept of “population clocks” that envisions perception of time as an emerging trait of recurrently connected neural networks that encode time, and specifically motor time, in the activity patterns of a population of neurons ( Buonomano, 2014 ). Along with previous discussed evidence, these converging data support therefore an embodied perspective of time perception in humans ( Kranjec and Chatterjee, 2010 ; Wittmann, 2014 ) which integrates several sources, from emotions to body movements, to create our awareness of time.
Further support to the embodied perspective of time comes from Craig’s recent work on the “interoceptive matrix” and its relations to human time awareness. The interoceptive matrix located in the anterior insular cortex (AIC) receives afferent inputs from small diameter sensory fibers through the lamina I spinothalamocortical pathway, which carries fundamental information from all the tissues of the body ( Craig, 2002 , 2003 , 2009 ; Gu et al., 2013 ; Gu and FitzGerald, 2014 ) creating interoceptive perceptions. Recent studies showed selective activation of the AIC in time perception ( Rao et al., 2001 ; Coull, 2004 ; Lewis and Miall, 2006 ; Livesey et al., 2007 ) specifically within the range of sub-seconds to seconds, confirming AIC as one of the core constituents of human awareness of time.
A central component of the interoceptive matrix is the interoceptive buffer ( Craig, 2009 ) that processes and compares interoceptive information with previous and past states of the body, in order to predict future conditions of the organism ( Friston, 2009 , 2010 ; Friston et al., 2011 ; Seth, 2013 ; Ondobaka et al., 2017 ). These interoceptive predictions serve to optimize the functioning of the organism, thus promoting prediction regulation—a control-theoretic process that has been characterized in terms of allostasis ( Sterling, 2012 ) or, more formally, as a prediction error (or free-energy) minimization ( Seth et al., 2011 ; Seth, 2013 ; Suzuki et al., 2013 ).
Craig (2009) proposed that the interoceptive buffer may play a key role in time perception as well. This is because the buffer has a finite dimension and it can be easily filled up with interoceptive inputs, altering our perception of time. Specifically, high rate of salient stimuli can saturate the finite dimension of the buffer, speeding up the sampling frequency, effectively slowing ( Tse et al., 2004 ; Campbell and Bryant, 2007 ; van Wassenhove et al., 2008 ; Wittmann and Paulus, 2008 ; Droit-Volet et al., 2011 ) the perception of time, which will appear to “stand still to the subjective observer” ( Craig, 2009 ). Contrary, when the interoceptive buffer is not filled up “large intervals of time in the objective world can appear to pass quickly” ( Craig, 2009 ).
Nonetheless, some studies revealed a paradox in time perception, when high rates of high salience stimuli can produce opposite effects ( Droit-Volet and Meck, 2007 ; Droit-Volet and Gil, 2009 ). These findings may be explained by a functional processing lateralization of different interoceptive valences. Specifically, AIC appeared to be asymmetrically activated in definite conditions, whereas parasympathetic inputs are preferentially processed by the mid and left insula, while sympathetic activity is usually processed by the right AIC ( Craig, 2009 ). According to Craig’s emotional asymmetry perspective, when we experience a dominant sympathetic arousal, stimuli processed in the right AIC speed up the sample rate frequency, accumulating pulses in the internal clock, leading therefore to overestimation of subjective time perception. Conversely, when we are engaged in a parasympathetic (e.g., affiliative) activation, stimuli are preferentially processed by the left AIC, leading to a subjective underestimation of time due to a lack of sympathetic activity ( Craig, 2009 ).
The present study started from the hypothesis that time perception is intimately related to the functioning of the interoceptive buffer ( Craig, 2009 ). However, while previous studies explored how bodily states can alter subjective time perception, our study focused on the opposite possibility: that is, the possibility to assess the degree of bodily input processing through distortions of interoceptive time perception. The key idea of Interoceptive Buffer saturation (IBs) index is to assess parasympathetic interoceptive stimuli, which are preferentially processed by the left insula ( Gordon et al., 2013 ) for distortions in subjective time estimation due to the sympathetic workload of the coactive right insula. Parasympathetic interoceptive stimuli are reproducible through a specific kind of touch called affective touch ( Olausson et al., 2002 , 2016 ). This kind of touch encompasses activation of C-T afferents connected to the interoceptive system. To this goal, IBs design uses a custom developed “interoceptive stimulator” able to send interoceptive tactile inputs, consequently measuring distortion in subjective time estimation of these stimuli. The estimated degree of time distortion can therefore provide insight on subject’s bodily information processing due to the relative interference of other interoceptive inputs in the subjective time estimation.
To the best of our knowledge, interoceptive buffer has never been operationalized neither experimentally explored, therefore our results can provide powerful theoretical and clinical insights regarding the relationship between bodily states and interoceptive processing.
From a practical point of view, the rationale behind the IBs index is to reverse engineer the connection between the interoceptive matrix and the subjective perception of time, feeding interoceptive stimuli through the C-T afferents in the secondary touch system connected to the lamina I spinothalamocortical pathway. Using the IBs methodology, subjective overestimations of time would suggest a dominance of sympathetic arousal, while subjective underestimations of time would suggest the opposite condition.
According to the emotional asymmetry framework C-T (affective) touch ( Olausson et al., 2002 ; Ackerley et al., 2014a , b ; Liljencrantz and Olausson, 2014 ) comprises a parasympathetic activation primarily processed by the left AIC ( Gordon et al., 2013 ) that might lead to an underestimation of time perception in healthy subjects ( Craig, 2009 ). Nonetheless, contextual interferences caused by sympathetic stimuli—such as high arousing negative ones—processed by the right AIC should interfere with this endogenous time base creating distortions in perception towards an overestimation of time.
Numerous studies suggested an asymmetric effect of sympathetic and parasympathetic input on time perception. Indeed, time perception of parasympathetic interoceptive related stimuli appeared underestimated in normal conditions without sympathetic dominant activation ( Ogden et al., 2015b ); conversely, induced sympathetic stimuli are able to directly alter the internal time baseline, paradoxically leading to a more accurate perception ( Mella et al., 2011 ) endorsing the notion of an emotional advantage for homeostatic regulation ( Ogden et al., 2015a ) as also confirmed by other authors ( Angrilli et al., 1997 ; Buetti and Lleras, 2012 ; Droit-Volet et al., 2013 ; Pezzulo et al., 2018 ).
Comprehending and studying the interoceptive buffer has paramount value. Correct access to interoceptive information is key to allostasis and adaptive regulation of the organism, whereas different conditions such as anorexia nervosa ( Pollatos et al., 2008 ) depression ( Dunn et al., 2007 ) and chronic pain ( Di Lernia et al., 2016b ) appeared connected to alterations in interoceptive processing. Although several other indexes are currently available to assess different interoceptive factors ( Garfinkel et al., 2015 ), IBs index may provide an advanced instrument with the ability not only to identify specific alterations but also the nature and the direction of the processes involved in these alterations. Common interoceptive deficits can be therefore connected to low buffer saturation levels indicating a diminished processing of stimuli arising from the body (i.e., anorexia nervosa, depression) but also with high saturation levels of negative arousal stimuli (i.e., chronic pain, anxiety) that can impair the perception of other inputs. Furthermore, IBs might also detect altered processing before the presence of actual deficits, providing an early indicator of clinical conditions not yet manifested.
To test IBs methodology, the study utilized a stream of interoceptive parasympathetic stimuli sent through the C-T afferents in the secondary touch system connected to the lamina I spinothalamocortical pathway, consequentially measuring for subjects’ distortions in time perception. Stimuli were delivered using an interoceptive stimulator specifically developed for the task. Considering aforementioned body of evidence, we hypothesized that healthy subjects will underestimate the duration of parasympathetic C-T interoceptive stimuli. Furthermore, we hypothesized that IBs index (i.e., the degree of duration estimation of interoceptive stimulation) will positively correlate with interoceptive accuracy (IAc) as a proxy of insula’s activity ( Pollatos et al., 2007a ). Moreover, we hypothesized that several psycho-physiological conditions that are known to interfere with AIC activity will influence IBs as well, leading to distortions in time perception accordingly to subjects’ sympathetic and parasympathetic balance. Specifically, we hypothesized that depressive symptoms ( Dunn et al., 2007 , 2010 ; Pollatos et al., 2009 ; Paulus and Stein, 2010 ; Wiebking et al., 2015 ) and eating disorder tendencies ( Pollatos et al., 2008 ), will interfere in a negative direction, while anxiety symptoms ( Whyman and Moos, 1967 ; Pollatos et al., 2009 , 2014 ; Dunn et al., 2010 ; Paulus and Stein, 2010 ; Yoo and Lee, 2015 ) and other sympathetic stimuli ( Ogden et al., 2015a ) in a positive one.
We tested these hypotheses on healthy subjects assessing for common variables connected to interoceptive alterations. We assessed for risk of anorexia nervosa through EDI-3 ( Garner et al., 1983 ) Drive for Thinness subscale ( Eshkevari et al., 2012 ), and depressive symptoms through Beck Depression Inventory (BDI-II; Beck et al., 1961 ). Furthermore, we assessed anxiety factors through State Trait Anxiety Inventory (STAI; Spielberger et al., 1970 ) and endogenous interoceptive cardiac accuracy (IAc) as well ( Schandry, 1981 ).
Materials and Methods
Participants.
As part of a larger enlisting procedure in the university campus, 30 female subjects were recruited through consecutive sampling. Age (mean = 25.87 years, SD = 6.616) and BMI (mean = 20.827, SD = 2.24) were comparable to other healthy samples used in interoceptive studies ( Garfinkel et al., 2015 ). Sample was composed only by female subjects to avoid somatosensory differences in perception due to gender related factors ( Fillingim et al., 2009 ) as suggested by Ogden et al. (2015b) . Furthermore, a solely female sample ensured no differences related to scales sensitivity, especially regarding eating disorder risk assessed by EDI-3 ( Garner et al., 1983 ; Clausen et al., 2011 ; Eshkevari et al., 2012 ).
Exclusion criteria were the presence of current psychological or physical diagnoses, alterations in tactile perception (paraesthesia), allodynia and heart related conditions. Subjects were asked to avoid pharmacological medications in the 12 h before the experiment and nicotine and caffeine in the 2 h before the experiment.
This study was carried out in accordance with the recommendations of the Ethics Committee of Catholic University of Sacred Heart of Milan with written informed consent from all subjects. All subjects gave written informed consent in accordance with the Declaration of Helsinki (2008). The protocol was approved by the Ethics Committee of Catholic University of Sacred Heart of Milan.
On arrival, subjects received information about the experiment and proceeded to give written consent. Following a brief anamnestic interview and a series of psychological questionnaires, subjects were connected to a portable ECG device with Ag/AgCl electrodes to perform the IAc task. At the end of the task, electrodes were removed and subjects performed IBs, audio and video tasks. IBs, audio and video tasks were presented in randomized order.
Psychological Assessment
After their arrival subjects took part to a brief anamnestic interview with a psychologist specialized in psychopathological and personality assessment. After anamnestic data collection they performed a battery of questionnaires. Depressive symptoms were assessed through the BDI-II ( Beck et al., 1961 ). BDI-II is a 21 items self-report questionnaire with strong literature support. Anxiety symptoms were assessed through the well validated STAI ( Spielberger et al., 1970 ). STAI is a 40-item scale that provided measure of state (STAI-S) and trait (STAI-T) anxiety. Risks for eating disorders were assessed through EDI-3 ( Garner et al., 1983 ) risk subscales ( Eshkevari et al., 2012 ). EDI-3 risk subscales assessed three different aspect of eating disorders risk: Drive for Thinness (DT), Bulimia (B) and Body Dissatisfaction (BD). Global risk index (EDRC) is composed summing the scores of these three subscales.
Interoceptive Accuracy
An IAc score was established with the Schandry heart beat task ( Schandry, 1981 ) through a portable ECG unit sampling at 250 Hz ( Villarrubia et al., 2014a , b ; Stojanović et al., 2015 ; Ševcík et al., 2015 ; Hugeng and Kurniawan, 2016 ) with Ag/AgCl electrodes. Time intervals were 25, 35, 45 and 100 s. Accuracy index was calculated with the following formula: 1/4∑(1 − (|recorded heartbeats − counted heartbeats|)/recorded heartbeats).
We focused on cardiac interoception not only because Schandry task is considered the standard measure for IAc, but also because Craig (2009) suggested that time perception might be fundamentally based upon cardiorespiratory function.
Interoceptive Stimulation
The Interoceptive Buffer saturation (IBs) task applied interoceptive parasympathetic stimuli and asked the participants to estimate the duration of these stimuli. While there are several different kinds of interoceptive stimuli, for the goal of this study we used light touch as primary parasympathetic input ( Ackerley et al., 2014b ). Neuroanatomical evidence identified specific C tactile (C-T) afferents, which report directly to the AIC ( Gordon et al., 2013 ) that are exquisitely sensitive to light touch ( Vallbo et al., 1999 ; Ackerley et al., 2014b ). Unmyelinated C-T fibers selectively respond to slow tactile brushing stimuli between 1 cm/s and 10 cm/s ( Crucianelli et al., 2013 ; McGlone et al., 2014 ). Therefore, we used an instrument explicitly developed to distribute precise C-T stimuli (Figure 1 ) and specifically programmed for the IBs task.
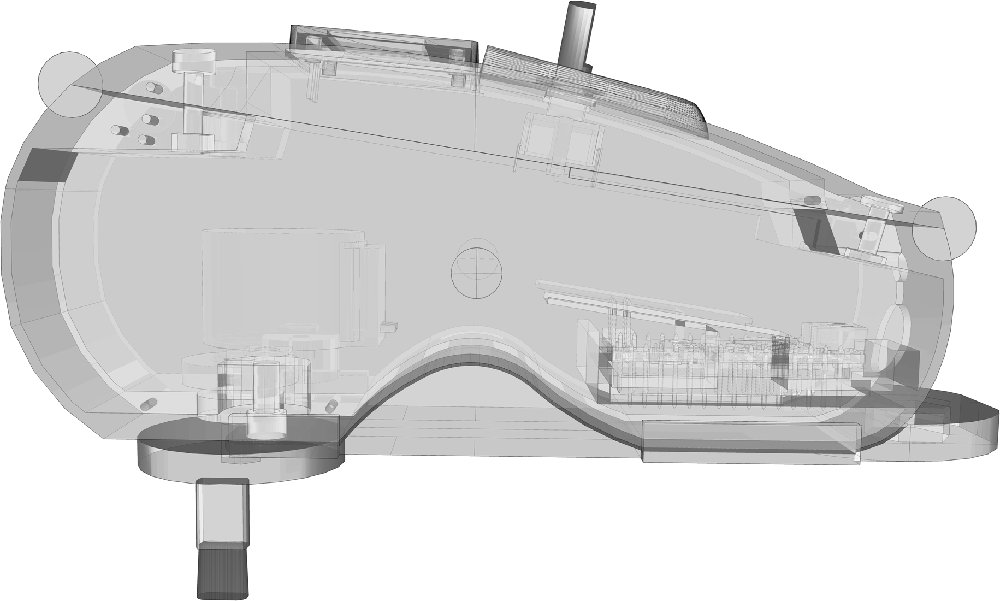
Figure 1 . Interoceptive Stimulator.
Unmyelinated C-fiber tactile afferents are a specific type of fibers that can be found in not glabrous skin, constituting a secondary touch system that projects in the AIC ( Olausson et al., 2002 ). They have been specifically identified in the facial skin ( Nordin, 1990 ) and in the forearm ( Vallbo et al., 1995 , 1999 ; Wessberg et al., 2003 ). They are receptive to low force and low velocity strokes stimuli ( Ackerley et al., 2014a , b ). They exhibit a particular firing behavior with and inverted U relationship with stroke velocity ( Löken et al., 2009 ), a tendency to fatigue ( Iggo, 1960 ; Wessberg et al., 2003 ) and an after-discharge pattern that may present secondary characteristic such as delayed acceleration ( Vallbo et al., 1995 , 1999 ).
For such reason, the interoceptive stimulator has been designed to provide continuous interoceptive stimuli on the forearm, accounting for all C-T afferents factors. C-T afferents showed their maximal mean firing frequency at slow stroking velocity of 3 cm/s ( Ackerley et al., 2014a ), thus the interoceptive stimulator was set to move the brush tip at the exact speed of 3 cm/s (± 0.5 cm/s). The brush tip had an oval shape area of ≈35 mm 2 , to match the receptive field of a human C-T afferent ( Wessberg et al., 2003 ; Liljencrantz and Olausson, 2014 ). The brush used a constant force <2.5 mN that is reported as C-T threshold by different authors ( Nordin, 1990 ; Vallbo et al., 1999 ; Macefield, 2009 ), measured according to Vallbo et al. (1999) . Furthermore, the brush design has been specifically developed to avoid fatigue and inexcitability ( Iggo, 1960 ; Wessberg et al., 2003 ). As a matter of fact, C-T afferents decrease their firing rate to zero after 5 s of continuous stimulation ( Liljencrantz and Olausson, 2014 ). Therefore, the brush tip moved in a circular pattern (10 cm of total length) at specific velocity of 3 cm/s plus a linear velocity of 0.5 cm/s. Considering circular pattern, receptive field area, brush tip dimensions (8.6 × 4.1 mm) and strokes velocity, a single cluster of C-T afferents received stimulation only for ≈0.3 s (0.28 s) within a single revolution, widely below the 5 s limit. The rest span between field stimuli allowed possible after-discharges to be expressed within the active test duration. Furthermore, 6 s resting phase between single trials and 1 min resting phase between stimulation blocks ensured an acceptable recovery of the C-T afferent fields. Delayed acceleration did not have a particular relevance for our design, considering that is not associated with subjective sensations ( Vallbo et al., 1995 , 1999 ).

Interoceptive Buffer Saturation (IBs) Index
The IBs task is based on the Tactile Estimation Task (TET) for spatial recognition, but the protocol is adapted to temporal estimation ( Kramer et al., 2011 ), thus providing a simple and non-invasive procedure to assess the degree of estimation of duration of the interoceptive stimulus. The task consists in delivering interoceptive brush strokes to subject’s left volar forearm, and successively asking for verbal time estimation (VET) of the time passed. The choice of VET 1 is due to its effectiveness in probing time perception related to visual, auditory ( Gil and Droit-Volet, 2011 ) and tactile dimensions ( Ogden et al., 2015b ) assessing for alterations in pulse rates within the pacemaker-accumulator ( Mioni et al., 2016 ). Previous studies focused upon inducing distortions in the inner flow of time, trough external inductive stimuli. Conversely, IBs index will use the natural flow of time inside the interoceptive matrix to probe the saturation of interoceptive buffer.
Previous studies regarding interoceptive touch utilized stimulation at different velocity (>20 cm/s) or different target sites (i.e., palms of the hands) as control procedures. These control tasks rely upon A-fibers stimulation and these fibers are selectively activated by high velocities/high pressure stimuli or selectively present in the palm of the hands ( Ackerley et al., 2014a , b ; Liljencrantz and Olausson, 2014 ; McGlone et al., 2014 ). Nonetheless, these kind of control conditions were not suitable for the present study design due to the fact that perceived duration depends on perceived speed ( Tomassini et al., 2011 ), excluding the possibility to use fast stimuli (speed > 20 cm/s) as control for interoceptive touch ( Crucianelli et al., 2013 ; Ackerley et al., 2014a ). Additionally, slow brush stimuli on the palm of the hands showed hedonic (parasympathetic) velocity-independent valence ( Ackerley et al., 2014b ), which could compromise IBs task design. Moreover, different clinical subjects exhibited deficits in tactile perception connected to A-fibers ( Keizer et al., 2011 , 2012 ; Stanton et al., 2013 ; Catley et al., 2014 ), thus A-fibers stimulation cannot be reliably used as control procedure in perspective of IBs index. Therefore, common audio and visual time estimation tasks were performed as control procedures upon separate sensory modalities.
Subjects were seated in a chair in a comfortable room while the clinician explained the experiment. They were instructed to give a “subjective time estimation of the stimulation”. They were instructed not to count the time passing, to pay attention to the feeling of the stimulation and to close their eyes for the entire duration of the procedure. To avoid counting, clinician suggested focusing on the physical sensation of the stimulation. Subjects were instructed that stimuli could last between “1 and 30 s approximately”, to avoid levelling underestimation effect ( Ogden et al., 2015b ). Subjects laid their left arm on the table in front of the clinician, bare skin ( Tsakiris et al., 2011 ; Ackerley et al., 2014b ). The clinician began the experiment starting the training block on the stimulator program delivering three brush stimuli of 7, 21, and 15 s, allowing the subject to familiarize with the procedure. No feedbacks were provided for effective durations or for performances. After each stimulus, a pause of 6 s allowed clinician to ask the subject, “ How many seconds do you feel the stimulus lasted ?” After the training procedure, clinician started the experimental task with randomized time durations. IBs index task delivered stimuli from 8 s to 18 s, at fixed intervals (8, 10, 12, 14, 16, 18). The experimental task provided six randomized predetermined stimuli per block, for three randomized blocks. Partial accuracy index for each time interval was calculated with the following formula: 1/3∑((time estimation − real time)/real time). Total index was calculated as mean of partial indexes.
Audio and Video Time Estimation
Audio and video estimation tasks were common tasks frequently used and well described in the literature. Following Kramer et al. (2011) , audio task presented a series of audio amplitude-steady complex tones. Video task replicated procedures from Wearden et al. (1998) substituting tones with a 4 × 4 cm light blue square presented on an iPad. Stimuli durations were 100, 200, 500, 1000 and 3000 ms replicated six times each and presented in random order. Audio task had a training procedure of 1000, 300 and 1500 ms. Video task had a training procedure of 1500, 1000 and 500 ms. Subjects were not informed about durations or performances ( Wearden et al., 1998 ; Grondin, 2010 ; Kramer et al., 2011 ) but they were informed that stimuli could last between 50 ms and 4000 ms ( Ogden et al., 2015b ). After each stimulus subjects wrote the estimated duration in milliseconds on a data collection grid. Accuracy indexes were calculated with the following formula: 1/6∑((time estimation − real time)/real time). Total index was calculated as mean of partial indexes.
Statistical Analyses
To verify underestimation of interoceptive C-T stimuli, a series of one sample t -test were used to determine if mean time estimation for every single interval differed significantly from real time values. The same procedure has also been applied to audio and video mean estimations.
A repeated measures ANOVA was run for IBs partial indexes between first, second and last stimulation block to verify that IBs task did not have any effect on the buffer.
A repeated measures ANOVA was run for IBs, audio and video accuracy indexes to identify significant differences between accuracy verbal estimation scores in different sensory modalities. Bonferroni post hoc was run to identify difference between groups.
Due to known limitations ( Dunn et al., 2010 ) inherent assessment scales used, different factor structures have been implemented to better explore relationship between variables. Specifically, analyses implemented a two-factors structure for BDI-II to explore somatic and cognitive depression factors ( Steer et al., 1999 ; Storch et al., 2004 ), and a four-factors structure for STAI to explore the presence and the absence of anxiety elements, both in state and trait dimensions ( Vigneau and Cormier, 2008 ). Furthermore, scatterplot graphs and literature regarding interaction between interoception and depression ( Dunn et al., 2007 , 2010 ; Pollatos et al., 2009 ) suggested a quadratic negative relationship between BDI-II somatic factor and IBs.
Correlation analyses were run for variables of main interest. Moreover, a multiple regression analysis was conducted with IBs index as dependent variable and IAc, EDI DT subscale and BDI-II somatic symptoms as predictors.
Following literature suggestions ( Pollatos et al., 2009 ; Dunn et al., 2010 ), a second multiple regression analysis was conducted with IBs index as dependent variable and IAc, EDI DT subscale, BDI-II somatic symptoms and interaction between the somatic component of BDI-II and the positive factor of STAI-S as predictors. All variables were centered before entering the regression analysis. The positive factor of STAI-S and the somatic factor of BDI-II were also z-standardized before calculating the interaction term. All the low level terms were left in the regression, as per methodological recommendations ( Aiken et al., 1991 ). Residual plots were checked along with normality for observed standardized and unstandardized residuals. The same regression analyses were also conducted with audio and video accuracy indexes in substitution of IBs index.
Sample Psychological Characteristics
Total sample of N = 30 showed high levels of trait anxiety (mean = 42.90, SD = 9.732) and moderate to high level of state anxiety (mean = 35.80 SD = 7.406). Results were comparable to other previous studies ( Aktekin et al., 2001 ; Pollatos et al., 2007b , 2009 ).
Depressive symptoms were higher (mean = 7.60, SD = 5.157) than previous samples in interoceptive studies ( Pollatos et al., 2009 ) with subjects in range of mild clinical depression ( Steer et al., 1999 ; Storch et al., 2004 ) allowing us to meaningfully explore relationship with this variable.
Nonetheless, depressive symptoms levels were comparable to normative data for similar populations ( Storch et al., 2004 ). EDI-3 subscales assessed moderate risks of ED specifically related to BD (mean = 12.77, SD = 7.938; BD) and Drive for Thinness (mean = 7.47, SD = 6.564; DT). Several significant correlations were found between psychometric variables. Results are summarized in Tables 1 , 2 . Scatterplot distributions are provided in Figure 2 .
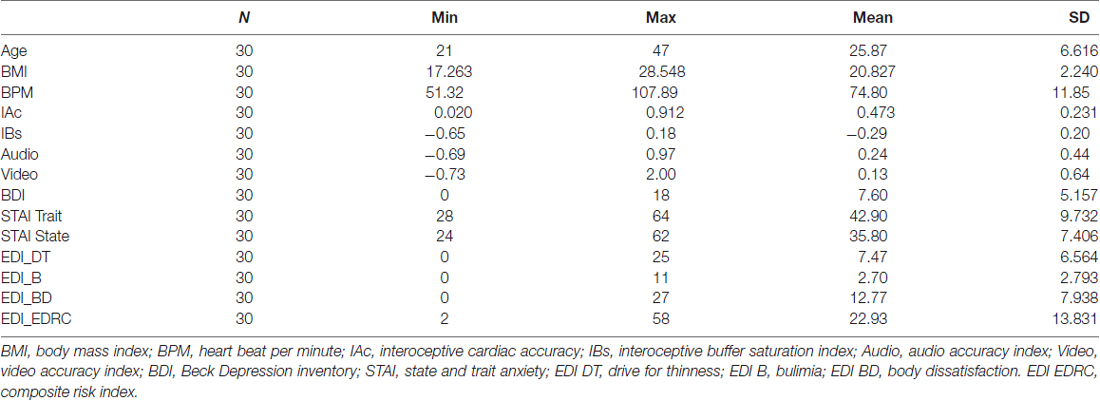
Table 1 . Sample characteristics and psychological assessment.
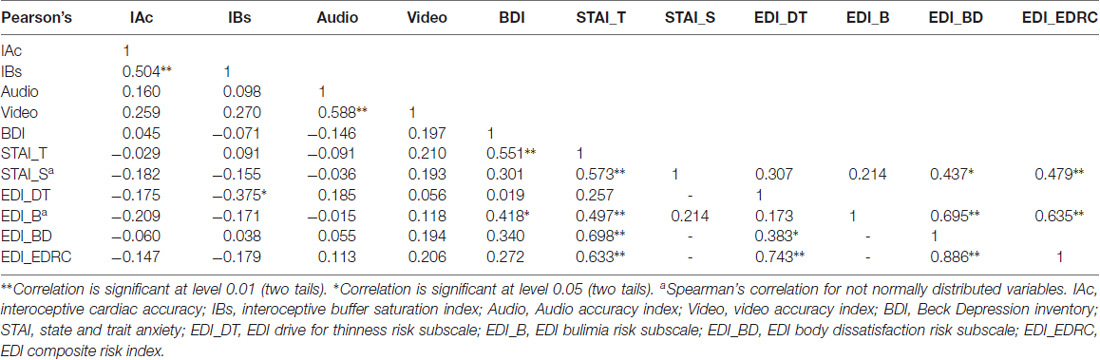
Table 2 . Correlation analyses for normally distributed variables of main interest.
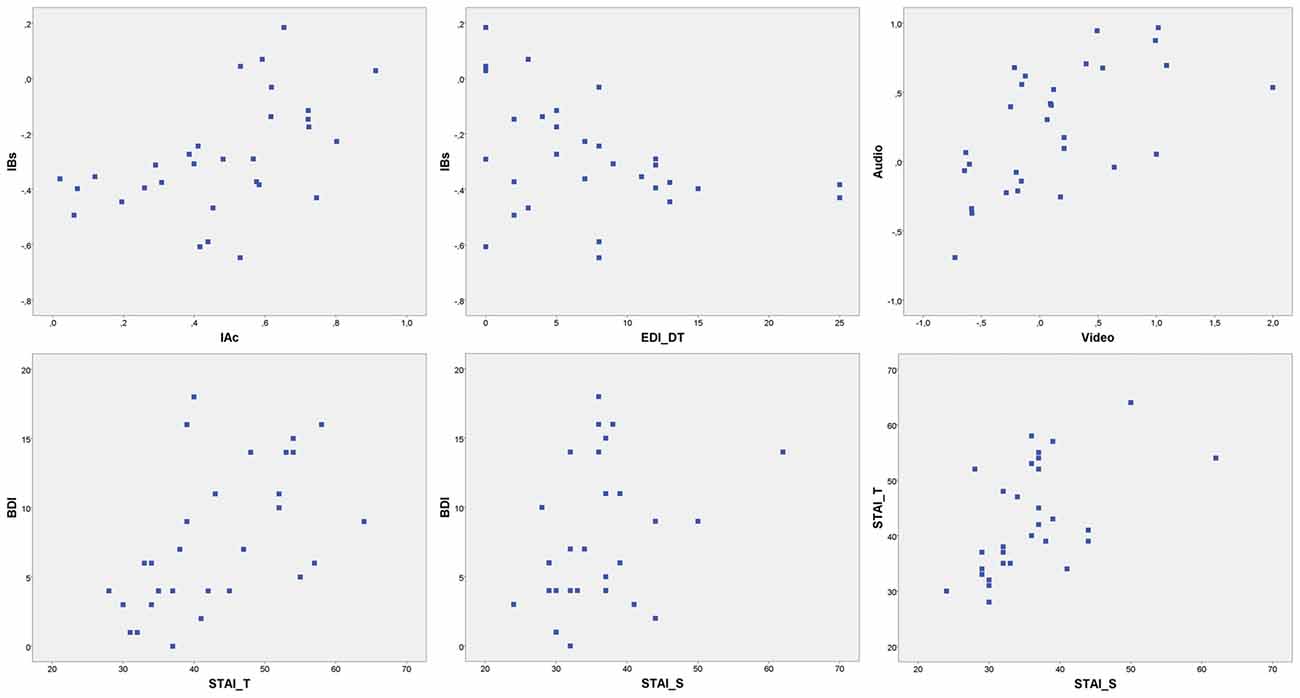
Figure 2 . Scatterplot distributions. IBs, interoceptive buffer saturation index; IAc, interoceptive cardiac accuracy; EDI_DT, EDI drive for thinness risk subscale; Audio, Audio accuracy index; Video, video accuracy index; BDI, Beck Depression inventory; STAI_T, STAI trait anxiety; STAI_S, STAI state anxiety.
Interoceptive cardiac accuracy ( Schandry, 1981 ) is a measure of heart-rate detection ability, and this sensitivity has been correlated with activation in the anterior insula ( Pollatos et al., 2007a ) and provided a standard measure of interoceptive awareness ( Garfinkel et al., 2015 ). IAc mean score was 0.473 (SD = 0.231) and median was 0.50. IAc significantly positively correlated with IBs index ( r = 0.504, p = 0.005). Results are summarized in Table 2 .
Interoceptive Buffer Saturation Index
As hypothesized, healthy subjects significantly underestimated durations of interoceptive stimuli. Mean scores were normally distributed. A series of one sample t -test showed significantly underestimation of mean time perception of interoceptive stimuli for all time spans: 8 s ( t (29) = −7.396, p < 0.001, d = −1.35), 10 s ( t (29) = −5.628, p < 0.001, d = −1.02), 12 s ( t (29) = −8.162, p < 0.001, d = −1.49), 14 s ( t (29) = −7.004, p < 0.001, d = −1.27), 16 s ( t (29) = −6.955, p < 0.001, d = −1.27), 18 s ( t (29) = −7.111, p < 0.001, d = −1.298). Results are summarized in Figure 3 .
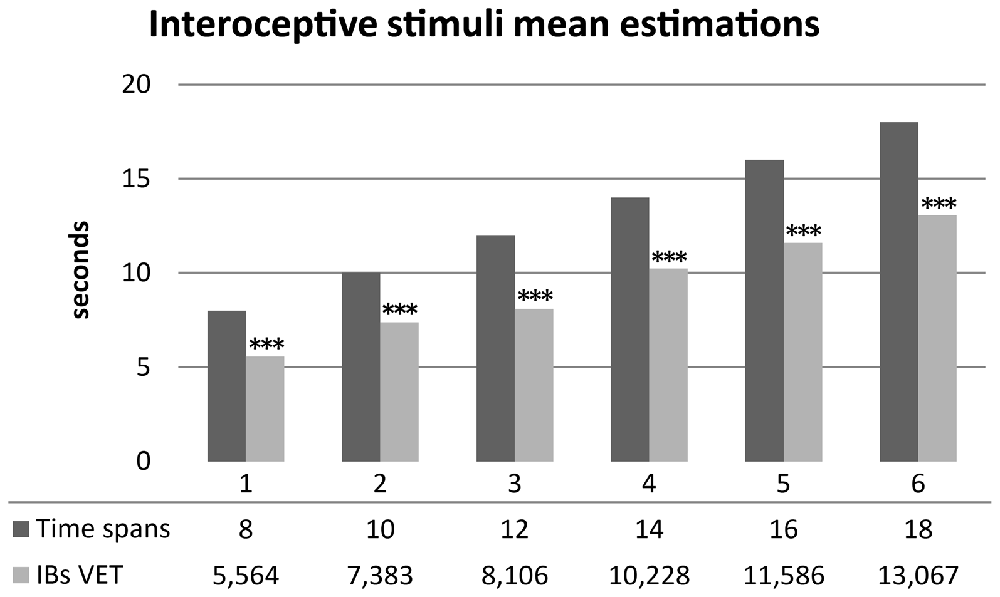
Figure 3 . Mean time estimations of interoceptive tactile stimuli across different time spans. Interoceptive Buffer saturation (IBs) VET, mean verbal interoceptive time estimation. * p <0.05, ** p < 0.01, *** p < 0.001.
As hypothesized, IBs task did not have any manipulative effect on the buffer, confirming the effectiveness of the task as assessment instrument. A repeated measures ANOVA showed no statistically significant differences on partial IBs indexes between first, second and last stimulation block ( F (2,58) = 0.142, p = 0.868, η p 2 = 0.005). Results indicated that IBs task did not alter subjects’ endogenous baseline 2 .
As hypothesized, Pearson’s correlation analyses showed a significantly positive linear relationship between IBs and IAc ( r = 0.504, p = 0.005) and significantly negative linear relationship between IBs and EDI Drive for Thinness ( r = −0.375, p = 0.041). Results are summarized in Table 2 .
Scatterplot and literature ( Dunn et al., 2007 , 2010 ) suggested a quadratic relationship between depression and interoceptive processing. As hypothesized, IBs correlation with a quadratic term of BDI-II somatic factor approach significance for a negative relationship ( r = −0.358, p = 0.052).
A multiple regression analysis was conducted with IBs index as dependent variable and IAc, EDI Drive for Thinness and quadratic transformation of BDI-II somatic factor as predictors. Sample dimension assured an adequate power ( Harrell, 2015 ) for the analysis. As hypothesized, variables significantly predicted IBs ( R 2 = 0.443, F (3,26) = 6.880, p < 0.001). Beta standardized coefficients indicated that IAc significantly positively predicted IBs ( β = 0.420, p = 0.009) while EDI Drive for Thinness ( β = −0.306, p ≤ 0.05) and somatic depressive factor of BDI-II ( β = −0.324, p ≤ 0.05) 3 significantly negatively predicted saturation levels in the interoceptive buffer.
To explore relationship between anxiety and interoceptive buffer levels, a second multiple regression analysis was conducted with IBs index as dependent variable and IAc, EDI Drive for Thinness, BDI-II somatic quadratic transformation and interaction between the somatic component of BDI-II and the positive STAI-S factor as predictors ( Pollatos et al., 2009 ; Dunn et al., 2010 ; Paulus and Stein, 2010 ). Variables significantly predicted IBs ( R 2 = 0.529, adjusted R 2 = 0.406, F (6,23) = 4.306, p < 0.005). IAc positively predicted saturation levels in the buffer ( β = 0.465, p = 0.005) while EDI Drive for Thinness ( β = −0.345, p = 0.028) and BDI-II somatic factor ( β = −0.394, p = 0.018) significantly negatively predicted IBs. Interaction term between somatic BDI-II factor and positive STAI-S factor approached significance ( β = 0.318, p = 0.083). Sample size was slightly underpowered for this regression model, nonetheless methodological recommendations indicated that multiple regression is quite robust to small sample if adjusted R 2 is considered in substitution of R 2 ( Austin and Steyerberg, 2015 ).
One sample t -tests were performed between audio and video time estimations and real time spans. Wilcoxon tests were performed for not-normally distributed variables. Audio estimations did not show any statistically significant difference for 100, 200, 1000 and 3000 ms time spans ( p > 0.05) compared to real time spans. Subjects significantly overestimated 500 ms audio stimuli ( t (29) = 3.958, p < 0.001, d = 0.722). Video estimations did not show any statistically significant difference for 100, 500, 1000 ms time spans ( p > 0.05) compared to real time spans. Subjects significantly overestimated 200 ms video stimuli ( t (29) = 2.242, p = 0.033, d = 0.409) and significantly underestimated 3000 ms video stimuli ( t (29) = −3.032, p = 0.005, d = −0.553). Results are summarized in Figure 4 . One sample t -tests for paired sample were performed between normally distributed audio and video time estimations. Wilcoxon tests were performed for not-normally distributed values. Subjects judged audio stimuli significantly longer than video stimuli in time spans 500, 1000 and 3000 ms ( p = 0.005). A t -test for paired sample was performed between audio and video accuracy indexes without any statistically significant difference ( p = 0.232). Audio and video accuracy indexes significantly positively correlated ( r = 0.588, p = 0.001). Results are summarized in Table 2 . Regression analyses for audio ( p = 0.490) and video ( p = 0.478) accuracy indexes were performed with IAc, EDI_DT and BDI-II somatic factor. Both models failed to reach statistical significance 4 .
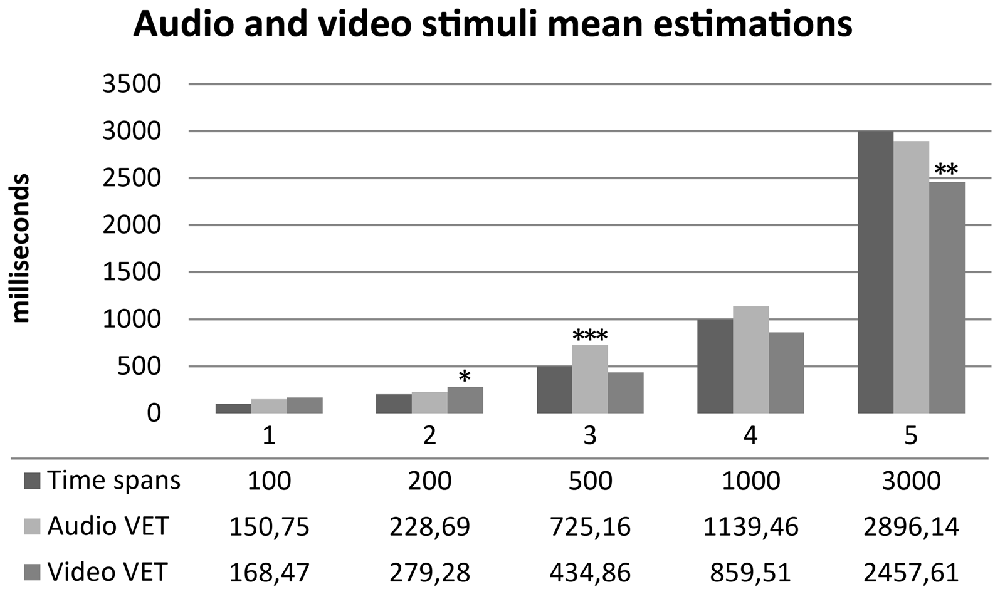
Figure 4 . Mean time estimation of audio and video stimuli across different time spans. Audio VET, mean verbal audio time estimation; Video VET, mean verbal video time estimation. * p <0.05, ** p < 0.01, *** p < 0.001.
Relationship Between Measures
A repeated measures ANOVA with a Greenhouse-Geisser correction was performed between IBs, audio and video accuracy indexes (factor accuracy). Results reported a statistically significant difference between accuracy indexes ( F (1.806,52.371) = 16.037, p < 0.001, η p 2 = 0.356). Post hoc tests using Bonferroni correction revealed that IBs accuracy index was significantly underestimated compared to both audio ( p < 0.001) and video ( p = 0.003) accuracy indexes. No statistically significant differences were found between audio and video accuracy indexes ( p = 0.697) although audio stimuli (mean = 0.241, SD = 0.080) were judged longer than video ones (mean = 0.126, SD = 0.116).
Several statistically significant correlations were found between psychometric variables. Results are summarized in Table 2 .
The study explored the connection between time perception and interoceptive processing, proposing the IBs index as a novel construct able to provide information about co-active processing within the interoceptive matrix (AIC; Craig, 2002 , 2003 , 2009 ). The IBs index relied upon a verbal estimation task of interoceptive stimuli sent through the secondary touch system connected to the lamina I spinothalamocortical pathway. Bidirectional distortions in the subjective time perception of these stimuli provide information about sympathetic and parasympathetic activity in the cortex. The interoceptive buffer is a key component of the interoceptive matrix and it constantly processes and compares information arising from the body, creating bodily meta-representations shaped upon the asymmetrical relationship between the right and the left insula. Understanding saturation levels within the buffer can provide meaningful insight about this relationship, whereas high levels of saturation may indicate large amount of sympathetic stimuli processed in the right insula, while opposite results may indicate that the interoceptive system is actually processing a low amount of high salience sympathetic stimuli from the body.
Recent evidence identified deficits in the insula connected with radically different psychopathological conditions. Chronic pain ( Schaefer et al., 2012 ; Weiss et al., 2014 ; Duschek et al., 2015 ), depression ( Dunn et al., 2007 , 2010 ; Pollatos et al., 2009 ; Sprengelmeyer et al., 2011 ; Sliz and Hayley, 2012 ; Stratmann et al., 2014 ), eating disorders ( Pollatos et al., 2008 ; Fischer et al., 2016 ) and several other conditions ( Rosso et al., 2010 ; Wylie and Tregellas, 2010 ; Hatton et al., 2012 ; Naqvi et al., 2014 ) comprised alterations within the interoceptive network. Nonetheless, it is not clearly understood how radically different conditions can impair the insula network in a similar way.
In our previous work ( Di Lernia et al., 2016a ), we suggested that IBs may provide a better measure of interoceptive processing compared to other available indexes, because it permits to disentangle the differential effects of interoceptive alterations within the matrix. Conceptualizing these alterations in terms of buffer saturation levels suggests that high levels of negative arousing stimuli may impair interoceptive processing through widespread interferences (i.e., interoceptive noise), whereas low stimuli processing may reduce body awareness (i.e., interoceptive silence), with both conditions leading to deficits in interoceptive perception.
The design of the study did not include non-interoceptive control stimuli, as explained in the “Materials and Methods” section. This was due to the nature of the task we adopted, in which different stimulation speeds—which are necessary to perform non interoceptive stimulation—would have yielded incomparable results in a time estimation task. A second equally important implication that defined IBs design is also that interoceptive tactile stimuli are never exclusively related to C-T fibers. Neurophysiological evidence indicated that every C-T related stimulus always comprises a concomitant activation of myelinated tactile fibers processed by the somatosensory cortex ( Roudaut et al., 2012 ). Although a mechanical stimulation such as the one delivered by the interoceptive stimulator can be tuned to produce massive peak activations in C-T tactile afferents, every interoceptive tactile stimulus always partially activates other myelinated fibers ( Crucianelli et al., 2013 ; Ackerley et al., 2014a , b ). Therefore a non-interoceptive control task would have yielded incomparable, albeit informative, results from IBs perspective. This was, among others, one of the prominent reasons according to which we have chosen different sensory modalities (audio and video) as control procedures; also in agreement with protocols to study emotions and time perception that usually compared results across different unrelated perceptive systems. Nonetheless, considering IBs design, only correlations between the index with the Schandry’s task and the questionnaires can be used for an interpretation regarding interoceptive processes.
As hypothesized, results confirmed that healthy subjects significantly underestimated the duration of parasympathetic interoceptive C-T stimuli, compared to actual time spans. Results confirmed previous findings about C-T processing ( Leonard et al., 2014 ; Ogden et al., 2015b ), supporting the methodology used in the present study and the effectiveness of the device developed. Comparing our results with previous studies about sympathetic stimuli ( Droit-Volet et al., 2011 ; Ogden et al., 2015a ) also endorsed Craig’s emotional time asymmetry framework and consequently the rationale behind the IBs index. Significant underestimation of C-T stimuli suggested that healthy subjects without dominant sympathetic activation tend to have a reduced stimuli processing in the right insula buffer, matching a low pulse rate within the interoceptive internal clock therefore experiencing a contraction in time perception due to a lack of salience in bodily arousal.
As hypothesized, IBs index positively correlated and was positively predicted by interoceptive cardiac accuracy (IAc). This result provided strong support regarding the validity of IBs index due to the ontological connection between the buffer and the degree of activation of the insula ( Pollatos et al., 2007a ). Nonetheless, correlation was sufficiently distinct to suggest that IBs index and interoceptive cardiac accuracy are two different, albeit intertwined, constructs.
IBs index negatively correlated and was negatively predicted by EDI-3 Drive for Thinness subscale, suggesting an interesting insight regarding the connection of the buffer with body perception. IAc is significantly reduced in clinical subjects with anorexia nervosa ( Pollatos et al., 2008 ); nonetheless, the nature of these deficits is not really understood even though they can be equally found in radically different conditions ( Di Lernia et al., 2016b ). In our sample, we found no significant correlation between IAc and Drive for Thinness, as expected for healthy females that did have neither interoceptive deficits nor anorexia nervosa. Conversely, we found a negative correlation between IBs and Drive for Thinness risk, suggesting that predisposition for anorexia nervosa can be connected to low saturation levels within interoceptive buffer. Considering our results, low saturation levels in the buffer (IBs) can be described as a hypo-saturation condition whereas the interoceptive matrix processes lower amounts of high salience (i.e., hunger, thirst etc.) stimuli from the body. This impaired processing activity can therefore lead to pervasive alterations in body perception and, ultimately, to the interoceptive deficits and bodily distortions identified in anorexia nervosa ( Pollatos et al., 2008 ). Recent fMRI evidence showed consistent alterations in the insula network both on functional ( Gaudio et al., 2015 ; Kerr et al., 2016 ) and structural level ( Gaudio et al., 2017 ) indicating impaired processing of high salience bodily stimuli in anorexia nervosa ( Wierenga et al., 2015 ), therefore supporting our results. Moreover, anorexic subjects showed no impairment in a time duration task connected to non-interoceptive neutral tactile stimuli ( Spitoni et al., 2015 ) fostering the conclusion that IBs results are strictly connected to processing within insula network and its relationship with body perception.
Depression and anxiety symptoms did not show a direct linear correlation neither with IBs nor IAc accuracy indexes, partially diverging from previous literature results ( Pollatos et al., 2009 ). This difference could be due either to limited sample dimension in our study or to the lack of sensitivity of the two assessment scales we used, which would be in keeping with other previous results ( Dunn et al., 2010 ). Our results indicate a poor effectiveness of BDI-II and STAI questionnaires to assess interoceptive dimensions of depression and anxiety. Dunn et al. (2010) suggested that BDI-II and STAI questionnaires may have confounding overlapping constructs, more directly connected to global severity measures and therefore they might be relatively inappropriate to measure interoceptive components. Our findings, related to a small albeit representative sample, confirmed this perspective also through a significant correlation between the scales.
To effectively assess bodily components of depression and anxiety, we therefore implemented several well validated factor structures. BDI-II was divided into two-factors connected to somatic and cognitive components of depression ( Steer et al., 1999 ; Storch et al., 2004 ). STAI was divided into four-factors that described positive and negative items of anxiety, both in state and trait conditions ( Vigneau and Cormier, 2008 ).
Consequently, we focused on the somatic factor of BDI-II and a more accurate analysis identified a not-linear negative relationship ( Dunn et al., 2007 ) between depressive somatic symptoms and IBs levels. Our results suggested that depression negatively interfered with saturation levels in the buffer promoting a hypo-saturation condition in which depressive somatic components appeared to be related to a diminished processing of high valence arousing stimuli in the right insula. These results are also supported by previous neurophysiological evidence that indicated a hypo-response ( Wiebking et al., 2015 ) along with reduction in gray matter volume of the insula ( Sprengelmeyer et al., 2011 ; Stratmann et al., 2014 ) in major depressive disorders.
In our study, anxiety measured through STAI questionnaire failed to provide statistically significant results. This could be due to the small sample size but more probably to the general construct within the scale that is primarily oriented to global severity measures rather than anxiety positive arousal symptoms ( Dunn et al., 2010 ). Following literature recommendations ( Pollatos et al., 2009 ; Dunn et al., 2010 ; Paulus and Stein, 2010 ), we nonetheless explored the interaction between somatic depressive factor and positive state anxiety factor. The interaction approached a positive statistical significance suggesting some further considerations that, nonetheless, must be considered with caution. Specifically, comorbidity between somatic depressive and anxiety symptoms appeared to have a moderately positive influence upon saturation levels in the buffer, suggesting a biological prevalence of sympathetic arousal preferentially processed in the right insula. Several authors backed this perspective that relied upon the role of high arousal stimuli in the optimal functioning of the organism ( Jänig, 2008 ; Wiech et al., 2010 ; Uddin, 2015 ) supporting the notion of an emotional advantage in perception processing for homeostatic regulatory purposes, which foster enhanced attention to bodily signals ( Ogden et al., 2015a ; Yoo and Lee, 2015 ).
Interestingly, healthy subjects did not systematically underestimate durations of audio and video stimuli. Moreover, a significant difference between IBs, audio and video accuracy indexes suggested secondary implications regarding time perception theories. Specifically, our findings indicated different pacemaker-accumulator units, connected to different sensory modalities. Audio and video indexes showed a positive significant correlation between each other, but failed to significantly correlate to interoceptive time perception indicating separated time processing pathways. Moreover, regression analysis also failed to be significant for the audio and video accuracy indexes, suggesting that audio and video time estimation probably referred to different processing circuits, partially distinct from the interoceptive system. These results are supported by recent literature ( Mioni et al., 2016 ) according to which time perception in depression showed inconclusive results ( Thones and Oberfeld, 2015 ) along with not-inductive time studies in anxiety that also provided mixed results ( Lueck, 2007 ; Brown, 2016 ).
A possible explanation for the significant difference between audio, video and IBs accuracy indexes can be related to the different time spans used, suggesting that time estimation in the range of milliseconds is not susceptible to the same psycho-physiological components that contribute to IBs index. Nonetheless, different studies used time spans within milliseconds range to identify a parasympathetic ( Ogden et al., 2015b ) and sympathetic ( Droit-Volet and Meck, 2007 ; Gil and Droit-Volet, 2011 , 2012 ; Droit-Volet et al., 2013 ; Ogden et al., 2015a ; Thones and Oberfeld, 2015 ; Yoo and Lee, 2015 ; Mioni et al., 2016 ) influence upon the internal clock. Moreover, time spans in the range from seconds to subseconds have often been considered connected to the same time perception mechanisms ( Church, 1984 ) whereas evidence from literature found no difference in time intervals as a factor ( Macar et al., 2002 ). Furthermore, evidence from computational neuroscience suggested that time estimations from millisecond to seconds rely upon the same encoding processing patterns ( Karmarkar and Buonomano, 2007 ). Karmarkar and Buonomano (2007) also suggested that short time intervals—as the lower ones in the audio and video estimation tasks—are also probably connected to a nonlinear time metric encoded in local neural networks and therefore they should be even more sensible to the psychophysiological variables that contribute to IBs index. In a similar manner, Craig suggested that the interoceptive network might “provide a basis for the human capacity to perceive and estimate time intervals in the range of seconds to subseconds” ( Craig, 2009 ) also supporting the possibility to coherently explore different time spans. This evidence suggested that time spans in milliseconds range should be sensible to alterations in bodily processing, thus excluding the duration of the stimuli as a factor and allowing the design of the study to select the most appropriate and the most sensible time intervals for each sensory modality.
Considering our results, it is therefore possible that time perception is processed differently depending on the sensory modality investigated and that time information collected by several different internal clocks are sub-sequentially merged in a global time perception awareness, composed by different elements according to their contextual salience. This hypothesis remains to be tested in future studies.
Interoceptive Buffer, Active Inference and Predictive Coding
From a more formal, predictive coding (or interoceptive inference) perspective, buffer saturation can be understood in terms of well-known mechanisms of precision (or gain) control during (Bayesian) inference. One essential role of interoceptive inference is integrating various interoceptive signals to form an estimate of the state of the body (e.g., heart rate as well as gastric and respiratory signals to assess momentary fatigue or fear), based on which the organism can take adaptive action ( Pezzulo, 2014 ; Pezzulo et al., 2015 ). Importantly, while forming this estimate, the reliability of all the interoceptive signals (as well as of prior information) must be evaluated, too; signals that have higher salience or precision (or lower uncertainty) are weighted more and have higher impact on the inference, whereas lower-precision signals have lesser impact and in some cases can be also disattended or ignored—thus implementing a form of precision (or gain) control over interoceptive processing. Within this framework, signals that are highly salient or have high behavioral significance (e.g., threats) would be plausibly afforded a high gain, hence dominating the inference and reducing the impact of other signals (aka, saturating the buffer). This is in general an adaptive mechanism, which would afford priority to the most important signals; but in some cases, it can become maladaptive. For example, expecting the environment to be too volatile might lead to over-sampling it (like, e.g., in anxiety) and to a systematic overestimation of the precision of sensory and interoceptive signals. Conversely, other pathological conditions (e.g., anorexia) may be linked to interoceptive precision down-regulation, and thus to a systematically diminished processing of stimuli arising from the body (aka, silencing one of the channels of the buffer rather than saturating it). This framework would shed light on the different ways interoceptive inference can become aberrant, due (for example) to the fallacious up- or down-regulation of the precision of interoceptive (or other) signals—and how this in turn would produce maladaptive behavior that is specific of different clinical conditions ( Friston et al., 2014 ).
Clinical Implication of Interoceptive Buffer Saturation
IBs index can provide insight regarding interoceptive alterations in apparently different conditions, nonetheless this knowledge may serve other purposes besides simple assessment. Specifically, pathological conditions with an hyper-saturated status might present alterations in bodily signals connected to an overflow of sympathetic-related stimuli in the buffer (i.e., interoceptive noise) lowering the access to other interoceptive information ( Di Lernia et al., 2016a ). Conversely, hypo-saturated conditions may be connected to a general impairment of whole stimuli processing that limited inputs (i.e., interoceptive silence) from actually reaching AIC.
If confirmed, this perspective may have important implications beyond assessment, foreseeing the concept of “interoceptive treatments”. As a matter of fact, clinical conditions (i.e., anxiety or chronic pain) characterized by an overflow of sympathetic related interoceptive stimuli (i.e., hyper-saturation) may benefit from an interoceptive parasympathetic stimulation with low variance to promote co-activation of the left insula, reducing processing in the right AIC with the ultimate goal of contrasting symptoms severity. Conversely, if a clinical condition will show hypo-saturation due to a block that impairs interoceptive information to actually reach the insula (i.e., depression or anorexia nervosa), interoceptive treatments based upon interoceptive stimuli with high variance could provide a way to foster high salience processing in the right AIC, restoring a correct access to bodily information. If confirmed, albeit highly theoretical, these perspectives can provide innovative insights in treatment fields, for several different psychopathological conditions.
Limitations
Several limitations impaired study design and results. Sample size was reduced, nonetheless consecutive sampling provided highly informative data, and literature confirmed an adequate statistical power ( Austin and Steyerberg, 2015 ; Harrell, 2015 ) related to number of subjects and statistical analyses performed.
A solely female sample ensured comparable results on somatosensory related tests ( Fillingim et al., 2009 ) and psychological assessment ( Garner et al., 1983 ; Clausen et al., 2011 ; Eshkevari et al., 2012 ) nevertheless the design of the study did not explore IBs in male population.
The study utilized audio and video stimuli as control procedures due to the fact that somatic non-interoceptive conditions (i.e., different velocities or different body locations) were not suitable within IBs design. This choice allowed us to explore time perception upon different sensory modalities. However, the absence of somatic control stimuli did not allow us to explore possible differences in somatic non-interoceptive processing. Moreover, interoceptive stimulation always presents concomitant activation of Aβ receptors ( Ackerley et al., 2014a , b ; Ogden et al., 2015b ) therefore non-interoceptive stimuli with a parasympathetic component may correlate with IBs index. These problems might be addressed by using reliable somatic controls, such as vibrational stimuli that specifically target ( Liljencrantz and Olausson, 2014 ) Pacinian corpuscles at a frequency between 150 Hz and –300 Hz ( Roudaut et al., 2012 ). Nonetheless, this kind of stimulation requires a specifically designed prototype not available during this study.
BDI-II and STAI questionnaires presented several limitations regarding construct measures, overlapping theoretical relevance, and effectiveness to measure interoceptive components ( Dunn et al., 2010 ). In our study, these limitations were partially addressed through several factor structures nonetheless a different model of anxiety and depression could provide better assessment of interoceptive dimensions. Specifically, the tripartite model of mood disorders ( Clark and Watson, 1991 ) might provide a more effective framework for interoceptive related components. This model relies upon the Mood and Anxiety Symptom Questionnaire (MASQ-S; Watson et al., 1995 ; Keogh and Reidy, 2000 ) to assess several dimensions connected to symptoms of nonspecific general distress, symptoms specific to depression and symptoms specific to anxiety. Specifically, MASQ-S subscales Anxious Arousal (17 items) and Anhedonic Depression (22 items) might provide better results compared to global severity measures reported by BDI-II and STAI. Unfortunately, Italian validation of the instrument was not available at the time of this study.
Lastly, literature suggested that belief and expectations can limit Schandry task validity to assess for interoceptive cardiac awareness ( Ring et al., 2015 ). Nonetheless, heart beat perception task ( Schandry, 1981 ) remained the most validate and most reliable instrument for IAc assessment ( Pollatos et al., 2007a ; Critchley and Garfinkel, 2015 ; Garfinkel et al., 2015 ).
Conclusion and Future Directions
Our results provided a powerful theoretical insight regarding the relationship between time perception, interoceptive processing and psycho-physiological conditions. Distortions in interoceptive time perception recorded by IBs index appeared to be function of different sympathetic and parasympathetic co-activation processes within insula cortex.
Nonetheless, different questions remain to be explored, promoting future research directions. Specifically, it will be fundamental to test IBs index proposal on different pathological conditions connected to insula deficits, such as chronic pain, anorexia nervosa and depression also comparing results to healthy subjects’ performances.
Furthermore, IBs results may support the concept of “interoceptive treatments” as clinical applications for a new non-pharmacological option to treat a variety of disorders characterized by interoceptive network alterations. These kinds of treatments, tailored upon specific dysregulations of the insula cortex, might provide powerful instruments to reduce symptoms severity in those conditions that are resistant to pharmacological medications, without any side effect or interaction with concomitant therapies ( Di Lernia et al., 2016a ; Riva et al., 2016 , 2017 ). Interoceptive treatments may therefore prove themselves an effective option to promote a balanced functioning of the organism both in clinical and healthy population, opening a brand new field of medicine and neuroscience.
Author Contributions
DDL: conceptualization, investigation, writing—original draft, hardware and software development. DDL and SS: methodology. SS, GP, EP, PC and GR: writing—review, editing and supervision.
Conflict of Interest Statement
The authors declare that the research was conducted in the absence of any commercial or financial relationships that could be construed as a potential conflict of interest.
Acknowledgments
SS and GR were funded by the MIUR PRIN research project “Unlocking the memory of the body: Virtual Reality in Anorexia Nervosa” (201597WTTM).
- ^ On the other hand, we dismissed time production and reproduction tasks because that are usually dependent upon attention and working memory processes “rather than variation in internal clock” ( Mioni et al., 2016 ).
- ^ IBs is proposed as an assessing instrument; therefore we checked if the stimulation had any kind of effect upon subject’s temporal perception. We verified this by confronting mean estimations of the first stimulation block with mean estimations on the last stimulation block. An effect of the interoceptive stimulation would have produced variations in temporal estimation across blocks. Since we did not observe significative differences in time estimation between first and last stimuli, across all the protocol, we concluded that the stimulation itself did not have any impact on subject’s endogenous time perception.
- ^ BDI-II somatic factor quadratic transformation ( β = −0.324, p = 0.037) | Model with untransformed term R 2 = 0.461, F (4,25) = 5.337, p < 0.003, IAc β = 0.420, p = 0.01, EDI_DT β = −0.308, p = 0.05, BDI-II somatic untransformed term β = −0.136, p = 0.369, BDI-II somatic quadratic transformed term β = −0.303, p = 0.053. Residuals K-S > 0.200, S-W = 0.928.
- ^ Model with untransformed term: Audio accuracy index p = 0.611, Video accuracy index p = 0.288.
Ackerley, R., Backlund Wasling, H., Liljencrantz, J., Olausson, H., Johnson, R. D., and Wessberg, J. (2014a). Human C-tactile afferents are tuned to the temperature of a skin-stroking caress. J. Neurosci. 34, 2879–2883. doi: 10.1523/jneurosci.2847-13.2014
PubMed Abstract | CrossRef Full Text | Google Scholar
Ackerley, R., Carlsson, I., Wester, H., Olausson, H., and Backlund Wasling, H. (2014b). Touch perceptions across skin sites: differences between sensitivity, direction discrimination and pleasantness. Front. Behav. Neurosci. 8:54. doi: 10.3389/fnbeh.2014.00054
Aiken, L. S., West, S. G., and Reno, R. R. (1991). Multiple Regression: Testing and Interpreting Interactions. New York, NY: Sage.
Google Scholar
Aktekin, M., Karaman, T., Senol, Y. Y., Erdem, S., Erengin, H., and Akaydin, M. (2001). Anxiety, depression and stressful life events among medical students: a prospective study in Antalya, Turkey. Med. Educ. 35, 12–17. doi: 10.1111/j.1365-2923.2001.00726.x
Angrilli, A., Cherubini, P., Pavese, A., and Manfredini, S. (1997). The influence of affective factors on time perception. Percept. Psychophys. 59, 972–982. doi: 10.3758/bf03205512
Austin, P. C., and Steyerberg, E. W. (2015). The number of subjects per variable required in linear regression analyses. J. Clin. Epidemiol. 68, 627–636. doi: 10.1016/j.jclinepi.2014.12.014
Beck, A. T., Ward, C. H., Mendelson, M., Mock, J., and Erbaugh, J. (1961). An inventory for measuring depression. Arch. Gen. Psychiatry 4, 561–571. doi: 10.1001/archpsyc.1961.01710120031004
Brown, J. (2016). A Relationship Between Time Perception and State-Anxiety. (American Journal of Undergraduate Research, AJUR) (Online version).
Buetti, S., and Lleras, A. (2012). Perceiving control over aversive and fearful events can alter how we experience those events: an investigation of time perception in spider-fearful individuals. Front. Psychol. 3:337. doi: 10.3389/fpsyg.2012.00337
Buonomano, D. V. (2014). Neural dynamics based timing in the subsecond to seconds range. Adv. Exp. Med. Biol. 829, 101–117. doi: 10.1007/978-1-4939-1782-2_6
Buonomano, D. V., and Laje, R. (2010). Population clocks: motor timing with neural dynamics. Trends Cogn. Sci. 14, 520–527. doi: 10.1016/j.tics.2010.09.002
Burle, B., and Casini, L. (2001). Dissociation between activation and attention effects in time estimation: implications for internal clock models. J. Exp. Psychol. Hum. Percept. Perform. 27, 195–205. doi: 10.1037/0096-1523.27.1.195
Campbell, L. A., and Bryant, R. A. (2007). How time flies: a study of novice skydivers. Behav. Res. Ther. 45, 1389–1392. doi: 10.1016/j.brat.2006.05.011
Catley, M. J., O’Connell, N. E., Berryman, C., Ayhan, F. F., and Moseley, G. L. (2014). Is tactile acuity altered in people with chronic pain? a systematic review and meta-analysis. J. Pain 15, 985–1000. doi: 10.1016/j.jpain.2014.06.009
Church, R. M. (1984). Properties of the internal clock. Ann. N Y Acad. Sci. 423, 566–582. doi: 10.1111/j.1749-6632.1984.tb23459.x
Clark, L. A., and Watson, D. (1991). Tripartite model of anxiety and depression: psychometric evidence and taxonomic implications. J. Abnorm. Psychol. 100, 316–336. doi: 10.1037//0021-843x.100.3.316
Clausen, L., Rosenvinge, J. H., Friborg, O., and Rokkedal, K. (2011). Validating the eating disorder inventory-3 (EDI-3): a comparison between 561 female eating disorders patients and 878 females from the general population. J. Psychopathol. Behav. Assess. 33, 101–110. doi: 10.1007/s10862-010-9207-4
Coull, J. T. (2004). fMRI studies of temporal attention: allocating attention within, or towards, time. Cogn. Brain Res. 21, 216–226. doi: 10.1016/j.cogbrainres.2004.02.011
Craig, A. D. (2002). How do you feel? Interoception: the sense of the physiological condition of the body. Nat. Rev. Neurosci. 3, 655–666. doi: 10.1038/nrn894
Craig, A. D. (2003). Interoception: the sense of the physiological condition of the body. Curr. Opin. Neurobiol. 13, 500–505. doi: 10.1016/s0959-4388(03)00090-4
Craig, A. D. (2009). Emotional moments across time: a possible neural basis for time perception in the anterior insula. Philos. Trans. R. Soc. Lond. B Biol. Sci. 364, 1933–1942. doi: 10.1098/rstb.2009.0008
Critchley, H. D., and Garfinkel, S. N. (2015). Interactions between visceral afferent signaling and stimulus processing. Front. Neurosci. 9:286. doi: 10.3389/fnins.2015.00286
Crucianelli, L., Metcalf, N. K., Fotopoulou, A. K., and Jenkinson, P. M. (2013). Bodily pleasure matters: velocity of touch modulates body ownership during the rubber hand illusion. Front. Psychol. 4:703. doi: 10.3389/fpsyg.2013.00703
Di Lernia, D., Serino, S., Cipresso, P., and Riva, G. (2016a). Ghosts in the Machine. Interoceptive modeling for chronic pain treatment. Front. Neurosci. 10:314. doi: 10.3389/fnins.2016.00314
Di Lernia, D., Serino, S., and Riva, G. (2016b). Pain in the body. Altered interoception in chronic pain conditions: a systematic review. Neurosci. Biobehav. Rev. 71, 328–341. doi: 10.1016/j.neubiorev.2016.09.015
Droit-Volet, S., Fayolle, S. L., and Gil, S. (2011). Emotion and time perception: effects of film-induced mood. Front. Integr. Neurosci. 5:33. doi: 10.3389/fnint.2011.00033
Droit-Volet, S., and Gil, S. (2009). The time-emotion paradox. Philos. Trans. R. Soc. Lond. B Biol. Sci. 364, 1943–1953. doi: 10.1098/rstb.2009.0013
Droit-Volet, S., and Meck, W. H. (2007). How emotions colour our perception of time. Trends Cogn. Sci. 11, 504–513. doi: 10.1016/j.tics.2007.09.008
Droit-Volet, S., Ramos, D., Bueno, J. L., and Bigand, E. (2013). Music, emotion, and time perception: the influence of subjective emotional valence and arousal? Front. Psychol. 4:417. doi: 10.3389/fpsyg.2013.00417
Dunn, B. D., Dalgleish, T., Ogilvie, A. D., and Lawrence, A. D. (2007). Heartbeat perception in depression. Behav. Res. Ther. 45, 1921–1930. doi: 10.1016/j.brat.2006.09.008
Dunn, B. D., Stefanovitch, I., Evans, D., Oliver, C., Hawkins, A., and Dalgleish, T. (2010). Can you feel the beat? Interoceptive awareness is an interactive function of anxiety- and depression-specific symptom dimensions. Behav. Res. Ther. 48, 1133–1138. doi: 10.1016/j.brat.2010.07.006
Duschek, S., Montoro, C. I., and Reyes Del Paso, G. A. (2015). Diminished interoceptive awareness in fibromyalgia syndrome. Behav. Med. 43, 100–107. doi: 10.1080/08964289.2015.1094442
Eshkevari, E., Rieger, E., Longo, M. R., Haggard, P., and Treasure, J. (2012). Increased plasticity of the bodily self in eating disorders. Psychol. Med. 42, 819–828. doi: 10.1017/s0033291711002091
Fillingim, R. B., King, C. D., Ribeiro-Dasilva, M. C., Rahim-Williams, B., and Riley, J. L. (2009). Sex, gender, and pain: a review of recent clinical and experimental findings. J. Pain 10, 447–485. doi: 10.1016/j.jpain.2008.12.001
Fischer, D., Berberich, G., Zaudig, M., Krauseneck, T., Weiss, S., and Pollatos, O. (2016). Interoceptive processes in anorexia nervosa in the time course of cognitive-behavioral therapy: a pilot study. Front. Psychiatry 7:199. doi: 10.3389/fpsyt.2016.00199
Friston, K. (2009). The free-energy principle: a rough guide to the brain? Trends Cogn. Sci. 13, 293–301. doi: 10.1016/j.tics.2009.04.005
Friston, K. (2010). The free-energy principle: a unified brain theory? Nat. Rev. Neurosci. 11, 127–138. doi: 10.1038/nrn2787
Friston, K., Mattout, J., and Kilner, J. (2011). Action understanding and active inference. Biol. Cybern. 104, 137–160. doi: 10.1007/s00422-011-0424-z
Friston, K. J., Stephan, K. E., Montague, R., and Dolan, R. J. (2014). Computational psychiatry: the brain as a phantastic organ. Lancet Psychiatry 1, 148–158. doi: 10.1016/s2215-0366(14)70275-5
Garfinkel, S. N., Seth, A. K., Barrett, A. B., Suzuki, K., and Critchley, H. D. (2015). Knowing your own heart: distinguishing interoceptive accuracy from interoceptive awareness. Biol. Psychol. 104, 65–74. doi: 10.1016/j.biopsycho.2014.11.004
Garner, D. M., Olmstead, M. P., and Polivy, J. (1983). Development and validation of a multidimensional eating disorder inventory for anorexia nervosa and bulimia. Int. J. Eat. Disord. 2, 15–34. doi: 10.1002/1098-108x(198321)2:2<15::aid-eat2260020203>3.0.co;2-6
CrossRef Full Text | Google Scholar
Gaudio, S., Piervincenzi, C., Beomonte Zobel, B., Romana Montecchi, F., Riva, G., Carducci, F., et al. (2015). Altered resting state functional connectivity of anterior cingulate cortex in drug naïve adolescents at the earliest stages of anorexia nervosa. Sci. Rep. 5:10818. doi: 10.1038/srep10818
Gaudio, S., Quattrocchi, C. C., Piervincenzi, C., Zobel, B. B., Montecchi, F. R., Dakanalis, A., et al. (2017). White matter abnormalities in treatment-naive adolescents at the earliest stages of Anorexia Nervosa: a diffusion tensor imaging study. Psychiatry Res. 266, 138–145. doi: 10.1016/j.pscychresns.2017.06.011
Gavazzi, G., Bisio, A., and Pozzo, T. (2013). Time perception of visual motion is tuned by the motor representation of human actions. Sci. Rep. 3:1168. doi: 10.1038/srep01168
Gibbon, J., Church, R. M., and Meck, W. H. (1984). Scalar timing in memory. Ann. N Y Acad. Sci. 423, 52–77. doi: 10.1111/j.1749-6632.1984.tb23417.x
Gil, S., and Droit-Volet, S. (2011). “Time flies in the presence of angry faces” and depending on the temporal task used!. Acta Psychol 136, 354–362. doi: 10.1016/j.actpsy.2010.12.010
Gil, S., and Droit-Volet, S. (2012). Emotional time distortions: the fundamental role of arousal. Cogn. Emot. 26, 847–862. doi: 10.1080/02699931.2011.625401
Gordon, I., Voos, A. C., Bennett, R. H., Bolling, D. Z., Pelphrey, K. A., and Kaiser, M. D. (2013). Brain mechanisms for processing affective touch. Hum. Brain Mapp. 34, 914–922. doi: 10.1002/hbm.21480
Grecucci, A., Giorgetta, C., Rattin, A., Guerreschi, C., Sanfey, A. G., and Bonini, N. (2014). Time devours things: how impulsivity and time affect temporal decisions in pathological gamblers. PLoS One 9:e109197. doi: 10.1371/journal.pone.0109197
Grondin, S. (2010). Timing and time perception: a review of recent behavioral and neuroscience findings and theoretical directions. Atten. Percept. Psychophys. 72, 561–582. doi: 10.3758/app.72.3.561
Gu, X., and FitzGerald, T. H. (2014). Interoceptive inference: homeostasis and decision-making. Trends Cogn. Sci. 18, 269–270. doi: 10.1016/j.tics.2014.02.001
Gu, X., Hof, P. R., Friston, K. J., and Fan, J. (2013). Anterior insular cortex and emotional awareness. J. Comp. Neurol. 521, 3371–3388. doi: 10.1002/cne.23368
Harrell, F. (2015). Regression Modeling Strategies: With Applications to Linear Models, Logistic and Ordinal Regression and Survival Analysis. Heidelberg: Springer.
Hatton, S. N., Lagopoulos, J., Hermens, D. F., Naismith, S. L., Bennett, M. R., and Hickie, I. B. (2012). Correlating anterior insula gray matter volume changes in young people with clinical and neurocognitive outcomes: an MRI study. BMC Psychiatry 12:45. doi: 10.1186/1471-244x-12-45
Hugeng, H., and Kurniawan, R. (2016). Development of the ‘Healthcor’system as a cardiac disorders symptoms detector using an expert system based on arduino uno. Int. J. Technol. 7,78–87. doi: 10.14716/ijtech.v7i1.1575
Iggo, A. (1960). Cutaneous mechanoreceptors with afferent C fibres. J. Physiol. 152, 337–353. doi: 10.1113/jphysiol.1960.sp006491
Jänig, W. (2008). Integrative Action of the Autonomic Nervous System: Neurobiology of Homeostasis. Cambridge: Cambridge University Press.
Karmarkar, U. R., and Buonomano, D. V. (2007). Timing in the absence of clocks: encoding time in neural network states. Neuron 53, 427–438. doi: 10.1016/j.neuron.2007.01.006
Keizer, A., Smeets, M. A., Dijkerman, H. C., van den Hout, M., Klugkist, I., van Elburg, A., et al. (2011). Tactile body image disturbance in anorexia nervosa. Psychiatry Res. 190, 115–120. doi: 10.1016/j.psychres.2011.04.031
Keizer, A., Smeets, M. A., Dijkerman, H. C., van Elburg, A., and Postma, A. (2012). Aberrant somatosensory perception in Anorexia Nervosa. Psychiatry Res. 200, 530–537. doi: 10.1016/j.psychres.2012.05.001
Keogh, E., and Reidy, J. (2000). Exploring the factor structure of the Mood and Anxiety Symptom Questionnaire (MASQ). J. Pers. Assess. 74, 106–125. doi: 10.1207/S15327752JPA740108
Kerr, K. L., Moseman, S. E., Avery, J. A., Bodurka, J., Zucker, N. L., and Simmons, W. K. (2016). Altered insula activity during visceral interoception in weight-restored patients with anorexia nervosa. Neuropsychopharmacology 41, 521–528. doi: 10.1038/npp.2015.174
Kramer, P., Bressan, P., and Grassi, M. (2011). Time estimation predicts mathematical intelligence. PLoS One 6:e28621. doi: 10.1371/journal.pone.0028621
Kranjec, A., and Chatterjee, A. (2010). Are temporal concepts embodied? A challenge for cognitive neuroscience. Front. Psychol. 1:240. doi: 10.3389/fpsyg.2010.00240
Leonard, J. A., Berkowitz, T., and Shusterman, A. (2014). The effect of friendly touch on delay-of-gratification in preschool children. Q. J. Exp. Psychol. 67, 2123–2133. doi: 10.1080/17470218.2014.907325
Lewis, P. A., and Miall, R. (2006). A right hemispheric prefrontal system for cognitive time measurement. Behav. Processes 71, 226–234. doi: 10.1016/j.beproc.2005.12.009
Liljencrantz, J., and Olausson, H. (2014). Tactile C fibers and their contributions to pleasant sensations and to tactile allodynia. Front. Behav. Neurosci. 8:37. doi: 10.3389/fnbeh.2014.00037
Livesey, A. C., Wall, M. B., and Smith, A. T. (2007). Time perception: manipulation of task difficulty dissociates clock functions from other cognitive demands. Neuropsychologia 45, 321–331. doi: 10.1016/j.neuropsychologia.2006.06.033
Löken, L. S., Wessberg, J., Morrison, I., McGlone, F., and Olausson, H. (2009). Coding of pleasant touch by unmyelinated afferents in humans. Nat. Neurosci. 12, 547–548. doi: 10.1038/nn.2312
Lueck, M. D. (2007). Anxiety levels: do they influence the perception of time? J. Undergrad. Res. X, 1–5.
Lui, M. A., Penney, T. B., and Schirmer, A. (2011). Emotion effects on timing: attention versus pacemaker accounts. PLoS One 6:e21829. doi: 10.1371/journal.pone.0021829
Macar, F., Lejeune, H., Bonnet, M., Ferrara, A., Pouthas, V., Vidal, F., et al. (2002). Activation of the supplementary motor area and of attentional networks during temporal processing. Exp. Brain Res. 142, 475–485. doi: 10.1007/s00221-001-0953-0
Macefield, V. G. (2009). “Tactile C fibers,” in Encyclopedia of Neuroscience , eds M. D. Binder, N. Hirokawa and U. Windhorst (Germany: Springer), 3995–3998.
McGlone, F., Wessberg, J., and Olausson, H. (2014). Discriminative and affective touch: sensing and feeling. Neuron 82, 737–755. doi: 10.1016/j.neuron.2014.05.001
Mella, N., Conty, L., and Pouthas, V. (2011). The role of physiological arousal in time perception: psychophysiological evidence from an emotion regulation paradigm. Brain Cogn. 75, 182–187. doi: 10.1016/j.bandc.2010.11.012
Mioni, G., Stablum, F., Prunetti, E., and Grondin, S. (2016). Time perception in anxious and depressed patients: a comparison between time reproduction and time production tasks. J. Affect. Disord. 196, 154–163. doi: 10.1016/j.jad.2016.02.047
Naqvi, N. H., Gaznick, N., Tranel, D., and Bechara, A. (2014). The insula: a critical neural substrate for craving and drug seeking under conflict and risk. Ann. N Y Acad. Sci. 1316, 53–70. doi: 10.1111/nyas.12415
Nordin, M. (1990). Low-threshold mechanoreceptive and nociceptive units with unmyelinated (C) fibres in the human supraorbital nerve. J. Physiol. 426, 229–240. doi: 10.1113/jphysiol.1990.sp018135
Ogden, R. S. (2013). The effect of facial attractiveness on temporal perception. Cogn. Emot. 27, 1292–1304. doi: 10.1080/02699931.2013.769426
Ogden, R. S., Moore, D., Redfern, L., and McGlone, F. (2015a). The effect of pain and the anticipation of pain on temporal perception: a role for attention and arousal. Cogn. Emot. 29, 910–922. doi: 10.1080/02699931.2014.954529
Ogden, R. S., Moore, D., Redfern, L., and McGlone, F. (2015b). Stroke me for longer this touch feels too short: the effect of pleasant touch on temporal perception. Conscious. Cogn. 36, 306–313. doi: 10.1016/j.concog.2015.07.006
Olausson, H., Lamarre, Y., Backlund, H., Morin, C., Wallin, B. G., Starck, G., et al. (2002). Unmyelinated tactile afferents signal touch and project to insular cortex. Nat. Neurosci. 5, 900–904. doi: 10.1038/nn896
Olausson, H., Wessberg, J., and McGlone, F. (2016). Affective Touch and the Neurophysiology of CT Afferents. New York, NY: Springer.
Ondobaka, S., Kilner, J., and Friston, K. (2017). The role of interoceptive inference in theory of mind. Brain Cogn. 112, 64–68. doi: 10.1016/j.bandc.2015.08.002
Orgs, G., Kirsch, L., and Haggard, P. (2013). Time perception during apparent biological motion reflects subjective speed of movement, not objective rate of visual stimulation. Exp. Brain Res. 227, 223–229. doi: 10.1007/s00221-013-3502-8
Paulus, M. P., and Stein, M. B. (2010). Interoception in anxiety and depression. Brain Struct. Funct. 214, 451–463. doi: 10.1007/s00429-010-0258-9
Pezzulo, G. (2014). Why do you fear the bogeyman? An embodied predictive coding model of perceptual inference. Cogn. Affect. Behav. Neurosci. 14, 902–911. doi: 10.3758/s13415-013-0227-x
Pezzulo, G., Iodice, P., Barca, L., Chausse, P., Monceau, S., and Mermillod, M. (2018). Increased heart rate after exercise facilitates the processing of fearful but not disgusted faces. Sci. Rep. 8:398. doi: 10.1038/s41598-017-18761-5
Pezzulo, G., Rigoli, F., and Friston, K. (2015). Active inference, homeostatic regulation and adaptive behavioural control. Prog. Neurobiol. 134, 17–35. doi: 10.1016/j.pneurobio.2015.09.001
Pollatos, O., Kurz, A. L., Albrecht, J., Schreder, T., Kleemann, A. M., Schöpf, V., et al. (2008). Reduced perception of bodily signals in anorexia nervosa. Eat. Behav. 9, 381–388. doi: 10.1016/j.eatbeh.2008.02.001
Pollatos, O., Laubrock, J., and Wittmann, M. (2014). Interoceptive focus shapes the experience of time. PLoS One 9:e86934. doi: 10.1371/journal.pone.0086934
Pollatos, O., Schandry, R., Auer, D. P., and Kaufmann, C. (2007a). Brain structures mediating cardiovascular arousal and interoceptive awareness. Brain Res. 1141, 178–187. doi: 10.1016/j.brainres.2007.01.026
Pollatos, O., Traut-Mattausch, E., Schroeder, H., and Schandry, R. (2007b). Interoceptive awareness mediates the relationship between anxiety and the intensity of unpleasant feelings. J. Anxiety Disord. 21, 931–943. doi: 10.1016/j.janxdis.2006.12.004
Pollatos, O., Traut-Mattausch, E., and Schandry, R. (2009). Differential effects of anxiety and depression on interoceptive accuracy. Depress. Anxiety 26, 167–173. doi: 10.1002/da.20504
Rao, S. M., Mayer, A. R., and Harrington, D. L. (2001). The evolution of brain activation during temporal processing. Nat. Neurosci. 4, 317–323. doi: 10.1038/85191
Ring, C., Brener, J., Knapp, K., and Mailloux, J. (2015). Effects of heartbeat feedback on beliefs about heart rate and heartbeat counting: a cautionary tale about interoceptive awareness. Biol. Psychol. 104, 193–198. doi: 10.1016/j.biopsycho.2014.12.010
Riva, G., Serino, S., Di Lernia, D., Pavone, E. F., and Dakanalis, A. (2017). Embodied medicine: mens sana in corpore virtuale sano. Front. Hum. Neurosci. 11:120. doi: 10.3389/fnhum.2017.00120
Riva, G., Villani, D., Cipresso, P., Repetto, C., Triberti, S., Di Lernia, D., et al. (2016). Positive and transformative technologies for active ageing. Stud. Health Technol. Inform. 220, 308–315. doi: 10.3233/978-1-61499-625-5-308
Rosso, I. M., Makris, N., Britton, J. C., Price, L. M., Gold, A. L., Zai, D., et al. (2010). Anxiety sensitivity correlates with two indices of right anterior insula structure in specific animal phobia. Depress. Anxiety 27, 1104–1110. doi: 10.1002/da.20765
Roudaut, Y., Lonigro, A., Coste, B., Hao, J., Delmas, P., and Crest, M. (2012). Touch sense: functional organization and molecular determinants of mechanosensitive receptors. Channels 6, 234–245. doi: 10.4161/chan.22213
Schaefer, M., Egloff, B., and Witthöft, M. (2012). Is interoceptive awareness really altered in somatoform disorders? Testing competing theories with two paradigms of heartbeat perception. J. Abnorm. Psychol. 121, 719–724. doi: 10.1037/a0028509
Schandry, R. (1981). Heart beat perception and emotional experience. Psychophysiology 18, 483–488. doi: 10.1111/j.1469-8986.1981.tb02486.x
Seth, A. K. (2013). Interoceptive inference, emotion and the embodied self. Trends Cogn. Sci. 17, 565–573. doi: 10.1016/j.tics.2013.09.007
Seth, A. K., Suzuki, K., and Critchley, H. D. (2011). An interoceptive predictive coding model of conscious presence. Front. Psychol. 2:395. doi: 10.3389/fpsyg.2011.00395
Ševcík, J., Kainz, O., Fecilak, P., and Jakab, F. (2015). System for EKG monitoring. Int. J. Adv. Res. Artif. Intell. 4, doi: 10.14569/IJARAI.2015.040905
Sliz, D., and Hayley, S. (2012). Major depressive disorder and alterations in insular cortical activity: a review of current functional magnetic imaging research. Front. Hum. Neurosci. 6:323. doi: 10.3389/fnhum.2012.00323
Spielberger, C. D., Gorsuch, R. L., and Lushene, R. E. (1970). Manual for The State-Trait Anxiety Inventory. Palo Alto, CA: Consulting Psychologists Press.
Spitoni, G. F., Serino, A., Cotugno, A., Mancini, F., Antonucci, G., and Pizzamiglio, L. (2015). The two dimensions of the body representation in women suffering from Anorexia Nervosa. Psychiatry Res. 230, 181–188. doi: 10.1016/j.psychres.2015.08.036
Sprengelmeyer, R., Steele, J. D., Mwangi, B., Kumar, P., Christmas, D., Milders, M., et al. (2011). The insular cortex and the neuroanatomy of major depression. J. Affect. Disord. 133, 120–127. doi: 10.1016/j.jad.2011.04.004
Stanton, T. R., Lin, C. W., Bray, H., Smeets, R. J., Taylor, D., Law, R. Y., et al. (2013). Tactile acuity is disrupted in osteoarthritis but is unrelated to disruptions in motor imagery performance. Rheumatology 52, 1509–1519. doi: 10.1093/rheumatology/ket139
Steer, R. A., Ball, R., Ranieri, W. F., and Beck, A. T. (1999). Dimensions of the beck depression inventory-II in clinically depressed outpatients. J. Clin. Psychol. 55, 117–128. doi: 10.1002/(sici)1097-4679(199901)55:1<117::aid-jclp12>3.0.co;2-a
Sterling, P. (2012). Allostasis: a model of predictive regulation. Physiol. Behav. 106, 5–15. doi: 10.1016/j.physbeh.2011.06.004
Stojanović, R., Čaplánová, A., Kovačević, Ž., Nemanja, N., and Bundalo, Z. (2015). Alternative approach to addressing infrastructure needs in biomedical engineering programs (Case of emerging economies). Folia Med. Fac. Med. Univ. Sarav. 50, 29–33.
Storch, E. A., Roberti, J. W., and Roth, D. A. (2004). Factor structure, concurrent validity, and internal consistency of the Beck Depression Inventory-Second Edition in a sample of college students. Depress. Anxiety 19, 187–189. doi: 10.1002/da.20002
Stratmann, M., Konrad, C., Kugel, H., Krug, A., Schöning, S., Ohrmann, P., et al. (2014). Insular and hippocampal gray matter volume reductions in patients with major depressive disorder. PLoS One 9:e102692. doi: 10.1371/journal.pone.0102692
Suzuki, K., Garfinkel, S. N., Critchley, H. D., and Seth, A. K. (2013). Multisensory integration across exteroceptive and interoceptive domains modulates self-experience in the rubber-hand illusion. Neuropsychologia 51, 2909–2917. doi: 10.1016/j.neuropsychologia.2013.08.014
Thones, S., and Oberfeld, D. (2015). Time perception in depression: a meta-analysis. J. Affect. Disord. 175, 359–372. doi: 10.1016/j.jad.2014.12.057
Tomassini, A., Gori, M., Burr, D., Sandini, G., and Morrone, C. (2011). Perceived duration of visual and tactile stimuli depends on perceived speed. Front. Integr. Neurosci. 5:51. doi: 10.3389/fnint.2011.00051
Tomassini, A., and Morrone, M. C. (2016). Perceived visual time depends on motor preparation and direction of hand movements. Sci. Rep. 6:27947. doi: 10.1038/srep27947
Treisman, M., Faulkner, A., Naish, P. L., and Brogan, D. (1990). The internal clock: evidence for a temporal oscillator underlying time perception with some estimates of its characteristic frequency. Perception 19, 705–742. doi: 10.1068/p190705
Tsakiris, M., Tajadura-Jiménez, A., and Costantini, M. (2011). Just a heartbeat away from one’s body: interoceptive sensitivity predicts malleability of body-representations. Proc. Biol. Sci. 278, 2470–2476. doi: 10.1098/rspb.2010.2547
Tse, P. U., Intriligator, J., Rivest, J., and Cavanagh, P. (2004). Attention and the subjective expansion of time. Percept. Psychophys. 66, 1171–1189. doi: 10.3758/bf03196844
Uddin, L. Q. (2015). Salience processing and insular cortical function and dysfunction. Nat. Rev. Neurosci. 16, 55–61. doi: 10.1038/nrn3857
Vallbo, A. B., Olausson, H., and Wessberg, J. (1999). Unmyelinated afferents constitute a second system coding tactile stimuli of the human hairy skin. J. Neurophysiol. 81, 2753–2763. doi: 10.1152/jn.1999.81.6.2753
Vallbo, A. B., Olausson, H., Wessberg, J., and Kakuda, N. (1995). Receptive field characteristics of tactile units with myelinated afferents in hairy skin of human subjects. J. Physiol. 483, 783–795. doi: 10.1113/jphysiol.1995.sp020622
van Wassenhove, V., Buonomano, D. V., Shimojo, S., and Shams, L. (2008). Distortions of subjective time perception within and across senses. PLoS One 3:e1437. doi: 10.1371/journal.pone.0001437
Vigneau, F., and Cormier, S. (2008). The factor structure of the State-Trait Anxiety Inventory: an alternative view. J. Pers. Assess. 90, 280–285. doi: 10.1080/00223890701885027
Villarrubia, G., De Paz, J. F., Bajo, J., and Corchado, J. M. (2014a). EKG mobile. Adv. Sci. Technol. Lett. 49, 95–100. doi: 10.14257/astl.2014.49.20
Villarrubia, G., De Paz, J. F., Corchado, J. M., and Bajo, J. (2014b). “EKG intelligent mobile system for home users,” in Advances in Artificial Intelligence—IBERAMIA 2014. IBERAMIA 2014 , eds A. Bazzan and K. Pichara, (Cham: Springer) 8864, 767–778. doi: 10.1007/978-3-319-12027-0_62 Lecture Notes in Computer Science.
Watson, D., Weber, K., Assenheimer, J. S., Clark, L. A., Strauss, M. E., and McCormick, R. A. (1995). Testing a tripartite model: I. Evaluating the convergent and discriminant validity of anxiety and depression symptom scales. J. Abnorm. Psychol. 104, 3–14. doi: 10.1037/0021-843x.104.1.3
Wearden, J. H., Edwards, H., Fakhri, M., and Percival, A. (1998). Why “sounds are judged longer than lights”: application of a model of the internal clock in humans. Q. J. Exp. Psychol. B 51, 97–120.
PubMed Abstract | Google Scholar
Wearden, J., O’Rourke, S. C., Matchwick, C., Min, Z., and Maeers, S. (2010). Task switching and subjective duration. Q. J. Exp. Psychol. 63, 531–543. doi: 10.1080/17470210903024768
Wearden, J. H., and Penton-Voak, I. S. (1995). Feeling the heat: body temperature and the rate of subjective time, revisited. Q. J. Exp. Psychol. B 48, 129–141.
Weiss, S., Sack, M., Henningsen, P., and Pollatos, O. (2014). On the interaction of self-regulation, interoception and pain perception. Psychopathology 47, 377–382. doi: 10.1159/000365107
Wessberg, J., Olausson, H., Fernström, K. W., and Vallbo, A. B. (2003). Receptive field properties of unmyelinated tactile afferents in the human skin. J. Neurophysiol. 89, 1567–1575. doi: 10.1152/jn.00256.2002
Whyman, A. D., and Moos, R. H. (1967). Time perception and anxiety. Percept. Mot. Skills 24, 567–570. doi: 10.2466/pms.1967.24.2.567
Wiebking, C., de Greck, M., Duncan, N. W., Tempelmann, C., Bajbouj, M., and Northoff, G. (2015). Interoception in insula subregions as a possible state marker for depression-an exploratory fMRI study investigating healthy, depressed and remitted participants. Front. Behav. Neurosci. 9:82. doi: 10.3389/fnbeh.2015.00082
Wiech, K., Lin, C. S., Brodersen, K. H., Bingel, U., Ploner, M., and Tracey, I. (2010). Anterior insula integrates information about salience into perceptual decisions about pain. J. Neurosci. 30, 16324–16331. doi: 10.1523/JNEUROSCI.2087-10.2010
Wierenga, C. E., Bischoff-Grethe, A., Melrose, A. J., Irvine, Z., Torres, L., Bailer, U. F., et al. (2015). Hunger does not motivate reward in women remitted from anorexia nervosa. Biol. Psychiatry 77, 642–652. doi: 10.1016/j.biopsych.2014.09.024
Wittmann, M. (2009). The inner experience of time. Philos. Trans. R. Soc. Lond. B Biol. Sci. 364, 1955–1967. doi: 10.1098/rstb.2009.0003
Wittmann, M. (2014). “Embodied time: the experience of time, the body, and the self,” in Subjective Time: The Philosophy, Psychology, and Neuroscience of Temporality , eds V. Arstila and D. Lloyd (Cambridge, MA: MIT press), 507–523.
Wittmann, M., and Paulus, M. P. (2008). Decision making, impulsivity and time perception. Trends Cogn. Sci. 12, 7–12. doi: 10.1016/j.tics.2007.10.004
Wittmann, M., Simmons, A. N., Aron, J. L., and Paulus, M. P. (2010). Accumulation of neural activity in the posterior insula encodes the passage of time. Neuropsychologia 48, 3110–3120. doi: 10.1016/j.neuropsychologia.2010.06.023
Wittmann, M., and van Wassenhove, V. (2009). The experience of time: neural mechanisms and the interplay of emotion, cognition and embodiment. Philos. Trans. R. Soc. Lond. B Biol. Sci. 364, 1809–1813. doi: 10.1098/rstb.2009.0025
Wylie, K. P., and Tregellas, J. R. (2010). The role of the insula in schizophrenia. Schizophr. Res. 123, 93–104. doi: 10.1016/j.schres.2010.08.027
Yoo, J. Y., and Lee, J. H. (2015). The effects of valence and arousal on time perception in individuals with social anxiety. Front. Psychol. 6:1208. doi: 10.3389/fpsyg.2015.01208
Zakay, D., and Block, R. A. (1996). The role of attention in time estimation processes. Adv. Psychol. 115, 143–164. doi: 10.1016/s0166-4115(96)80057-4
Keywords: interoception, interoceptive buffer, C-T fibers, time perception
Citation: Di Lernia D, Serino S, Pezzulo G, Pedroli E, Cipresso P and Riva G (2018) Feel the Time. Time Perception as a Function of Interoceptive Processing. Front. Hum. Neurosci. 12:74. doi: 10.3389/fnhum.2018.00074
Received: 28 October 2017; Accepted: 12 February 2018; Published: 06 March 2018.
Reviewed by:
Copyright © 2018 Di Lernia, Serino, Pezzulo, Pedroli, Cipresso and Riva. This is an open-access article distributed under the terms of the Creative Commons Attribution License (CC BY) . The use, distribution or reproduction in other forums is permitted, provided the original author(s) and the copyright owner are credited and that the original publication in this journal is cited, in accordance with accepted academic practice. No use, distribution or reproduction is permitted which does not comply with these terms.
*Correspondence: Daniele Di Lernia, [email protected]
Disclaimer: All claims expressed in this article are solely those of the authors and do not necessarily represent those of their affiliated organizations, or those of the publisher, the editors and the reviewers. Any product that may be evaluated in this article or claim that may be made by its manufacturer is not guaranteed or endorsed by the publisher.
The Illusions of Time
Philosophical and Psychological Essays on Timing and Time Perception
- © 2019
- Valtteri Arstila 0 ,
- Adrian Bardon 1 ,
- Sean Enda Power 2 ,
- Argiro Vatakis 3
University of Turku, Turku, Finland
You can also search for this editor in PubMed Google Scholar
Wake Forest University, Winston-Salem, USA
University college cork, cork, ireland, panteion university of social and political sciences, athens, greece.
- Offers an interdisciplinary examination of our perception of time through the study of temporal illusions
- Demonstrates how valuable studies on timing and time perception are to furthering research on cognition and perception
- Explores the role cognition plays in explaining temporal illusions
14k Accesses
44 Altmetric
This is a preview of subscription content, log in via an institution to check access.
Access this book
Subscribe and save.
- Get 10 units per month
- Download Article/Chapter or eBook
- 1 Unit = 1 Article or 1 Chapter
- Cancel anytime
- Available as EPUB and PDF
- Read on any device
- Instant download
- Own it forever
- Compact, lightweight edition
- Dispatched in 3 to 5 business days
- Free shipping worldwide - see info
- Durable hardcover edition
Tax calculation will be finalised at checkout
Other ways to access
Licence this eBook for your library
Institutional subscriptions
About this book
Similar content being viewed by others.
Introduction: Time in Philosophy and Psychology

Temporal Synechism: A Peircean Philosophy of Time
The time of experience and the experience of time.
- consciousness
- temporal order
- time perception
Table of contents (18 chapters)
Front matter, the passage of time, one thing after another: why the passage of time is not an illusion.
- Natalja Deng
Does It Really Seem to Us as Though Time Passes?
- Kristie Miller
The Dynamic Block Universe and the Illusion of Passage
- Maria Balcells
The Perception of Duration and the Judgment of the Passage of Time
- Luke A. Jones
The Temporal Oddball Effect and Related Phenomena: Cognitive Mechanisms and Experimental Approaches
- Rolf Ulrich, Karin M. Bausenhart
Why the Intrinsic Value of Hedonic Sensations Is Not Quantifiable
- Ingmar Persson
The Temporal Dynamic of Emotion Effects on Judgment of Durations
- Sylvie Droit-Volet
Hidden Duration: Time-Lag in the World and Mind
- Kristoffer Sundberg
Modulations in the Experience of Duration
- Marc Wittmann, Tijana Jokic, Eric Pfeifer
Against Illusions of Duration
Sean Enda Power
Simultaneity and Temporal Order
Causality guides time perception.
- Andrea Desantis, Marc Buehner
Getting Stuck in the Ordered Sequence: Disrupted Temporal Processing in Patients with Schizophrenia and What It Tells Us About the Sense of Time Continuity
- Anne Giersch
When the Perception of a Synchronous World Is—Mostly—Just an Illusion
- Nadia Paraskevoudi, Argiro Vatakis
Cognition and Representation of Temporal Phenomena
Time opined: a being in the moment.
- Mark A. Elliott
Temporal Binding and the Perception/Cognition Boundary
- Christoph Hoerl
“The book The Illusions of Time is very well-structured and this structure helps understanding enormously. … Even if you are not a psychologist, neuroscientist or philosopher, all the contributions herein are understandable; the illustrations are particularly illuminating. … It is certainly not intended to be a conclusion, but rather an inventory of what certain sciences know at present. That is exactly what the book is good for, to question what we take for granted when talking about ‘time.’” (Kerstin Cuhls, Kronoscope, Issue 21, 2021)
Editors and Affiliations
Valtteri Arstila
Adrian Bardon
Argiro Vatakis
About the editors
Valtteri Arstila is a Collegium Researcher at the Turku Institute for Advanced Studies and the Department of Philosophy, University of Turku, Finland. His research focuses on the philosophy and psychology of subjective time, time consciousness, cognitive penetration, and artificial intelligence.
Adrian Bardon is Professor of Philosophy at Wake Forest University, USA. He is the author of A Brief History of the Philosophy of Time (2013) and The Truth about Denial: Bias and Self-Deception in Science, Politics, and Religion (2019).
Sean Power is a philosopher researching time, illusion, perception, and epistemology. He is a research affiliate at the University College Cork, Ireland, whose works include Philosophy of Time and Perceptual Experience (2018) and Philosophy of Time: A Contemporary Introduction (forthcoming).
Argiro Vatakis is Assistant Professor at the Psychology Department, Panteion University of Social and Political Sciences, Greece. She is the Editor-In-Chief for the journal Timing and Time Perception , and the founder of the Timing Research Forum.
Bibliographic Information
Book Title : The Illusions of Time
Book Subtitle : Philosophical and Psychological Essays on Timing and Time Perception
Editors : Valtteri Arstila, Adrian Bardon, Sean Enda Power, Argiro Vatakis
DOI : https://doi.org/10.1007/978-3-030-22048-8
Publisher : Palgrave Macmillan Cham
eBook Packages : Religion and Philosophy , Philosophy and Religion (R0)
Copyright Information : The Editor(s) (if applicable) and The Author(s), under exclusive licence to Springer Nature Switzerland AG 2019
Hardcover ISBN : 978-3-030-22047-1 Published: 08 October 2019
Softcover ISBN : 978-3-030-22050-1 Published: 08 October 2020
eBook ISBN : 978-3-030-22048-8 Published: 26 September 2019
Edition Number : 1
Number of Pages : XXVIII, 374
Number of Illustrations : 21 b/w illustrations
Topics : Philosophy of Science , Philosophy of Mind , Metaphysics
- Publish with us
Policies and ethics
- Find a journal
- Track your research
- Search Menu
- Sign in through your institution
- Browse content in Arts and Humanities
- Browse content in Archaeology
- Archaeological Methodology and Techniques
- Biblical Archaeology
- Environmental Archaeology
- Historical Archaeology
- Landscape Archaeology
- Prehistoric Archaeology
- Browse content in Art
- History of Art
- Browse content in Classical Studies
- Classical History
- Classical Literature
- Greek and Roman Epigraphy
- Greek and Roman Archaeology
- Late Antiquity
- Religion in the Ancient World
- Browse content in History
- Colonialism and Imperialism
- Diplomatic History
- Historical Geography
- History by Period
- History of Gender and Sexuality
- Intellectual History
- Legal and Constitutional History
- Local and Family History
- Military History
- Political History
- Regional and National History
- Revolutions and Rebellions
- Slavery and Abolition of Slavery
- Social and Cultural History
- Theory, Methods, and Historiography
- World History
- Language Teaching and Learning
- Browse content in Linguistics
- Grammar, Syntax and Morphology
- Historical and Diachronic Linguistics
- Language Reference
- Language Variation
- Language Families
- Linguistic Anthropology
- Linguistic Theories
- Linguistic Typology
- Sociolinguistics
- Translation and Interpretation
- Browse content in Literature
- Literary Studies (European)
- Literary Studies - World
- Literary Studies (1500 to 1800)
- Literary Studies (19th Century)
- Literary Studies (20th Century onwards)
- Literary Studies (British and Irish)
- Literary Studies (Early and Medieval)
- Literary Studies (Fiction, Novelists, and Prose Writers)
- Literary Studies (Poetry and Poets)
- Literary Studies (Travel Literature)
- Literary Studies (Women's Writing)
- Literary Theory and Cultural Studies
- Mythology and Folklore
- Browse content in Media Studies
- Browse content in Music
- Ethnomusicology
- Music Cultures
- Music and Culture
- Music Theory and Analysis
- Musical Structures, Styles, and Techniques
- Musicology and Music History
- Browse content in Philosophy
- History of Western Philosophy
- Metaphysics
- Moral Philosophy
- Philosophy of Mind
- Philosophy of Science
- Philosophy of Law
- Philosophy of Religion
- Philosophy of Mathematics and Logic
- Social and Political Philosophy
- Browse content in Religion
- Biblical Studies
- Christianity
- History of Religion
- Religion and Education
- Religion and Art, Literature, and Music
- Religious Studies
- Browse content in Society and Culture
- Cultural Studies
- Visual Culture
- Browse content in Law
- Constitutional and Administrative Law
- Criminal Law
- Environment and Energy Law
- History of Law
- Human Rights and Immigration
- Browse content in International Law
- Public International Law
- Legal System and Practice
- Browse content in Medicine and Health
- Public Health and Epidemiology
- Browse content in Science and Mathematics
- Browse content in Psychology
- Cognitive Psychology
- Evolutionary Psychology
- Social Psychology
- Browse content in Social Sciences
- Browse content in Anthropology
- Regional Anthropology
- Social and Cultural Anthropology
- Criminology and Criminal Justice
- Browse content in Economics
- Economic History
- Economic Development and Growth
- Labour and Demographic Economics
- Browse content in Education
- Educational Strategies and Policy
- Browse content in Human Geography
- Political Geography
- Browse content in Politics
- Comparative Politics
- Conflict Politics
- Environmental Politics
- Gender and Politics
- International Relations
- Political Economy
- Political Methodology
- Political Philosophy
- Political Sociology
- Political Theory
- UK Politics
- Browse content in Sociology
- Health, Illness, and Medicine
- Migration Studies
- Occupations, Professions, and Work
- Organizations
- Population and Demography
- Race and Ethnicity
- Social Movements and Social Change
- Social Research and Statistics
- Social Stratification, Inequality, and Mobility
- Sociology of Education
- Urban and Rural Studies
- Journals on Oxford Academic
- Books on Oxford Academic

- < Previous chapter
9. The Perception of Time: Philosophical Views and Psychological Evidence
- Published: March 2006
- Cite Icon Cite
- Permissions Icon Permissions
This chapter aims to present a psychological model of how people perceive time, and to explain some experimental evidence supporting it. It suggests that the apparently close correspondence between the spatiotemporal structures of the perceived world and of the physical world, in contrast to the complex and controversial relation between, for example, perceived colour and light, lies at the root of the primary quality/secondary quality distinction. It also examines the neural mechanisms by which people keep track of time. The answer to this question is a model in which the nervous system itself produces temporal information, in the form of a ‘pacemaker’ or pacemakers that emit pulses at regular characteristic intervals; the model also includes a ‘calibration unit’ to allow for flexibility in the accuracy of timing. The chapter ends by surveying the experimental evidence for this model, including EEG studies.
1. Philosophical views of time perception
Whenever I conceive of any material or corporeal substance, I am necessarily constrained to conceive of that substance as bounded and as possessing this or that shape,…as in this or that place during this or that time, as in motion or at rest…as being one, many, or few—and [I cannot] conceive of any corporeal body apart from these conditions. But I do not at all feel myself compelled to conceive of bodies as necessarily…being red or white, bitter or sweet, having sound or being mute…these tastes, odors, colors, etc.,…are nothing but mere names for something which resides exclusively in our sensitive body, so that if the perceiving creatures were removed, all of these qualities would be annihilated…But just because we have given special names to these qualities, different from the names we have given to the primary and real properties, we are tempted into believing that the former really and truly exist as well as the latter…I should judge that, if ears, tongues, and noses be taken away, the number, shape, and motion of bodies would remain, but not their tastes, sounds, and odors. (Galileo, 1623 / 1960 , pp. 27–30)
Galileo’s distinction is related to but not identical with that proposed by St Augustine (401/ 1960 ), who distinguished between those images in memory brought in by the senses, ‘for instance, light, color, and the shapes of bodies reach us through the eyes’ (p. 217), and ‘the innumerable principles and laws of numbers and dimensions [which are not] imprinted on it by any bodily sense, since none of these is colored or can be heard…’ (p. 222).
A distinction between two types of experience or knowledge has continued to exercise philosophers (for example Ayer, 1956 ; Quinton, 1973 ) but the light that this distinction has cast has fallen differently for different thinkers. For Galileo the primary qualities were necessary apprehensions of essential aspects of physical reality. For Locke ( 1690 / 1947 ) and Hume ( 1739 / 1911 ) our knowledge of those qualities could have no source other than the senses. For Kant ( 1787 / 1934 ) our appreciations of space and time are ‘pure intuitions’ which provide necessary conditions for the perception of a physical world of whose reality they do not form part.
The interest in this distinction is closely related to that in another perennial problem, the relation between phenomenological sensation and the physical world. We accept, for example, that the colours we experience ourselves as seeing (the red of the rose) relate, but not in any simple way, to the world that physics describes (the spectrum of reflected sunlight). But it is easy to imagine that the dimensions of space and time that frame perceptual experience and may be conveyed by different modalities of sensation evade this difficulty, and that they feature in essentially the same way as structural aspects of perception and of the physical world. We may make mistakes in perceiving spatial and temporal relations; illusions occur; but it is felt that in the main these dimensions as given in perception directly reproduce the same dimensions as occur in physical reality. Naive realism, defeated on the field of colour, remains upright on the plane of time and space.
The problems of phenomenology will not be resolved here. Past beliefs about the mechanisms of sensation determined corresponding views of experience, such as that ‘singly imperceptible bodies’ carried from objects by the senses produced ideas of them in the mind (Locke, 1690 / 1947 ), and that simple models for the aggregation of ideas or images or sense data accounted for the perceived world. Such hypotheses have been tenacious. ‘Out of the multitude of our sense experiences, we take…certain repeatedly occurring complexes of sense impressions [and] we attribute to them a meaning—the meaning of the bodily object’ (Einstein, 1936 , p. 350).
A more contemporary approach lays aside the unsolved, perhaps insoluble, phenomenological problems that terms such as ‘sense data’ refer to and focuses instead on understanding the relations between the descriptions we give of the outside world and the discriminations we make, and the information that world provides. We now appreciate that the information embodied in perception does not simply depict the physical world, but reflects the interaction of that world with the brain and sense organs. Thus there is no simple relation between the colours we see and the unidimensional spectrum of light. Three biological systems, differently sensitive to light frequency, create a representation of each locus in the visual field in terms of three colour dimensions. Furthermore, what is encoded is not determined solely by the light from an individual locus but is a function of that light and of light coming from elsewhere in the visual field.
It is also too simple to suppose that secondary but not primary qualities are given by one sense only (Quinton, 1973 ), even if it were evident why such a claim deserved to carry metaphysical weight. The sounds we hear when we listen to a word may be determined not only by the auditory system but also by visual information (McGurk and MacDonald, 1976 ). It is more useful to think of the different sensory modalities as constituting a cooperative biological system that interacts with our physical surroundings to determine not a collection of agglutinated sense data but the world as we perceive it—a world not only of objects but also having a four-dimensional spatial and temporal structure in which they are embedded.
How is it that the spatiotemporal structure of the perceived world appears to match that of the physical world so closely? The contrast between this apparently transparent relationship and the complex relation between, for example, perceived colour and light lies at the root of the primary quality-secondary quality distinction. Why have conceptions of space and time abstracted from perception served so well as a starting point for physics? Two answers can be offered. The first is a variant of the anthropic principle: the organism that perceives evolved to survive in the world that exists. Survival requires that predictions can be made and actions planned that achieve favourable consequences. If the relations embodied in the perceptual spatiotemporal framework are useful for such purposes, then abstracting those relations as part of an initial description of the physical world should prove useful too. Since evolution has favoured a perceptual model with three dimensions of space and one of time, the projection of this model on to the external world should provide a useful approximate description of it.
The second answer lies in our propensity to confuse similar things. If the same terms are used to refer to aspects of perception and of the physical world, it is easy to assume those terms have a single reference, and overlook differences. Thus a confusion between what is perceived and what is believed leads to the impression that perceived time and space are identical with the time and space of the physical world. Consequently, the world people commonly believe they experience is not restricted to what is given by perception, but merges this with the idealized version that serves as the basis for naïve physics. The resulting conception is that expressed by St Augustine (401/ 1960 ), who describes time as a linear sequence divided into past, present and future. The past and future do not exist, and the present ‘is a point of time so small that it cannot be divided into even the most minute particles of moments…Such a time must fly so rapidly from future to past that it has no duration and no extension’ (p. 269). A more explicit identification of the characteristics of perceived and physical time is offered by Einstein ( 1936 , p. 354): An important property of our sense experiences…is its time-like order. This…leads to the mental conception of a subjective time [and this] leads then through the concept of the bodily object and of space, to the concept of objective time.’ So the ‘subjective’, a word usually used dismissively, is offered as a basis for our description of the physical world.
While St Augustine’s (401/ 1960 ) and Newton’s ( 1689 / 1960 ) conception of time may serve for everyday physics, it has not proved adequate for fundamental physics. But neither does it supply a description of our actual perception of space and time. We do not experience ourselves as phenomenologically located at a point in an infinite homogeneous space extending equally in all directions. Rather we perceive ourselves as contained in a bubble of space whose extent is delimited by our viewpoint and the reach of our senses, and which moves and adjusts itself with us as we peregrinate through our personal worlds. But we believe in Newtonian space, and in the service of this belief most people (not being perceptual psychologists) fail to notice the extent to which our perceptions depart from it. We believe objects remain the same size wherever they may be whereas, as they move away from us, we see them maintain their size to a limiting distance, after which they start to dwindle. But if we are asked to consider this, it is dismissed as familiar and illusory, ‘subjective’; and a pragmatic belief in the identity of perceived and physical space is maintained.
It is common to distinguish between perception and cognition as though the former is limited to the phenomenology of the moment and the latter is a repository of abstract knowledge. This sharp distinction is misleading. The world we experience ourselves as inhabiting is not limited to what is momentarily given by our senses. It is shaped also by what we have recently perceived, by expectations, knowledge and memory, to provide an adequate arena in which to plan and act. The vaguely bounded bubble of space we inhabit extends not only from our faces forward but also backwards, behind us. The guest entering a room sees her hosts before her but remains aware of the location of the door behind: at the cry of ‘Fire!’ she will know where to dash. Current perception and the fruits of past perceptions form a seamless whole, together allowing us to negotiate the circumambient world.
What of time in this bubble? Though we may believe, with St Augustine, that what we perceive conforms to a physical model in which the present balances on a knife-edge of no extension, separating past and future, if one attentively examines one’s perception of the present moment this is not what one finds. As Clay (quoted by James, 1890 / 1950 ) puts it, the present
is really a part of the past—a recent past—delusively given as being a time that intervenes between the past and the future. Let it be named the specious present…All the notes of a bar of song seem to the listener to be contained in the present. All the changes of place of a meteor seem to the beholder to be contained in the present. At the instant of the termination of such series, no part of the time measured by them seems to be a past.
‘In short’, James adds, it ‘is no knife-edge, but a saddle-back,…from which we look in two directions into time (p. 609)…the short duration of which we are immediately and incessantly sensible’ (p. 631).
This would seem to be the temporal dimension of the bubble of immediate experience, a unit of perceived time which includes together as one the events of a short duration without compromising our impressions of their relations of simultaneity or successiveness. James considered it to correspond to a few seconds, but this is a very short period for following the evolution of events or planning actions in time. It restricts us too unreflectingly to what appears to be immediately phenomenologically given. If we divert our minds from the intriguing immediacy of those few seconds and consider also the range of temporally extended knowledge kept in mind and drawn upon in planning our course through the world, the picture is more complex. Just as the world we experience includes the space behind as well as the space before, borne up also by implicit knowledge of where the room is in the building and the building in the town, so it includes and orients us in relation to a series of overlapping durations of which we are continuously aware: the immediate moment, containing perhaps the phrase a lecturer is uttering; an awareness of the duration of her current argument and where she is in it; an awareness of the length of the lecture and how far into it we are; of the length of the day, and where the lecture lies in that expanse; of how far ahead dinner is and when we must leave not to miss it.
If we avoid imposing a sharp distinction between the phenomenologically given present moment and those longer durations of which we are or can be simultaneously aware, it may appear that our orientation in time is organized in parallel units which differ in extent and scale. The present minute and the present hour are unified by sharing the contents of the same present moment and some past moments. It is in this sense that they overlap. Extending this notion, our experience of time may suggest that we possess a set of overlapping ‘time receptors’, mechanisms that count out the events of a day, an hour, or a minute (and other intervals). To review or to orient ourselves in relation to a longer period of recent time we would draw on a longer-term temporal mechanism of greater reach but less precision; to focus on a lesser interval we would employ a shorter-term mechanism. Such a continuum of receptors provides a possible basis for understanding how it is that, of all the times and events which we have experienced and that live on in our memory, the present moment gains its unique and unchallengeable status. It is distinguished from other moments by the conjoint activity of time receptors possessing the whole range of temporal spans. Similarly, the events of a minute ago are marked by the associated activation of temporal mechanisms that process the events of a minute or longer, and the absence of those for shorter ranges. As an event recedes into the past, its representation would be associated with increasingly long-term ‘time receptors’ only. Eventually the event becomes historic and other more inferential mechanisms must be called on to place it in time.
These speculations serve to illustrate that the direct relation so often posited between primary qualities and corresponding features of the physical world may be too simple; just as with secondary qualities, the brain may implement a neural coding scheme to extract temporal information from its encounters with the presumed single linear dimension of physical time.
The senses provide us not with a picture of the external world but with a model of it. They serve not to achieve verisimilitude (whatever that might be) but to facilitate our interactions with it. Considering the range and nature of our abilities, we may suspect that the infant is equipped to acquire knowledge of space and time just as it is equipped to perceive colour or acquire language. Particular words must be learned, but the ability to acquire both those words and the rules for using them has been embodied in the nervous system during the course of evolution. Similarly, we may assume that our brains have been shaped to structure perception in a spatiotemporal framework. Not only colours and sounds, but also areas and durations contribute to the totality of our model of the physical world. If the perception of time is based on inbuilt mechanisms, the problem of identifying them arises. The three-dimensional nature of colour derives from the visual colour-coding system, not from the physics of light. Similarly, the features of psychological time that differ from those of physical time should have their origins in the neural mechanisms that process temporal information. So, as regards time, the task of experimental psychology is to offer an account of its biological basis that provides an understanding of our experience of time and our abilities to act in time.
2. The Calibrated Pacemaker: a Model of Psychological Time
The two main traditional views of time are mirrored by two corresponding psychological approaches. One view considers temporal judgements to be cognitive inferences based on sensory and other cues. Cues may be used to judge time just as we may use cues to judge distance. Time judgements may be guided by the silence of the early hours, cocks crowing, or the height of the sun. The alternative view proposes that our perception of time derives largely from the operation of specialized neural mechanisms that form a temporal sensory system. This can place us in time just as the vestibular system orients us in space. I shall look at some evidence for the latter position.
Time perception is constrained by the needs it must serve. One of the most important is the need for accurate prediction: perception must allow us to foresee the consequences of action. But a model of psychological time must explain not only more or less veridical perception, but also phenomena that reflect not the nature of the external world but the neural mechanisms that represent it. Such phenomena are easily found; for example, the common variations in apparent duration. Most people would agree that monotonous, repetitive tasks make time drag while interesting work makes it pass rapidly. Kerr and Keil ( 1963 ) tested this expectation by introducing a mechanic into a Chicago factory, where he ostentatiously worked on the clocks. Employees were then told the times had been altered and were asked to estimate how fast or slow the clocks now were. Contrary to the expectation, workers with dull jobs thought it was early; workers with varied jobs thought it was late. Isolation also causes large errors in the perception of time. Siffre spent two months in solitary boredom in an underground cave, a situation one might think would make time seem endless. But despite maintaining an approximately 24-hour sleep rhythm, at the end of 61 days he thought that only 36 days had passed. He said, ‘What threw me off in my estimation of the time spent underground was that I underestimated by almost half the length of my…waking hours; a “day” that I estimated as seven hours actually lasted…fourteen hours and forty minutes’ (Siffre, 1965 ).
A sensory system is designed to process incoming information. The eye or ear responds to energy from the environment. There are no equivalent inputs for short intervals of time. Hoagland ( 1933 ) proposed that time perception depends on an internal biochemical clock. He noted: ‘My wife, having fallen ill with influenza…was asked to count 60 seconds to herself at what she believed to be a rate of one per second. Simultaneously the actual duration of the count was observed with a stopwatch.’ As her temperature rose he found that her subjective estimate of a minute became shorter. Moreover, she was unaware that her counting rate had altered. Hoagland proposed that this illustrated the operation of a chemical clock whose rate increased as its temperature rose.
There are problems with this proposal. A rise in body temperature that makes an internal mechanism run faster might cause a fixed clock interval to seem longer or a subject to count off a minute more quickly. But changes in estimation may occur that are larger than can be accounted for by the effects of temperature on biochemical processes. Changes in body temperature would not account for the effects of factors such as occupation or isolation. Anticipation of an electric shock (Falk and Bindra, 1954 ) or apprehension of danger—being wheeled blindfolded towards a stairwell (Langer, Wapner and Werner, 1961 )—cause shortened time productions. (The subjects depressed a button and held it down for what they judged to be 15 seconds or 5 seconds respectively.) As we might expect, Lockhart ( 1967 ) found that time productions were shortened when subjects spent an hour in their underwear at 43°C, but they were also shortened by an hour spent at 4°C, as compared with an hour at 27°C.
To account for such effects, an information-processing model of the mechanisms underlying timing was put forward by Treisman ( 1963 : see Figure 9.1 ). Temporal information originates in a pacemaker that emits pulses at a regular, characteristic rate. The internal clock has additional components that apply this information to temporal tasks. If, for example, a subject wishes to measure the length of the interval between two signals, the counter will switch on when the first signal comes and record pacemaker pulses until the second signal arrives. The number of pulses counted in this interval is stored in a memory store, and can be used to determine an appropriate verbal estimate of the duration. If the subject wishes to reproduce a previously presented duration, a signal will initiate the reproduction and the subject will emit a second signal when she thinks the required interval has elapsed. To allow this, the counter will commence recording pulses at the initial signal, the comparator will continually compare the number cumulated with the count previously stored, and when the two numbers approach equality the subject will produce the final signal.
An information-processing model for time perception
In this model, the pacemaker is a biological mechanism which runs more quickly if its temperature is raised, or if its activation is increased by arousing inputs. These could be generated centrally or by strong external stimuli. Higher activation causes the rate at which pulses are emitted to rise. So an interval may be judged longer (more pulses are counted during its presentation) if body temperature rises or if loud noises or discomfort activate the pacemaker. The arousal produced by shivering in a cold room will outweigh any reduction in core body temperature and so make the interval seem longer. (But if body temperature is reduced by the pleasant chore of consuming sufficient ice cream, the pacemaker slows and intervals seem shorter.)
While this model can account for some results, it is still too simple. For example, everyday observation tells us that the stability of temporal judgements may differ for different tasks. If the music is dull, the hours spent listening to the concert seem to drag, whereas exciting music hastens them along. But the orchestra plays the music at the same rate in each case, and listeners seem able to assess its tempo in much the same way.
Before formulating a more complex model, we need to clarify what needs it must serve. The information-processing model explains the perception of duration. But we not only perceive time, we act in time as well. To act effectively, our speed of movement must be coordinated with the temporal evolution of the events we are responding to. The player must not only judge the time the ball will take to reach him; he must also move his arm at the right speed if he is to catch it. What capacities must a temporal pacemaker have if it is to be useful in controlling motor action?
One way to organize the control of timing would be for a single master clock to pace all movement. But this would be unacceptably restrictive: it would be difficult to move two limbs simultaneously at different rates, or to change their relative speeds smoothly. Damage to the master clock would disrupt all aspects of timing simultaneously, not a syndrome which is seen. Alternatively, just as we coordinate social activities by carrying synchronized watches, so physical actions might be coordinated by multiple pacemakers distributed at different levels of the nervous system and controlling different limbs, with these pacemakers set to run at similar rates. The central motor programme that controls a complex action, such as playing the piano, would issue instructions to different levels of a control hierarchy. These might specify the sequence and relative durations of movements, but their detailed production and timing would be implemented by pacemakers residing at different levels in the neural hierarchy, controlling the arm, for example, or the fingers. If the pacemakers run at similar rates, this would allow performance to be temporally coordinated.
While such a model answers one question, it creates others. Although distributed pacemakers running at the same rate provide a basis for coordination, they also present a problem for coordination. Each time a performance must be repeated at a different speed, as in dancing, or if the rate at which one limb moves relative to another must be altered, the central motor programme would need to recalculate its set of parametric durations. As skilled performance depends on the sensitive and flexible adjustment of the tempo at which we act, this would be a major difficulty. To alter the pace of action while preserving coordination, it would be more efficient to leave the programme unchanged and instead speed or slow pacemakers in the same proportion. In this way a runner could speed from slow to fast without losing coordination. But this requires that clock rates can be flexibly adjusted.
So the real world presents opposed requirements: the temporal pacemaker must provide a stable reference frequency; and it must produce an output frequency that can be flexibly modulated. Unless it can do both, the timing of action cannot be both accurate and flexible. It is also parsimonious, in relating action to perception, to assume that the sources of information used in judging time and in controlling the speed of movements are similar. The coupling between perceiving durations and acting in time requires that similar pacemakers mediate both, whether in pursuing prey, catching a ball, or driving a car.
To meet these requirements the calibrated pacemaker model, an expanded model for the generation of the temporal frequency, was developed (Treisman et al., 1990 : see Figure 9.2 ). This has two components: first, a non-linear temporal oscillator (TO) that emits pulses at a stable rate, the characteristic oscillator frequency; second, a calibration unit (CU). Pulses from the temporal oscillator go to the calibration unit, and this in turn emits a series of pulses, the final pacemaker output, whose frequency is given by multiplying the TO output by a calibration factor. The value of the calibration factor can be adjusted; it increases with the activation of the CU. The final pacemaker output from the CU is employed by the clock’s information-processing mechanisms to perform temporal tasks such as estimating durations. If especially high stability is required, when conducting an orchestra, perhaps, the stable output from the TO might be used directly.
Note: The model consists of a temporal oscillator (TO) and a calibration unit (CU). The TO is made up of elementary units linked by inhibitory or excitatory connections. This system emits regular pulses at an oscillator frequency, F o . These go to the CU. This emits the final pacemaker output, F p , that provides timing information for the temporal processing mechanisms. F p is a function of F o .
The calibrated temporal pacemaker model
The temporal oscillator is stable. The calibration unit allows flexibility of timing. Messages from the centre, or strong stimuli, can act on each CU (via the channel labelled S in the figure) to modify its activation. Such messages can act in parallel on all or a set of calibration units, so that the final pacemaker outputs all speed up or slow down in the same way or related ways. Thus the dancer can smoothly increase his speed or the runner her sprint.
The model can be examined by testing its predictions. The effects of temperature, or of arousing stimuli acting on the CU, give predictions similar to those discussed above. As the TO is non-linear, the properties of non-linear oscillators predict its behaviour. Two properties are important. First, an oscillator may be excited into oscillation or entrained by an external frequency that is close to its characteristic frequency. This causes it to take on the applied frequency. Second, if the two frequencies are not the same or nearly the same but the ratio between them is close to a simple integral relation, such as 1:2 or 2:3, the external frequency may entrain the oscillator.
This suggests a prediction that can be used to test the model. A time interval is presented to be judged, and during its presentation an external frequency is imposed on the system. If this frequency is just below the characteristic temporal oscillator output it may entrain the oscillator and slow it down. If so, the counter will count fewer final output pulses in a given time, and the estimated duration of the interval will be reduced. An applied frequency just above the characteristic oscillator frequency will have the opposite effect. This gives a biphasic perturbation of the TO output, a decrease below the resting level, followed by a rise above it, as the applied frequency increases over some small range. Similar perturbations will be produced by applied frequencies which are close to standing in simple integral relations to the TO frequency.
Figure 9.3 illustrates a computer simulation of the calibrated pacemaker model. The upper panel shows the TO output frequency (F o ) as a function of different applied frequencies (F A ) . The dashed line is the characteristic TO frequency, F c,o = 1, that is emitted at rest. The continuous line shows the TO output frequency F o when applied frequencies are presented. The applied frequency F A = 1 has no effect as it is identical with F c,o = 1 Applied frequencies slightly below this cause the oscillator frequency to fall; slightly higher applied frequencies cause it to rise. Thus we have a perturbation consisting of a dip and a peak in the vicinity of F c,o
Note: Upper panel: the TO output, F o , is plotted for different rates F A of an applied pulse input train (continuous line). The characteristic TO output frequency, in the absence of an applied frequency (1.00 in this case), is shown as a dashed line. Lower panel: the corresponding CU output, F p , is plotted against F A . This is here given by F p = C f F o = (5 + F A )F o . C f , the calibration factor, is a function of F A . Labelled vertical lines indicate applied frequencies that have simple relations to the characteristic TO frequency.
Simulations of the calibrated pacemaker model
Similar but smaller perturbations are seen where the ratio of F A to F c,o is close to 2:3, 1:2, and so on. Such a set of perturbations will be referred to as an ‘interference pattern’.
The upper panel assumes that the calibration factor by which the calibration unit multiplies F o is constant (here equal to one), whatever the applied frequency. The lower panel illustrates the case in which the calibration factor is an increasing linear function of the applied frequency. In this case a rise in F A increases the activation of the calibration unit.
Experimental predictions arise when we transpose these results to behaviour. If observers are asked to estimate a fixed duration while regular auditory pulses are presented to them, at different frequencies on different trials, and if the assumptions of the model are correct and the applied frequency used in the experiment is capable of interfering with the TO, then a plot of the observers’ estimates of the presented interval against F A should show perturbations. If such perturbations occur they should form an interference pattern similar to that in Figure 9.3 . Furthermore, it may be possible to use the pattern obtained to estimate F c,o .
An experiment was run to test these predictions (Treisman et al., 1990 , Experiment 1). On each trial the subject attended to an asterisk presented on a computer screen for a short interval such as 500 milliseconds (ms), and then estimated its duration. The intervals varied over a small range from trial to trial. Each interval was accompanied by a concurrent train of auditory clicks (the applied frequency, F A ) . The clicks were given at rates from 2.5 to 27.5 Hz, spaced at 0.5 Hz. Two sets of durations were intermingled, ‘short’ (S) or ‘long’ (L). Short durations were derived from reference values such as 550 ms (for the higher click rates) or 750 ms (for the lower click rates). For long durations the reference values were 250 ms greater. The duration actually presented on each trial was obtained by adjusting the reference interval so that it coincided with a concurrent series of clicks. For example, if clicks are given at 10 per second then six clicks will coincide with a 500 ms duration. But if the clicks are given at 9.5 per second then six clicks will coincide with a duration of 526 ms.
According to the present theory, an estimate is determined by three factors: the length of the interval presented, the number of clicks presented during that interval—both of these effects are assumed to be monotonic— and the non-linear perturbations. The effects of the first two factors can be estimated by expressing subjects’ time estimates as a bilinear function of the durations presented and the numbers of clicks. The non-linear effects are shown as systematic residual deviations about this regression line. Results analysed in this way are shown in Figure 9.4 . The upper panel gives the mean deviations about the regression lines for all the data. These take the form of alternating dips and peaks in the curve. The arrows, labelled with the corresponding click rates, indicate the positions of the larger dips. The lower panel shows the results separately for short and long durations. The arrows from the top panel are repeated below these two curves. There is considerable agreement between the two cases. For example, the curve for short durations shows noticeable dips (marked by arrowheads) at 9.0, 11.5, 13.5 and 16.0 Hz. For long durations there are dips at the same location or within 0.5 Hz of it. (At the lowest click rates the differences between the curves increase. The largest dip for the short durations is at 4.5 Hz and for the long durations at 3.5 Hz. The same difference was shown by all subjects in this experiment.)
Note: Upper panel: a bilinear regression of time estimates on to presented durations and numbers of clicks was found. Deviations from this fit are plotted against auditory click rate, for all the data. Nine dips are indicated by upward-pointing arrows. Lower panel: mean residuals for short durations and long durations are plotted separately. The arrows from the upper panel are reproduced in the same positions below the S and L curves. The vertical lines indicate click rates having simple relations to the estimated F c,o (24.75 Hz).
(opposite) Experiment 1 (Treisman et al., 1990 )
The pattern of dips may be used to identify possible underlying characteristic frequencies. The present interference pattern could be matched to the patterns given by two candidate F c,o values, 24.75 and 49.5 Hz. A second experiment gave a single candidate frequency: 37.3 Hz. These values are almost exactly twice, thrice and four times 12.4 Hz.
A replication at a later date with a new subject, JM, is shown in Figure 9.5 . The arrows represent the positions of the main dips in the combined data of Experiment 1. They match equivalent dips in the present data, to within 0.5 Hz. The present data were subdivided into observations from the first (1) and second (2) half of each session, for short (S) and long (L) durations. The agreement between the first and second half-sessions shows that performance was stable throughout the course of a session in this subject.
Note: The data are divided into short and long, and in each case data obtained from the first half of each session are plotted separately from data obtained in the second half. The arrows are replotted from the upper panel of Figure 9.4 .
(opposite) A replication of the procedure illustrated in Figure 9.4 , with a new subject
These results show an interference pattern such as is predicted by the non-linear oscillator model. Another way to test the model is to look at the control of movement. In catching a ball or any other action, the need to relate the course of movement to our perception of ongoing events requires that related measures of time are employed for each. If both use similar pacemakers we can predict, first, that it may be possible to see an interference pattern in the timing of motor performance, and, second, that this pattern should be related to the pattern given by time estimation.
Subjects again watched a computer screen on which an asterisk appeared, in this case slightly to the left or right of centre (Treisman, Faulkner and Naish, 1992 ). Auditory clicks were presented from the moment the asterisk appeared until the subject had responded. The subject was to move his arm about 16 inches and press a key indicating the position of the asterisk, as quickly as possible.
If clicks at a given rate slow the TO, fewer pulses are counted, the subject’s estimate is reduced, and we get a dip in the time estimation interference pattern. If a similar pacemaker paces a motor response, the reduction in its rate by the clicks will cause a slower response. Thus a click rate that produces a dip in time estimation should produce a peak in response time. In Figure 9.6 the estimation interference pattern is replotted from the upper panel of Figure 9.4 as a dashed line. Median response times were regressed on to click rates, and the deviations from these regressions are shown as a continuous line, with positive residuals (peaks) plotted downwards, to facilitate comparison with the dips in the time estimation pattern.
Note: Response times were regressed on to click rate, and deviations from the best-fitting regression in ms are plotted. Note that this plot is inverted. The time estimation curve from the upper panel of Figure 9.4 is replotted as a dashed line. Points where dips and peaks coincide are indicated by arrows. If there is a divergence of 0.5 Hz, the arrow is unfilled.
Data from a choice reaction time task (Treisman et al., 1992 ; continuous line)
For identical pacemakers, and in the absence of noise, the motor peaks and dips should match the estimation dips and peaks in location. (No predictions are made for their amplitudes, as these may be affected by other factors.) The filled arrows indicate locations where such a match occurs. Empty arrows indicate a 0.5 Hz disparity. There is a significant degree of agreement between the two interference patterns, which supports the hypothesis that similar pacemakers underlie both tasks. The motor interference pattern estimated the TO characteristic frequency as 49.8 Hz.
3. Timing Mechanisms in the Brain
We have obtained psychophysical evidence for a hypothesis about the mechanisms underlying the timing of short intervals. Our understanding of these mechanisms would be amplified if we could demonstrate the activity of such oscillators in the electroencephalogram (EEG). The advantage of looking at the EEG is that it is a record of oscillatory processes in the brain and these might include the output of a temporal oscillator. The disadvantage is that the EEG may include a great many unrelated oscillations, sufficient to hide any TO output from view. Supposing temporal oscillators contribute to the EEG, and we could see this, what might we expect to see?
Two features of the TO output provide a guide to its EEG signature. We have provisional estimates of its frequency: 24.75, 37.3 and 49.5 Hz; and we believe it is sensitive to clicks at certain rates. Thus if the EEG contains a temporal oscillator producing an output at, say, 24 Hz, and if we could make this TO output visible, and if clicks were presented at rates at which they affect time estimates, we might see the frequency of this EEG oscillation vary in accordance with the time estimation interference pattern. However, even if a click-sensitive TO output oscillation is present in the EEG, and is affected in the predicted way by applied frequencies, this would be difficult to observe. The problem is comparable to trying to detect whether one of the violinists in an orchestra has switched from playing one note of a chord to playing another. One solution is to select a narrow band of EEG frequencies which we expect to include the oscillator output, say 21–25 Hz, and to measure the mean frequency or centroid of this band. if it does include the TO output, and if clicks are given at a rate that reduces its frequency, the mean frequency of the band may be detectably lowered.
A third consideration may affect what we see: the multiplicity of TOs. If timing is based on distributed pacemakers, the nervous system must contain not one but a set of clocks. Since biological quantities vary, it is likely that the TO characteristic frequencies will vary about a mean, giving a distribution of click-sensitive frequencies. Figure 9.7 illustrates some predictions these assumptions lead to, for a hypothetical simplified EEG with arbitrary parameters.
Note: The EEG spectrum consists of a rectangular distribution of non-temporal frequencies, together with a normal distribution of click-sensitive oscillations (mean 98, standard deviation 7.5 Hz). The left panel plots the EEG spectrum against nine click rates, with frequency on the ordinate, and click rate on the abscissa. Clicks at different rates may shift the distribution up or down one or two standard deviation units. The simulated EEG spectrum is partitioned into 5 Hz bands and the centroid frequency of each band is plotted against click rate on the right to give a CF curve. The parameter on each curve is the upper limit of the frequency band. Dashed lines delimit bands 96–100 Hz at click rates 1 and 2, and bands 96–100 Hz, and 101–105 Hz at click rates 5 and 6.
(this page and facing page) A simplified model of the EEG with arbitrary parameters
On the far left, a simplified model of the EEG spectrum is plotted vertically, for frequencies ranging from 50 to 130 Hz (for illustration). The spectrum is assumed to consist of a rectangular distribution of non-click-sensitive frequencies (not shown), combined with a normal distribution of click-sensitive frequencies, represented by a hatched bell-shaped curve with mean 98 and standard deviation 7.5 Hz. This spectrum is plotted against auditory click rate 1, on the abscissa. (These values are arbitrary.) The spectrum is replotted for several higher click rates. At some rates, such as 2 and 6, the TO output frequencies fall, so that the click-sensitive distribution moves downwards. At slightly higher rates, such as 3 and 8, the distribution moves upwards.
The observations that would result are shown in the panel on the right. Here the EEG is analysed in 5 Hz bands, for example, 61–65 Hz (labelled with its upper limit, 65). The centroid frequency (CF) for each band is plotted as a function of click rate. The lowest curve plotted is the CF curve for the frequencies 51–55 Hz (labelled 55). At each click rate the spectrum contains no click-sensitive oscillations between 51 and 55 Hz, and so is unfailingly rectangular, with a centroid frequency of 53 Hz. Thus these points give a horizontal straight line.
The band of frequencies from 96 to 100 Hz gives a more complex CF curve. At click rate 1, the click-sensitive distribution is centred at 98 Hz and is symmetrical (the band is indicated by two dashed lines). The corresponding centroid frequency is plotted against click rate 1 in the right panel (the curve is labelled 100). At click rate 2, TO frequencies are reduced and the click-sensitive distribution moves downward. The section of the distribution between 96 and 100 Hz is no longer symmetrical. The height of the distribution is now greater at the lower end of this range, so that the mean frequency is reduced: thus CF curve 100 shows a dip at click rate 2. For similar reasons, the curve shows a dip at click rate 6, and peaks at 3 and 8. Thus CF curve 100, for the range from 96 to 100 Hz that includes the mean of the click-sensitive distribution (98), traces out an interference pattern similar to that given by time estimation.
The assumption that there is a distribution of click-sensitive frequencies has a further consequence. Curve 105 (for the band 101–105) has a peak at click rate 6 (a vertical dashed line links this to the dip in curve 100). At click rate 5, the section of the distribution between 101 and 105 Hz is greater at the lower end of the range, giving a relatively low CF. As the distribution moves down at 6, the weighting within the band 101–105 Hz becomes more uniform and the CF rises, giving the peak seen on the right. At click rate 6, the peak in curve 105, paired with the dip in curve 100, forms a diamond pattern. Such effects tend to produce a vertical mirror image relation between centroid curves that bracket the mean TO frequency.
This brief analysis leads to the following predictions:
If the brain contains a distribution of click-sensitive TOs with a mean frequency at 24.75 Hz or thereabouts, a sufficiently small band of EEG frequencies (such as 21–25 Hz or less) will give a CF curve that reproduces the time estimation interference pattern.
If so, the CF curve for a higher band (for example, curve 30) may have the form of a partial vertical mirror image of curve 25. The two curves may enclose ‘diamonds’ between them at rates which cause dips in curve 25.
If the click-sensitive distribution is centred near 37.3 Hz, these predictions would apply to CF curves 40 and 45, covering the range 36–45 Hz. If the distribution is centred at 49.5 Hz, the relevant pair of curves would be those labelled 50 and 55, covering the range 46–55 Hz.
In an experimental test of this model, EEGs were recorded while subjects estimated time intervals that were accompanied by clicks at different rates (Treisman et al., 1994 ). Power spectrum analyses were performed on the EEG samples, the results divided into five Hz bands, and CF curves plotted against click rate. Results for one subject are shown in Figure 9.8 .
Note: The CF curves of successive 5 Hz bands are plotted against click rate. The upper limit of the frequency band is shown as the parameter for each centroid frequency curve. Diamond patterns between curves 25 and 30 are indicated by vertical dashed lines. These lines are repeated at the same click rates between curves 40 and 45, and curves 50 and 55.
(opposite) EEG data for subject PN
The earlier findings predict that CF curve 25 should show an interference pattern. This curve has a number of dips at frequencies at which the time estimation interference patterns commonly showed a dip. CF curve 30 presents a vertical mirror image of the 25 Hz pattern. Peaks in curve 30 correspond to major dips in curve 25, so that diamond patterns (indicated by vertical dashed lines) are enclosed between them. This accords with the model’s second prediction.
The second possible mirror-image CF curve pair (curves 50 and 55) is seen, as is the third (curves 40 and 45). In each case, vertical dashed lines are shown at the locations of the diamond patterns enclosed by curves 25 and 30. For the most part, diamonds are seen at the same locations for the different pairs of curves. Furthermore, further such pairs of curves occur (such as 65 and 70, 90 and 95, and higher-valued pairs not shown in this figure). Results for other subjects were similar. In each case, mirror-image pairs of curves appear to recur at intervals of about 12.8 Hz.
The EEG findings are compared with the earlier psychophysical results in Figure 9.9 . The pair of curves 25 and 30 is plotted for three subjects, and time estimation residual curves are shown from three data sets (Experiment 1 of Treisman et al., 1990 , short and long, and time estimation results from the present experiment). The major dips are indicated in each case. There are differences between the various results, but similarities are evident. For example, in five of the six plots, the highest dip shown lies between 16.0 and 16.7 Hz. In five cases there is a dip at 13.5 and in one at 13.9 Hz. In every case there is a dip between 11.1 and 11.6 Hz. Disparities are greater at lower frequencies. In three cases there is a dip at 3.5 and in three at 4.5 Hz.
Note: Dashed vertical lines indicate the locations of diamonds; where complementary peaks and dips differ by about 0.5 Hz, dotted lines are drawn. The dips are indicated by labelled arrows. Short and long time estimation interference patterns from Treisman et al . ( 1990 ) and the mean time estimation interference pattern from the EEG experiment are shown. Certain time estimation dips are labelled.
(next facing pages) CF curves 25 and 30 shown for three subjects
4. Discussion and Conclusion
The studies above (and other work not reviewed here) provide evidence for internal mechanisms enabling us to process relatively short intervals of time. The biological pacemakers that underlie long-term periodicities such as circadian rhythms differ from the internal clocks discussed here in that they are temperature-compensated (Moore-Ede, Sulzman and Fuller, 1982 ; Glass and Mackey, 1988 ).
The evidence not only supports a biological basis for time perception; it also indicates that similar systems may coordinate perception and action in time. The EEG findings suggest further conclusions, of which two are of special interest.
First is the evidence that time-keeping does not depend on a single internal clock. Timing is based on multiple pacemakers. There is evidence for a distribution of temporal oscillators emitting frequencies with a mean of approximately 24.75 Hz. How would this system function? The present hypothesis is that each oscillation comes from a separate pacemaker consisting of a temporal oscillator and a calibration unit. Two purposes would be served by having multiple pacemakers. First, different pacemakers may time the different components of a complex action, so that we can control the pacing of each separately. Second, in normal chaotic experience we must keep track of the times of occurrence and durations of the many overlapping events around us. A separate pacemaker selected from the set available may be dedicated to monitor each such event as it occurs. Just as the eye contains an extended system of receptors, designed to respond in parallel to light from the different objects around us, so also may the temporal sensory system monitor durations in parallel. As objects appear about us, persist, and disappear, so, perhaps, do different temporal sensory units record their multiple overlapping durations.
Second, the EEG data suggest that sets of oscillators recur, centred on different mean frequencies, at intervals of about 12–13 Hz. Since the phenomena of the world about us persist for shorter or longer periods of time, it may be useful to have sets of TOs running at different rates, each set appropriate for recording events of a different order of duration. Similarly, the movements we make take shorter or longer periods of time and may be organized at higher or lower levels, on different time scales, so that it may be useful to have pacemakers running at different rates available for these purposes.
The frequencies that have been studied here are relatively high, appropriate for mechanisms that process short intervals. There may also be lower-frequency oscillators that time longer intervals, but these remain to be demonstrated.
The ear and eye respond to energies from the environment that impinge upon their receptive surfaces. The tongue and nose sample molecules. To orient us in space, the vestibular system depends on properties the body possesses as a physical object (its response to gravity and inertia). It would seem that the time sense relies on properties of the brain itself as a physical system, the propensity of neurons or neural networks to produce oscillations that can be used as timing devices. Thus one function of the brain may be to act as the sense organ for time.
Rather than our experience being focused on a present moment of no duration intercalated between two realms that do not currently exist (St Augustine, 401/ 1960 ), I suggested earlier that we experience ourselves as included in and oriented in relation to a series of overlapping levels of time perception representing different orders of duration. The electroen-cephalographic evidence has suggested that we possess sets of oscillators running at different rates. We may understand these as forming part of the neural substrate that allows time to be experienced as we do. Thus, imagine that you are listening to a phrase of music. On the present hypothesis, temporal oscillators running at a high frequency would allow you to judge the length of each note in the sequence. You would be aware, at the same time, that the concert was half over. This would rely on the count from a pacemaker set to measure periods of a few hours and running at a correspondingly slower rate. The accuracy given by a higher rate of firing would be traded for the ability to track longer periods of time. So while a change of a fraction of a second might be detectable in the length of a note, you might be wrong by several minutes in your perception of how long the concert had taken so far. A yet slower system, tuned to track periods of the order of a day, might place the concert in the afternoon. Even without the help of the many cues we employ, from clocks to concert programmes, a synthesis of the contributions of these different internal clocks will place the listener securely in an experienced temporal framework.
Ayer, A. J. ( 1956 ) The Problem of Knowledge (Harmondsworth, Middlesex: Penguin Books).
Google Scholar
Google Preview
Einstein, A. ( 1936 ) ‘ Physics and reality ’, Journal of the Tranklin Institute 221 , 349–382. 10.1016/S0016-0032(36)91047-5
Falk, J. L. and Bindra, D. ( 1954 ) ‘ Judgment of time as a function of serial position and stress ’, Journal of Experimental Psychology 47 , 279–282. 10.1037/h0061946
Galileo Galilei ( 1623 /1960) The Assayer , tr. A. C. Danto ; extract reprinted in A. Danto and S. Morgenbesser (eds) Philosophy of Science (New York: Meridian Books), pp. 27–32.
Glass L. and Mackey, M. C. ( 1988 ) Trom Clocks to Chaos: The Rhythms of Life (Princeton, NJ: Princeton University Press).
Hoagland, H. ( 1933 ) ‘ The physiological control of judgments of duration: evidence for a chemical clock ’, Journal of General Psychology 9 , 267–287. 10.1080/00221309.1933.9920937
Hume, D. ( 1739 /1911) A Treatise of Human Nature, Vol. 1 (London: Dent).
James, W. ( 1890 /1950) The Principles of Psychology, Vol. 1 (New York: Dover). 10.1037/11059-000
Kant, I. ( 1787 /1934) Critique of Pure Reason . 2nd edn; tr. J. M. D. Meiklejohn (London: Dent).
Kerr, W. A. and Keil, R. C. ( 1963 ) ‘ A theory and factory experiment on the time-drag concept of boredom ’, Journal of Applied Psychology 47 , 7–9. 10.1037/h0041728
Langer, J. , Wapner, S. and Werner, H. ( 1961 ) ‘ The effect of danger upon the experience of time ’, American Journal of Psychology 74 , 94–97. 10.2307/1419830
Locke, J. ( 1690 /1947) An Essay Concerning Human Understanding (London: Dent).
Lockhart, J. M. ( 1967 ) ‘ Ambient temperature and time estimation ’, Journal of Experimental Psychology 73 , 286–291. 10.1037/h0024077
McGurk, H. and MacDonald, J. ( 1976 ) ‘ Hearing lips and seeing voices ’, Nature 264 , 746–748 . 10.1038/264746a0
Moore-Ede, M. C. , Sulzman, F. M. and Fuller, C. A. ( 1982 ) The Clocks That Time Us (Cambridge, MA: Harvard University Press).
Newton, I. ( 1689 /1960) Philosophiae Naturalis Principia Methematica, Bk. 1, tr. A. Motte and F. Cajori ; extract reprinted in A. Danto and S. Morgenbesser (eds) Philosophy of Science (New York: Meridian Books), pp. 322–329.
Quinton, A. ( 1973 ) The Nature of Things (London: Routledge and Kegan Paul).
Siffre, M. ( 1965 ) Beyond Time, tr. H. Briffault (London: Chatto and Windus).
St Augustine (401/ 1960 ) The Confessions of St. Augustine , tr. R. Warner (New York: Mentor-Omega Books).
Treisman, M. ( 1963 ) ‘Temporal discrimination and the indifference interval: implications for a model of the “internal clock”’, Psychological Monographs 77 (576), 1–31.
Treisman, M. , Cook, N. , Naish, P. L. N. and MacCrone, J. K. ( 1994 ) ‘ The internal clock: electroencephalographic evidence for oscillatory processes underlying time perception ’, Quarterly Journal of Experimental Psychology 47A , 241–289.
Treisman, M. , Faulkner, A. and Naish, P. L. N. ( 1992 ) ‘ On the relation between time perception and the timing of motor action: evidence for a temporal oscillator controlling the timing of movement ’, Quarterly Journal of Experimental Psychology 45A , 235–263.
Treisman, M. , Faulkner, A. , Naish, P. L. N. and Brogan, D. ( 1990 ) ‘ The internal clock: evidence for a temporal oscillator underlying time perception with some estimates of its characteristic frequency ’, Perception 19 , 705–743. 10.1068/p190705
I wish to acknowledge support extended by the Leverhulme Trust. Correspondence may be addressed to Michel Treisman, Department of Experimental Psychology, University of Oxford, South Parks Road, Oxford OX1 3UD. Email: [email protected]
- About Oxford Academic
- Publish journals with us
- University press partners
- What we publish
- New features
- Open access
- Institutional account management
- Rights and permissions
- Get help with access
- Accessibility
- Advertising
- Media enquiries
- Oxford University Press
- Oxford Languages
- University of Oxford
Oxford University Press is a department of the University of Oxford. It furthers the University's objective of excellence in research, scholarship, and education by publishing worldwide
- Copyright © 2024 Oxford University Press
- Cookie settings
- Cookie policy
- Privacy policy
- Legal notice
This Feature Is Available To Subscribers Only
Sign In or Create an Account
This PDF is available to Subscribers Only
For full access to this pdf, sign in to an existing account, or purchase an annual subscription.

IMAGES
VIDEO
COMMENTS
7. The metaphysics of time perception. In giving an account of the various aspects of time perception, we inevitably make use of concepts that we take to have an objective counterpart in the world: the past, temporal order, causation, change, the passage of time and so on.
The term time perception refers to a large subfield within the more general study of the psychology of time. It is an old and venerable topic in psychology. When psychology emerged from philosophy and medicine in the late 1800s, time perception became a major topic of interest. Researchers investigated many aspects of the psychology of time ...
Abstract. Although the study of time has been central to physics and philosophy for millennia, questions of how time is represented in the brain and how this representation is related to time perception have only recently started to be addressed. Emerging evidence subtly yet profoundly challenges our intuitive notions of time over short scales ...
Perception. Time perception is inherently subjective and malleable. We experience a wide range of time scales, from less than a second to decades. In addition, our perception of time can be ...
Time is a universal psychological dimension, but time perception has often been studied and discussed in relative isolation. Increasingly, researchers are searching for unifying principles and integrated models that link time perception to other domains. In this review, we survey the links between temporal cognition and other psychological processes. Specifically, we describe how subjective ...
Time perception. In psychology and neuroscience, time perception or chronoception is the subjective experience, or sense, of time, which is measured by someone's own perception of the duration of the indefinite and unfolding of events. [1][2][3] The perceived time interval between two successive events is referred to as perceived duration.
Neurosci., 05 March 2018. Feel the Time. Time Perception as a Function of Interoceptive Processing. The nature of time is rooted in our body. Constellations of impulses arising from the flesh constantly create our interoceptive perception and, in turn, the unfolding of these perceptions defines human awareness of time.
The aim of the present review article is to guide the reader through portions of the human time perception, or temporal processing, literature. After distinguishing the main contemporary issues related to time perception, the article focuses on the main findings and explanations that are available in the literature on explicit judgments about temporal intervals. The review emphasizes studies ...
Sean Power is a philosopher researching time, illusion, perception, and epistemology. He is a research affiliate at the University College Cork, Ireland, whose works include Philosophy of Time and Perceptual Experience (2018) and Philosophy of Time: A Contemporary Introduction (forthcoming).
It also examines the neural mechanisms by which people keep track of time. The answer to this question is a model in which the nervous system itself produces temporal information, in the form of a ‘pacemaker’ or pacemakers that emit pulses at regular characteristic intervals; the model also includes a ‘calibration unit’ to allow for ...