What are the Three Lines of Defense?
- Download PDF Copy
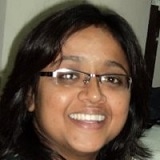
The human body has three primary lines of defense to fight against foreign invaders, including viruses, bacteria, and fungi. The immune system’s three lines of defense include physical and chemical barriers, non-specific innate responses, and specific adaptive responses.
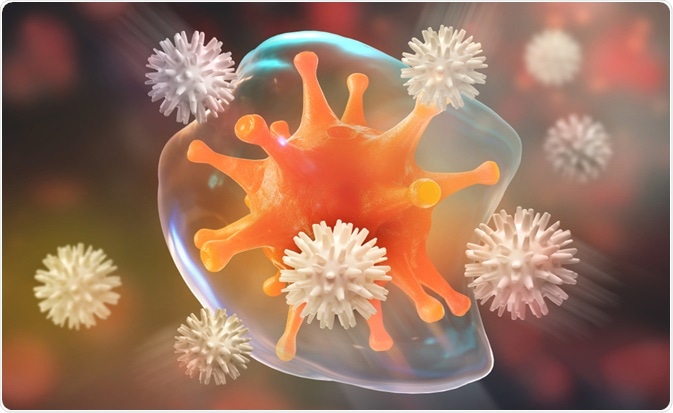

What is the immune system?
The immune system is a complex network of specific immune cells and proteins that work in synergy to protect the body against foreign invaders and harmful toxic materials coming from the environment.
Foreign substances that trigger an immune response are called antigens . However, under certain circumstances, such as in autoimmune diseases, the immune system can be activated by self-antigens, leading to the destruction of the body’s cellular components.
In general, the immune system can be activated to generate two types of immune responses: nonspecific response (innate immunity) and specific adaptive response (acquired immunity).
What are the three lines of defense of the immune system?
The immune system comprises three levels of defense mechanism that a pathogen needs to cross to develop infection inside the body.
Physical barrier
The innate immune system provides the first line of defense, which is divided broadly into two categories – physical/chemical barriers and nonspecific resistance.
Physical barriers, including the skin and mucosa of the digestive and respiratory tracts, help eliminate pathogens and prevent tissue and/or blood infections. Moreover, components that are secreted by the skin or mucosa, such as sweat, saliva, tears, mucous, help provide a basic barrier against invading pathogens.
The skin is the impermeable physical/mechanical barrier that protects many pathogens from entering the body. Similarly, mucosa or mucous membranes that line the immediate internal systems help trap pathogens by producing mucous. Hairs inside the nasal cavity, as well as cerumen (earwax), also trap pathogens and environmental pollutants.
Some acidic fluids, such as gastric juice, urine, and vaginal secretions, destroy pathogens by creating low pH conditions. Also, lysozyme found in tears, sweat, and saliva acts as a vital antimicrobial agent to destroy pathogens.
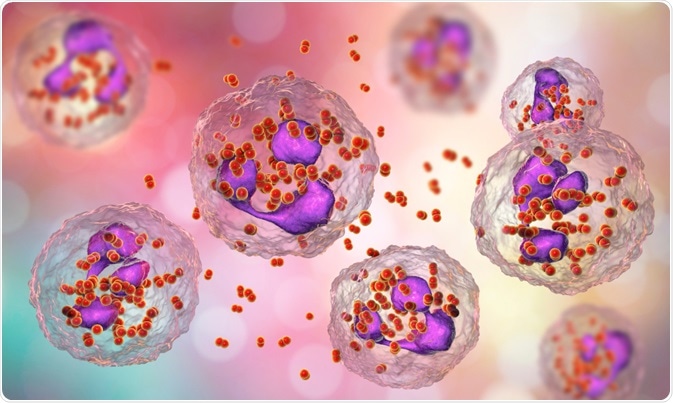
Nonspecific innate response
Pathogens that successfully cross the physical barriers are next encountered by the second line of defense. This innate immune response mostly involves immune cells and proteins to nonspecifically recognize and eliminate any pathogen that enters the body.
Phagocytosis is a crucial phenomenon of the innate immune system that utilizes a special type of immune cells called phagocytes. There are two types of phagocytes namely macrophages and neutrophils. These cells are found in the tissues and blood.
In the beginning, phagocytes recognize and bind pathogens and then use the plasma membrane to surround and engulf pathogens inside the cell. As a result, a separate internal compartment (phagosome) is generated, which subsequently fuses with another type of cellular compartment called the lysosome. The digestive enzymes present inside lysosomes finally destroy pathogens by breaking them into fragments.
Digestion of pathogens inside a phagosome produces indigestible materials and antigenic fragments; of which, indigestible materials are removed by exocytosis. However, the antigenic fragments are displayed on the surface of phagocytes, which are subsequently recognized and destroyed by cytotoxic T cells .
In addition, complement proteins are activated, which in turn recruit more white blood cells (neutrophils, eosinophils, and basophils) at the site of infection, leading to an inflammatory response (swelling, redness, pain).
Specific adaptive response
The third line defense aims at eliminating specific pathogens that have been encountered by the immune system previously (adaptive or acquired immune response). Instead of being restricted to the site of infection, the adaptive immune response occurs throughout the body.
The adaptive immune system mainly involves two types of white blood cells (lymphocytes) – B lymphocytes (B cells) and T lymphocytes (T cells). B cells are involved in antibody-mediated immune responses (humoral immunity), whereas T cells are involved in cell-mediated immune responses.
In antibody-mediated immunity, B cells are activated when they encounter a ‘known’ antigen. Activated B cells then engulf and digest the antigen, which is followed by a representation of MHC (major histocompatibility complex)-bound antigenic fragments on the B cell surface.
The combination of antigen-MHC further activates helper T cells, which in turn secrete cytokines (interleukins) to trigger the growth and maturation of antigen-presenting B cells into antibody-producing B cells (plasma cells). At this point, some B cells are transformed into memory cells to keep the immune system ready for the next attack.
Antibodies produced by the plasma cells are secreted into the bloodstream where they execute their functions in different ways. For example, by forming the antigen-antibody complex, antibodies can prevent antigens from binding host cells, leading to the prevention of infection. Antibodies also bind and mark pathogens for destruction through phagocytosis.
The antigen-antibody complex can initiate a series of signaling events to activate complement proteins, which in turn kills pathogens by rupturing their cell membrane. Complement proteins also trigger an inflammatory response, leading to the accumulation of white blood cells at the infection site.
In cell-mediated immunity, T cells are activated when they encounter antigen-presenting cells, such as B cells or dendritic cells. Activated T cells then secrete cytokines that further trigger the production and maturation of T cells.
T cells that mature into cytotoxic or killer T cells mainly destroy pathogen-infected cells, damaged cells, and cancer cells by rupturing the cell membrane. Whereas, T cells that mature into helper T cells facilitate B cells to execute antibody-mediated immune responses.
Some T cells that mature into regulatory T cells help cease the immune response and maintain the immune system homeostasis when the threat is eliminated. Also, some T cells that mature into memory T cells remember the pathogen and initiate an immediate response when the body encounters the same pathogen for the second time.
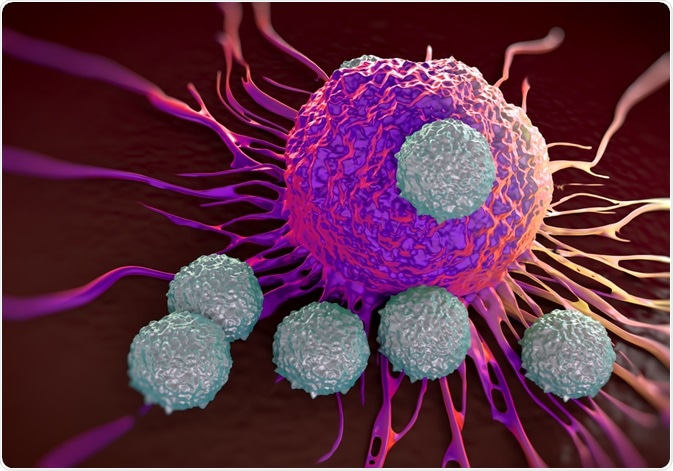
Image Credit: royaltystockphoto.com/Shutterstock.com
- Science Olympiad. Immune System. https://www.soinc.org/sites/default/files/uploaded_files/2018_IMMUNE_SYSTEM_HANDOUT.pdf
- Let’s talk science. 2019. The immune response. https://letstalkscience.ca/educational-resources/stem-in-context/immune-response
- Austin Community College. Immune System. https://www.austincc.edu/apreview/EmphasisItems/Inflammatoryresponse.html
- NCBI. 2020. How does the immune system work? https://www.ncbi.nlm.nih.gov/books/NBK279364/
Further Reading
- All Immune System Content
- What is the difference Between a Phagocyte, Macrophage, Neutrophil and Eosinophil?
- Does the Immune System Differ between Men and Women?
- What is Immune Fitness?
- Effects of Tobacco on the Immune System
Last Updated: Mar 11, 2021
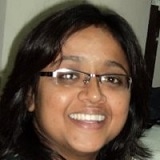
Dr. Sanchari Sinha Dutta
Dr. Sanchari Sinha Dutta is a science communicator who believes in spreading the power of science in every corner of the world. She has a Bachelor of Science (B.Sc.) degree and a Master's of Science (M.Sc.) in biology and human physiology. Following her Master's degree, Sanchari went on to study a Ph.D. in human physiology. She has authored more than 10 original research articles, all of which have been published in world renowned international journals.
Please use one of the following formats to cite this article in your essay, paper or report:
Dutta, Sanchari Sinha Dutta. (2021, March 11). What are the Three Lines of Defense?. News-Medical. Retrieved on September 16, 2024 from https://www.news-medical.net/health/What-are-the-Three-Lines-of-Defense.aspx.
Dutta, Sanchari Sinha Dutta. "What are the Three Lines of Defense?". News-Medical . 16 September 2024. <https://www.news-medical.net/health/What-are-the-Three-Lines-of-Defense.aspx>.
Dutta, Sanchari Sinha Dutta. "What are the Three Lines of Defense?". News-Medical. https://www.news-medical.net/health/What-are-the-Three-Lines-of-Defense.aspx. (accessed September 16, 2024).
Dutta, Sanchari Sinha Dutta. 2021. What are the Three Lines of Defense? . News-Medical, viewed 16 September 2024, https://www.news-medical.net/health/What-are-the-Three-Lines-of-Defense.aspx.

Is there a possible pathogen that the body can not build antibody against that? If yes what will happen but death?!
Cancel reply to comment
- Trending Stories
- Latest Interviews
- Top Health Articles

How can microdialysis benefit drug development
Ilona Vuist
In this interview, discover how Charles River uses the power of microdialysis for drug development as well as CNS therapeutics.

Global and Local Efforts to Take Action Against Hepatitis
Lindsey Hiebert and James Amugsi
In this interview, we explore global and local efforts to combat viral hepatitis with Lindsey Hiebert, Deputy Director of the Coalition for Global Hepatitis Elimination (CGHE), and James Amugsi, a Mandela Washington Fellow and Physician Assistant at Sandema Hospital in Ghana. Together, they provide valuable insights into the challenges, successes, and the importance of partnerships in the fight against hepatitis.

Addressing Important Cardiac Biology Questions with Shotgun Top-Down Proteomics
In this interview conducted at Pittcon 2024, we spoke to Professor John Yates about capturing cardiomyocyte cell-to-cell heterogeneity via shotgun top-down proteomics.

Latest News

Newsletters you may be interested in

Your AI Powered Scientific Assistant
Hi, I'm Azthena, you can trust me to find commercial scientific answers from News-Medical.net.
A few things you need to know before we start. Please read and accept to continue.
- Use of “Azthena” is subject to the terms and conditions of use as set out by OpenAI .
- Content provided on any AZoNetwork sites are subject to the site Terms & Conditions and Privacy Policy .
- Large Language Models can make mistakes. Consider checking important information.
Great. Ask your question.
Azthena may occasionally provide inaccurate responses. Read the full terms .
While we only use edited and approved content for Azthena answers, it may on occasions provide incorrect responses. Please confirm any data provided with the related suppliers or authors. We do not provide medical advice, if you search for medical information you must always consult a medical professional before acting on any information provided.
Your questions, but not your email details will be shared with OpenAI and retained for 30 days in accordance with their privacy principles.
Please do not ask questions that use sensitive or confidential information.
Read the full Terms & Conditions .
Provide Feedback
NOTIFICATIONS
The body’s first line of defence.
- + Create new collection
Your body has a two-line defence system against pathogens (germs) that make you sick. Pathogens include bacteria, viruses, toxins, parasites and fungi.
The first line of defence (or outside defence system) includes physical and chemical barriers that are always ready and prepared to defend the body from infection. These include your skin, tears, mucus, cilia, stomach acid, urine flow, ‘friendly’ bacteria and white blood cells called neutrophils.
Pathogenic (disease-causing) microorganisms must make it past this first line of defence. If this defence is broken, the second line of defence within your body is activated.
Skin The skin is the largest organ of your body. It acts as a barrier between invaders (pathogens) and your body. Skin forms a waterproof mechanical barrier. Microorganisms that live all over your skin can’t get through your skin unless it’s broken.
Tears, mucus and saliva Your nose, mouth and eyes are obvious entry points for pathogens. However, tears, mucus and saliva contain an enzyme that breaks down the cell wall of many bacteria. Those that are not killed immediately are trapped in mucus and swallowed. Special cells line and protect the nose, throat and other passages within your body. The inner lining of your gut and lungs also produces mucus to trap invading pathogens.
Cilia Very fine hairs (cilia) lining your windpipe move mucus and trapped particles away from your lungs. Particles can be bacteria or material such as dust or smoke.
Stomach acid Stomach acid kills bacteria and parasites that have been swallowed.
Urine flow Your urine flow flushes out pathogens from the bladder area.
‘Friendly’ (beneficial) bacteria You have beneficial bacteria growing on your skin, in your bowel and other places in the body (such as the mouth and the gut) that stop other harmful bacteria from taking over.
Neutrophils These are white blood cells that can find, kill and ingest pathogens seeking an entrance into the body.
You may now like to read this article The body's second line of defence .
Related content
See our newsletters here .
Would you like to take a short survey?
This survey will open in a new tab and you can fill it out after your visit to the site.
AP Notes, Outlines, Study Guides, Vocabulary, Practice Exams and more!

Class Notes
Social science.
- European History
- Human Geography
- US Gov and Politics
- World History
- Trigonometry
- Environmental Science
- Art History
- Music Theory
Textbook Notes
Members only.
- Textbook Request
You are here
1st and 2nd lines of defense.
skin - 1st line of defense
- 15% of an adult’s total weight
- oil/sweat glands >> low pH on surface >> many microorganisms killed
- prevents water loss
- lyxozyme - enzyme in sweat that digests bacterial cell walls
- cells constantly injured, worn, replaced
- stratum basale - innermost skin layer, produces new skin cells
- stratum spinosum - broad layer in middle of epidermis
- dermis - skin layer below epidermis, gives structural support
- mainly adipose (fat) cells below dermis
- mucus traps microorganisms in bronchi, cilia sweeps mucus towards glottis to stomach
cellular counterattack - 2nd line of defense
- uses nonspecific cellular/chemical devices to defend
- goes after any infection w/ leukocytes
- identity of pathogen doesn’t matter
- lymphatic system = central location for distribution of immune system cells
- ingests microbes through phagocytosis
- uses oxygen-free radicals to destroy microbes
- can release chemicals (similar to bleach) to kill all cells in surroundings
- drills hole into plasma membrane
complement system - in vertebrates, contains 20 proteins
- proteins encounter bacterial/fungal cell wall >> forms membrane attack complex
- forms pore on membrane >> cell swells/bursts
- adds on to the effects of other body defenses
- alpha, beta, gamma
- prostaglandin - produces clotting to block spread of pathogens
inflammatory response - localized, nonspecific response to infection
- infected cells release alarm signals >> blood vessels dilate >> increase blood flow >> area = red/warm
- pus = mixture of dead pathogens, tissue, neutrophils
- fever >> stimulates phagocytosis, iron production
- very high fever could start to denature enzymes
We hope your visit has been a productive one. If you're having any problems, or would like to give some feedback, we'd love to hear from you.
For general help, questions, and suggestions, try our dedicated support forums .
If you need to contact the Course-Notes.Org web experience team, please use our contact form .
Need Notes?
While we strive to provide the most comprehensive notes for as many high school textbooks as possible, there are certainly going to be some that we miss. Drop us a note and let us know which textbooks you need. Be sure to include which edition of the textbook you are using! If we see enough demand, we'll do whatever we can to get those notes up on the site for you!
About Course-Notes.Org
- Advertising Opportunities
- Newsletter Archive
- Plagiarism Policy
- Privacy Policy
- DMCA Takedown Request
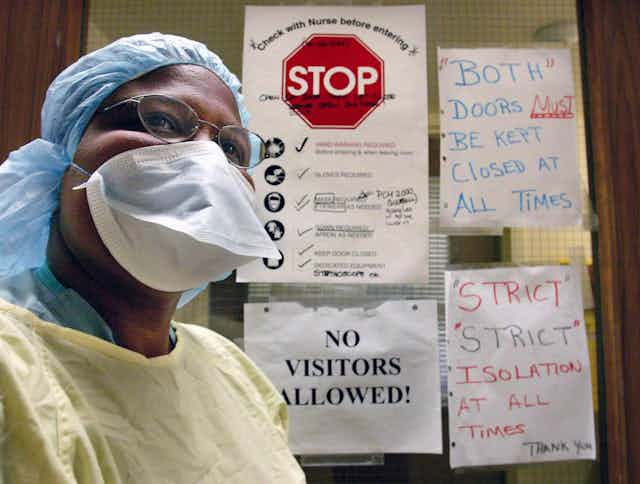
Explainer: How the human body first fights off pathogens
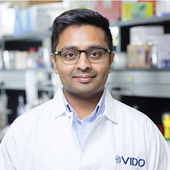
PhD Candidate in Veterinary Microbiology, University of Saskatchewan
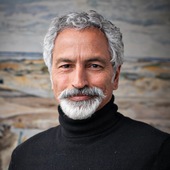
Professor of Veterinary Microbiology, University of Saskatchewan
Disclosure statement
Arinjay Banerjee receives funding from the Saskatchewan Innovation and Opportunity Scholarship.
Vikram Misra receives funding from Natural Sciences and Engineering Research Council (NSERC) of Canada through its Discovery and Collaborative Research and Training Enterprise (CREATE) funding programs.
University of Saskatchewan provides funding as a founding partner of The Conversation CA.
University of Saskatchewan provides funding as a member of The Conversation CA-FR.
View all partners
People constantly encounter viruses, bacteria or parasites. Fortunately, our skin, the specialized lining of our guts and other parts of our body that are exposed to the outside world prevent them from entering. When a pathogen breaches this barrier, our body’s defences come into play.
Those defences, which we also call the human immune system, have two branches — innate and adaptive . Our innate immune system is our first line of defence. It has special molecules that recognize “fingerprints” or patterns in proteins or genetic material that are only present in pathogens. These molecules signal the presence of a pathogen to the cellular genetic machinery, which then produces effector molecules called cytokines .
The effector molecules initiate a process to eliminate the pathogen. The adaptive immune response kicks in at a later stage. Its job is to mount a much more robust defence by destroying cells infected with a virus or by neutralizing the virus or toxins produced by bacterial pathogens.
The adaptive system also has a memory and activates very rapidly if it encounters the same pathogen again. The innate and adaptive immune systems are linked and the innate system primes the adaptive branch of immunity for a more robust immune response.
Response against invaders
The innate immune system is primitive and probably evolved earlier in invertebrates and lower vertebrates. Although innate immune response has systemic effects on the entire human body, every cell can be considered a factory housing some components of the innate immune response.
Innate immunity starts at the cellular level through conserved pathogen sensing proteins in the cell that sense a pathogen, such as a virus. During virus infection, sensors in human cells recognize viral proteins and nucleic acids to activate genes, such as interferons that inhibit viral replication.
Once infected cells have sensed an invading pathogen, they secrete molecules called cytokines and chemokines . Cytokines such as interferons are molecules that signal neighbouring cells and induce an antiviral state in them. These cells are then primed to resist an infection with the invading virus. Cytokines such as interferons activate anti-viral genes in the infected and neighbouring cells. These genes are called interferon stimulated genes (ISGs) since they are only made in the presence of interferons. ISGs impede virus replication in the cells.
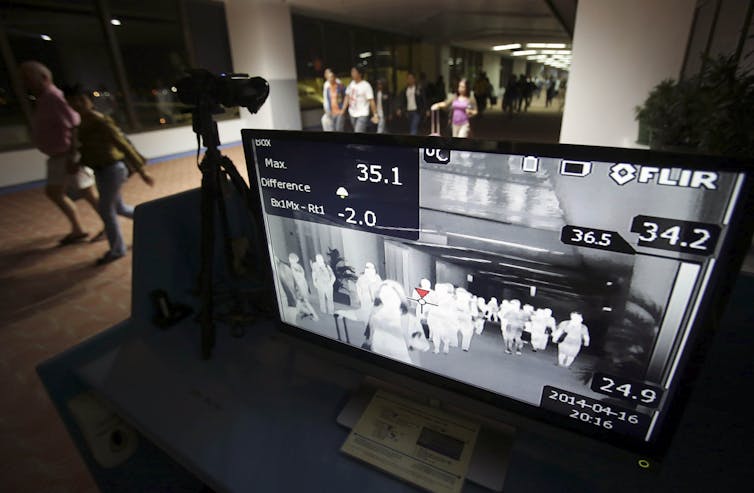
At the tissue level, an infection usually manifests itself in the form of an inflammation. Typical signs of inflammation include redness, swelling, pain, heat and loss of function. This is an area of intense battle between the invading microbe and the body’s immune system. Each cell within this area is like a soldier and several cell types together form an army against the microbes.
Chemokines are molecules that attract specialized immune cells to this site of infection. These include cells that “eat” pathogens and dead cells such as macrophages . In the battlefield, chemokines are like “radio calls.”
Macrophages respond to these calls and invade the site of infection, consuming any cell-free or cell-associated pathogen. These pathogens are then destroyed by internal mechanisms in the macrophages. Macrophages are also like the medics in a battle zone. They help remove damaged cells (“wounded soldiers”) and clear the area.
White blood cells such as neutrophils respond to chemokines by migrating to the site of infection. These cells secrete powerful inflammatory molecules and reactive oxygen species that aid in getting rid of the pathogen.
Neutrophils, just like macrophages, can also ingest microorganisms or particles. Neutrophils are cells that have internal granules that house powerful chemicals. When neutrophils accumulate at the site of infection, they release these chemicals that have bactericidal properties.
An evolutionary battle
Pathogens continue to evolve new mechanisms to evade the body’s immune response. Charles Darwin’s theory of evolution and survival of the fittest are relevant for microbes as well. Just like bacteria evolve to become resistant to antibiotics, viruses evolve ways to evade the immune responses.
Coronaviruses have evolved mechanisms to shut down cytokine production. In the absence of cytokines, the cell has no way to communicate with other cells about the viral infection. The virus can then multiply to higher levels, causing significant infection and even death in humans. This has been observed both in severe acute respiratory syndrome (SARS) and Middle-East respiratory syndrome (MERS) .
It’s therefore very important to identify the mechanisms employed by these viruses to shut down host defences. Drugs can then be designed to inhibit these viral mechanisms and boost human innate immune responses.
The innate immune system has control mechanisms to inhibit damage to the human body by excessive secretion of cytokines. Cytokines are deemed necessary for controlling virus replication, but excessive production of cytokines leads to tissue damage. Excessive cytokine production, a phenomenon called “cytokine storm” in SARS patients has been associated with lung damage. So a timely balance between inflammatory and anti-inflammatory processes is ideal to tackle infections.
The innate immune response is really the sentinel of the human body; standing at the doors to detect invading pathogens. These sentinels fight off an invading organism while alerting the adaptive immune response (“the cannons”) about the threat.
- Immune system
- Innate immune response
- Middle East Respiratory Syndrome (MERS)
- Severe acute respiratory syndrome (SARS)

Professor of Indigenous Cultural and Creative Industries (Identified)

Communications Director

Associate Director, Post-Award, RGCF

University Relations Manager

2024 Vice-Chancellor's Research Fellowships

Want to create or adapt books like this? Learn more about how Pressbooks supports open publishing practices.
17 Innate Immunity
The immune system in vertebrates, including humans, is a complex multilayered system for defending against external and internal threats to the integrity of the body. Many of these threats are caused by infectious microbes, called pathogens , which include viruses, bacteria, fungi, and parasites. The system can be divided into two types of defense systems: the innate immune system, which is nonspecific toward a particular kind of pathogen, and the adaptive immune system, which is specific ( Figure 1 ). Innate immunity is not caused by an infection or vaccination and depends initially on physical and chemical barriers that work on all pathogens, sometimes called the first line of defense. The second line of defense of the innate system includes chemical signals that produce inflammation and fever responses as well as mobilizing protective cells and other chemical defenses. Together, the two parts of the innate immune response to a pathogen produce a fast response that is the same every time a pathogen enters the body. This response does not recognize a specific pathogen, but rather recognizes broad patterns of molecules that are not found in healthy human cells or tissues. In contrast, the adaptive immune system mounts a highly specific response to substances and organisms that do not belong in the body, recognizing unique molecular “signatures” found on each pathogen. The adaptive system takes longer to respond and has a memory system that allows it to respond with greater intensity should the body reencounter a the same pathogen again, even years later.
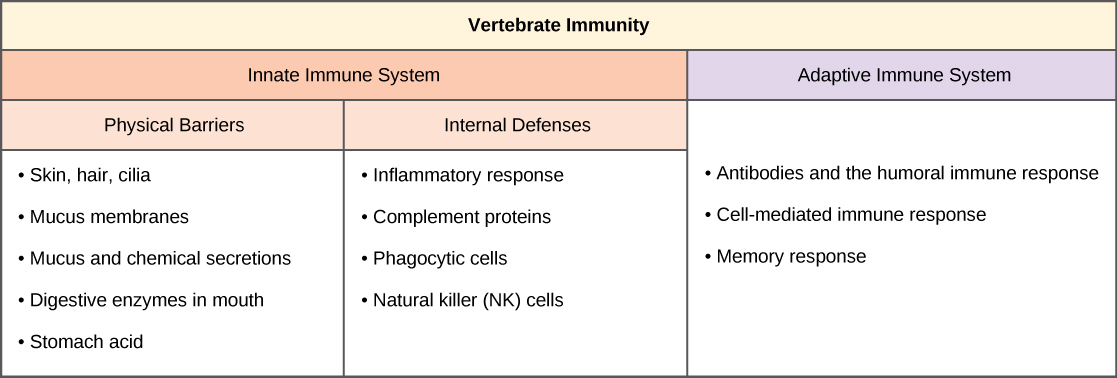
External and Chemical Barriers
The body has significant physical barriers to potential pathogens. The skin contains the protein keratin, which resists physical entry into cells. Other body surfaces, particularly those associated with body openings, are protected by the mucous membranes. The sticky mucus provides a physical trap for pathogens, preventing their movement deeper into the body. The openings of the body, such as the nose and ears, are protected by hairs that catch pathogens, and the mucous membranes of the upper respiratory tract have cilia that constantly move pathogens trapped in the mucus coat up to the mouth.
The skin and mucous membranes also create a chemical environment that is hostile to many microorganisms. The surface of the skin is acidic, which prevents bacterial growth. Saliva, mucus, and the tears of the eye contain an enzyme that breaks down bacterial cell walls. The stomach secretions create a highly acidic environment, which kills many pathogens entering the digestive system.
Finally, the surface of the body and the lower digestive system have a community of microorganisms such as bacteria, archaea, and fungi that coexist without harming the body. There is evidence that these organisms are highly beneficial to their host, combating disease-causing organisms and outcompeting them for nutritional resources provided by the host body. Despite these defenses, pathogens may enter the body through skin abrasions or punctures, or by collecting on mucosal surfaces in large numbers that overcome the protections of mucus or cilia.
Internal Defenses
When pathogens enter the body, the innate immune system responds with a variety of internal defenses. These include the inflammatory response, phagocytosis, natural killer cells, and the complement system. White blood cells in the blood and lymph recognize pathogens as foreign to the body, and carry out these defense responses, often with the help of chemical signaling molecules they release. A white blood cell, also called a leukocyte, is larger than a red blood cell, is nucleated, and is typically able to move using amoeboid locomotion. Because they can move on their own, white blood cells can leave the blood to go to infected tissues. For example, a monocyte is a type of white blood cell that circulates in the blood and lymph and develops into a macrophage after it moves into infected tissue. A macrophage is a large cell that engulfs foreign particles and pathogens. Mast cells are produced in the same way as white blood cells, but unlike circulating white blood cells, mast cells take up residence in the tissues other than blood. They are responsible for releasing chemicals in response to physical injury.
When a pathogen is recognized by white blood cells, chemicals called cytokines are released. A cytokine is a chemical messenger that regulates many different cellular processes, including cell division and gene expression, to produce a variety of immune responses. Approximately 40 types of cytokines exist in humans. In addition to being released from white blood cells after pathogen recognition, cytokines are also released by the infected cells and bind to nearby uninfected cells, inducing those cells to release cytokines. This positive feedback loop results in a burst of cytokine production.
One class of early-acting cytokines is the interferons, which are released by infected cells as a warning to nearby uninfected cells. An interferon is a small protein that signals a viral infection to other cells. The interferons stimulate uninfected cells to produce compounds that interfere with viral replication. Interferons also activate macrophages and other cells.
The Inflammatory Response and Phagocytosis
The first cytokines to be produced encourage inflammation , a localized redness, swelling, heat, and pain. Inflammation is a response to physical trauma, such as a cut or a blow, chemical irritation, and infection by pathogens (viruses, bacteria, or fungi). The chemical signals that trigger an inflammatory response enter the extracellular fluid and cause capillaries to dilate (expand) and capillary walls to become more permeable, or leaky. The serum and other compounds leaking from capillaries cause swelling of the area, which in turn causes pain. Various kinds of white blood cells are attracted to the area of inflammation. The types of white blood cells that arrive at an inflamed site depend on the nature of the injury or infecting pathogen. For example, a neutrophil is an early arriving white blood cell that engulfs and digests pathogens. Neutrophils are the most abundant white blood cells of the immune system ( Figure 2 ). Macrophages follow neutrophils and take over the phagocytosis function and are involved in the resolution of an inflamed site, cleaning up cell debris and pathogens. The process of ingesting and digesting up pathogens is called phagocytosis, and the cells that carry this process out are called phagocytes.
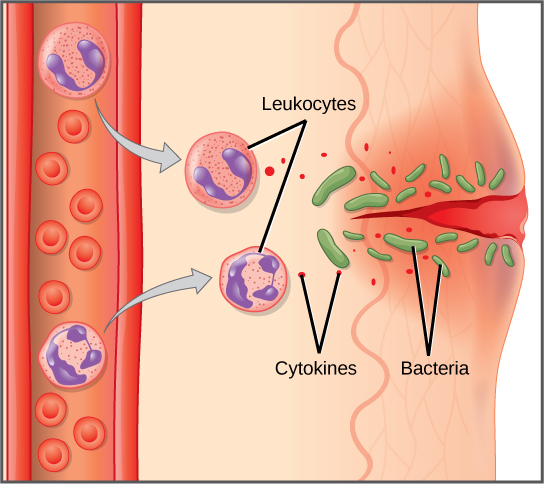
Cytokines also send feedback to cells of the nervous system to bring about the overall symptoms of feeling sick, which include lethargy, muscle pain, and nausea. Cytokines also increase the core body temperature, causing a fever. The elevated temperatures of a fever inhibit the growth of pathogens and speed up cellular repair processes. For these reasons, suppression of fevers should be limited to those that are dangerously high.
Concept in Action
Natural Killer Cells
A lymphocyte is a white blood cell that contains a large nucleus ( Figure 3 ). Most lymphocytes are associated with the adaptive immune response, but infected cells are identified and destroyed by natural killer cells, the only lymphocytes of the innate immune system. A natural killer (NK) cell is a lymphocyte that can kill cells infected with viruses (or cancerous cells). NK cells identify intracellular infections, especially from viruses, by the altered expression of particular types of proteins on the surface of infected cells. Unhealthy cells, whether infected or cancerous, display an altered set of proteins on their cell surfaces.
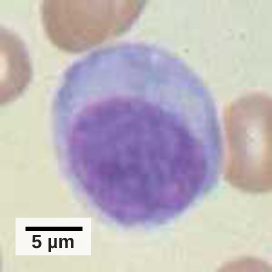
After the NK cell detects an infected or tumor cell, it induces programmed cell death, or apoptosis. Phagocytic cells then come along and digest the cell debris left behind. NK cells are constantly patrolling the body and are an effective mechanism for controlling potential infections and preventing cancer progression. The various types of immune cells are shown in Figure 4 .
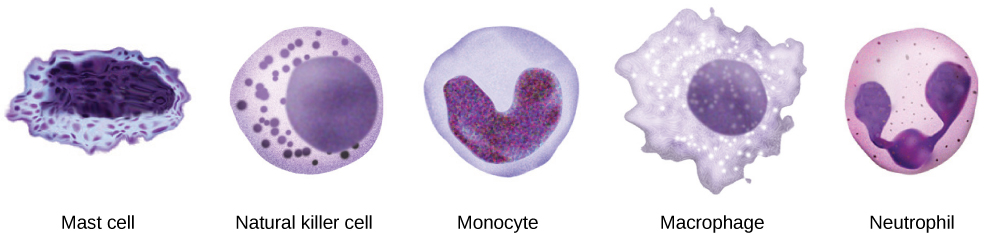
An array of approximately 20 types of proteins, called a complement system , is also activated by infection or the activity of the cells of the adaptive immune system and functions to destroy extracellular pathogens. Liver cells and macrophages synthesize inactive forms of complement proteins continuously; these proteins are abundant in the blood serum and are capable of responding immediately to infecting microorganisms. The complement system is so named because it is complementary to the innate and adaptive immune system. Complement proteins bind to the surfaces of microorganisms and are particularly attracted to pathogens that are already tagged by the adaptive immune system. This “tagging” involves the attachment of specific proteins called antibodies (discussed in detail later) to the pathogen. When they attach, the antibodies change shape providing a binding site for one of the complement proteins. After the first few complement proteins bind, a cascade of binding in a specific sequence of proteins follows in which the pathogen rapidly becomes coated in complement proteins.
Complement proteins perform several functions, one of which is to serve as a marker to indicate the presence of a pathogen to phagocytic cells and enhance engulfment. Certain complement proteins can combine to open pores in microbial cell membranes and cause lysis of the cells.
Section Summary
The innate immune system consists first of physical and chemical barriers to infection including the skin and mucous membranes and their secretions, ciliated surfaces, and body hairs. The second line of defense is an internal defense system designed to counter pathogenic threats that bypass the physical and chemical barriers of the body. Using a combination of cellular and molecular responses, the innate immune system identifies the nature of a pathogen and responds with inflammation, phagocytosis, cytokine release, destruction by NK cells, or the complement system.
pathogen a microbe (virus, bacteria, fungus, parasite) capable of causing disease
CC LICENSED CONTENT, SHARED PREVIOUSLY
OpenStax, Concepts of Biology, Section 17.2 Innate Immunity
Provided by: Rice University
Access for free at https://openstax.org/details/books/concepts-biology
License: CC-BY 4.0
Adapted By: Sarah Malmquist
Human Biology 2nd edition Copyright © by Sarah Malmquist and Kristina Prescott is licensed under a Creative Commons Attribution-NonCommercial 4.0 International License , except where otherwise noted.
Share This Book
Volume 14 Supplement 2
Practical guide for allergy and immunology in Canada 2018
- Open access
- Published: 12 September 2018
An introduction to immunology and immunopathology
- Jean S. Marshall 1 ,
- Richard Warrington 2 ,
- Wade Watson 3 &
- Harold L. Kim 4 , 5
Allergy, Asthma & Clinical Immunology volume 14 , Article number: 49 ( 2018 ) Cite this article
331k Accesses
519 Citations
282 Altmetric
Metrics details
Beyond structural and chemical barriers to pathogens, the immune system has two fundamental lines of defense: innate immunity and adaptive immunity. Innate immunity is the first immunological mechanism for fighting against an intruding pathogen. It is a rapid immune response, initiated within minutes or hours after aggression, that has no immunologic memory. Adaptive immunity, on the other hand, is antigen-dependent and antigen-specific; it has the capacity for memory, which enables the host to mount a more rapid and efficient immune response upon subsequent exposure to the antigen. There is a great deal of synergy between the adaptive immune system and its innate counterpart, and defects in either system can provoke illness or disease, such as inappropriate inflammation, autoimmune diseases, immunodeficiency disorders and hypersensitivity reactions. This article provides a practical overview of innate and adaptive immunity, and describes how these host defense mechanisms are involved in both heath and illness.
There are continuous advances in our current understanding of the immune system and how it functions to protect the body from infection. Given the complex nature of this subject, it is beyond the scope of this article to provide an in-depth review of all aspects of immunology. Rather, the purpose of this article is to provide medical students, medical residents, primary-care practitioners and other healthcare professionals with a basic introduction to the main components and function of the immune system and its role in both health and disease. This article will also serve as a backgrounder to the immunopathological disorders discussed in the remainder of this supplement.
The immune system: innate and adaptive immunity
The immune system refers to a collection of cells, chemicals and processes that function to protect the skin, respiratory passages, intestinal tract and other areas from foreign antigens, such as microbes (organisms such as bacteria, fungi, and parasites), viruses, cancer cells, and toxins. Beyond, the structural and chemical barriers which protect us from infection, the immune system can be simplistically viewed as having two “lines of defense”: innate immunity and adaptive immunity. Innate immunity represents the first line of defense to an intruding pathogen. It is an antigen-independent (non-specific) defense mechanism that is used by the host immediately or within hours of encountering an antigen. The innate immune response has no immunologic memory and, therefore, it is unable to recognize or “memorize” the same pathogen should the body be exposed to it in the future. Adaptive immunity, on the other hand, is antigen-dependent and antigen-specific and, therefore, involves a lag time between exposure to the antigen and maximal response. The hallmark of adaptive immunity is the capacity for memory which enables the host to mount a more rapid and efficient immune response upon subsequent exposure to the antigen. Innate and adaptive immunity are not mutually exclusive mechanisms of host defense, but rather are complementary, with defects in either system resulting in host vulnerability or inappropriate responses [ 1 , 2 , 3 ].
Innate immunity
Innate immunity can be viewed as comprising four types of defensive barriers: anatomic (skin and mucous membrane), physiologic (temperature, low pH and chemical mediators), endocytic and phagocytic, and inflammatory. Table 1 summarizes the non-specific host-defense mechanisms for each of these barriers. Cells and processes that are critical for effective innate immunity to pathogens that evade the anatomic barriers have been widely studied. Innate immunity to pathogens relies on pattern recognition receptors (PRRs) which allow a limited range of immune cells to detect and respond rapidly to a wide range of pathogens that share common structures, known as pathogen associated molecular patterns (PAMPs). Examples of these include bacterial cell wall components such as lipopolysaccharides (LPS) and double-stranded ribonucleic acid (RNA) produced during viral infection.
An important function of innate immunity is the rapid recruitment of immune cells to sites of infection and inflammation through the production of cytokines and chemokines (small proteins involved in cell–cell communication and recruitment). Cytokine production during innate immunity mobilizes many defense mechanisms throughout the body while also activating local cellular responses to infection or injury. Key inflammatory cytokines released during the early response to bacterial infection are: tumour necrosis factor (TNF), interleukin 1 (IL-1) and interleukin 6 (IL-6). These cytokines are critical for initiating cell recruitment and the local inflammation which is essential for clearance of many pathogens. They also contribute to the development of fever. Dysregulated production of such inflammatory cytokines is often associated with inflammatory or autoimmune disease, making them important therapeutic targets.
The complement system is a biochemical cascade that functions to identify and opsonize (coat) bacteria and other pathogens. It renders pathogens susceptible to phagocytosis, a process by which immune cells engulf microbes and remove cell debris, and also kills some pathogens and infected cells directly. The phagocytic action of the innate immune response promotes clearance of dead cells or antibody complexes and removes foreign substances present in organs, tissues, blood and lymph. It can also activate the adaptive immune response through the mobilization and activation of antigen-presenting cells (APCs) (discussed later) [ 1 , 3 ].
Numerous cells are involved in the innate immune response such as phagocytes (macrophages and neutrophils), dendritic cells, mast cells, basophils, eosinophils, natural killer (NK) cells and innate lymphoid cells. Phagocytes are sub-divided into two main cell types: neutrophils and macrophages. Both of these cells share a similar function: to engulf (phagocytose) microbes and kill them through multiple bactericidal pathways. In addition to their phagocytic properties, neutrophils contain granules and enzyme pathways that assist in the elimination of pathogenic microbes. Unlike neutrophils (which are short-lived cells), macrophages are long-lived cells that not only play a role in phagocytosis, but are also involved in antigen presentation to T cells (see Fig. 1 ) [ 1 ].
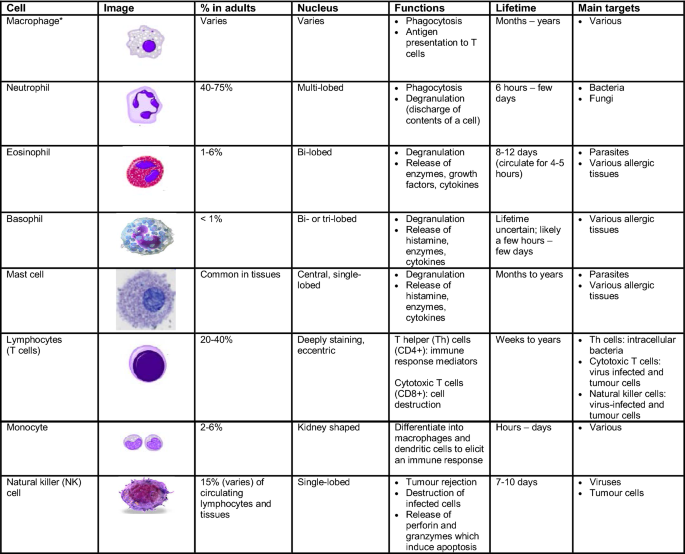
Characteristics and function of cells involved in innate immunity [ 1 , 3 , 4 ]. *Dust cells (within pulmonary alveolus), histiocytes (connective tissue), Kupffer cells (liver), microglial cells (neural tissue), epithelioid cells (granulomas), osteoclasts (bone), mesangial cells (kidney)
Dendritic cells also phagocytose and function as APCs, initiating the acquired immune response and acting as important messengers between innate and adaptive immunity. Mast cells and basophils share many salient features with each other, and both are instrumental in the initiation of acute inflammatory responses, such as those seen in allergy and asthma. Mast cells also have important functions as immune “sentinel cells” and are early producers of cytokines in response to infection or injury. Unlike mast cells, which generally reside in the connective tissue surrounding blood vessels and are particularly common at mucosal surfaces, basophils reside in the circulation. Eosinophils are granulocytes that possess phagocytic properties and play an important role in the destruction of parasites that are often too large to be phagocytosed. Along with mast cells and basophils, they also control mechanisms associated with allergy and asthma. Natural killer (NK) cells play a major role in the rejection of tumours and the destruction of cells infected by viruses. Destruction of infected cells is achieved through the release of perforins and granzymes (proteins that cause lysis of target cells) from NK-cell granules which induce apoptosis (programmed cell death) [ 4 ]. NK cells are also an important source of another cytokine, interferon-gamma (IFN-γ), which helps to mobilize APCs and promote the development of effective anti-viral immunity. Innate lymphoid cells (ILCs) play a more regulatory role. Depending on their type (i.e., ILC-1, ILC-2, ILC-3), they selectively produce cytokines such as IL-4, IFN-γ and IL-17 that help to direct the appropriate immune response to specific pathogens and contribute to immune regulation in that tissue.
The main characteristics and functions of the cells involved in the innate immune response are summarized in Fig. 1 .
Adaptive immunity
The development of adaptive immunity is aided by the actions of the innate immune system, and is critical when innate immunity is ineffective in eliminating infectious agents. The primary functions of the adaptive immune response are: the recognition of specific “non-self” antigens, distinguishing them from “self” antigens; the generation of pathogen-specific immunologic effector pathways that eliminate specific pathogens or pathogen-infected cells; and the development of an immunologic memory that can quickly eliminate a specific pathogen should subsequent infections occur [ 2 ]. Adaptive immune responses are the basis for effective immunization against infectious diseases. The cells of the adaptive immune system include: antigen-specific T cells, which are activated to proliferate through the action of APCs, and B cells which differentiate into plasma cells to produce antibodies.
T cells and APCs
T cells derive from hematopoietic stem cells in bone marrow and, following migration, mature in the thymus. These cells express a series of unique antigen-binding receptors on their membrane, known as the T-cell receptor (TCR). Each T cell expresses a single type of TCR and has the capacity to rapidly proliferate and differentiate if it receives the appropriate signals. As previously mentioned, T cells require the action of APCs (usually dendritic cells, but also macrophages, B cells, fibroblasts and epithelial cells) to recognize a specific antigen.
The surfaces of APCs express a group of proteins known as the major histocompatibility complex (MHC). MHC are classified as either class I (also termed human leukocyte antigen [HLA] A, B and C) which are found on all nucleated cells, or class II (also termed HLA DP, DQ and DR) which are found only on certain cells of the immune system, including macrophages, dendritic cells and B cells. Class I MHC molecules present endogenous (intracellular) peptides, while class II molecules on APCs present exogenous (extracellular) peptides to T cells. The MHC protein displays fragments of antigens (peptides) when a cell is infected with an intracellular pathogen, such as a virus, or has phagocytosed foreign proteins or organisms [ 2 , 3 ].
T cells have a wide range of unique TCRs which can bind to specific foreign peptides. During the development of the immune system, T cells that would react to antigens normally found in our body are largely eliminated. T cells are activated when they encounter an APC that has digested an antigen and is displaying the correct antigen fragments (peptides) bound to its MHC molecules. The opportunities for the right T cells to be in contact with an APC carrying the appropriate peptide MHC complex are increased by the circulation of T cells throughout the body (via the lymphatic system and blood stream) and their accumulation (together with APCs) in lymph nodes. The MHC-antigen complex activates the TCR and the T cell secretes cytokines which further control the immune response. This antigen presentation process stimulates T cells to differentiate primarily into either cytotoxic T cells (CD8+ cells) or T-helper (Th) cells (CD4+ cells) (see Fig. 2 ). CD8+ cytotoxic T cells are primarily involved in the destruction of cells infected by foreign agents, such as viruses, and the killing of tumour cells expressing appropriate antigens. They are activated by the interaction of their TCR with peptide bound to MHC class I molecules. Clonal expansion of cytotoxic T cells produces effector cells which release substances that induce apoptosis of target cells. Upon resolution of the infection, most effector cells die and are cleared by phagocytes. However, a few of these cells are retained as memory cells that can quickly differentiate into effector cells upon subsequent encounters with the same antigen [ 2 , 3 ].
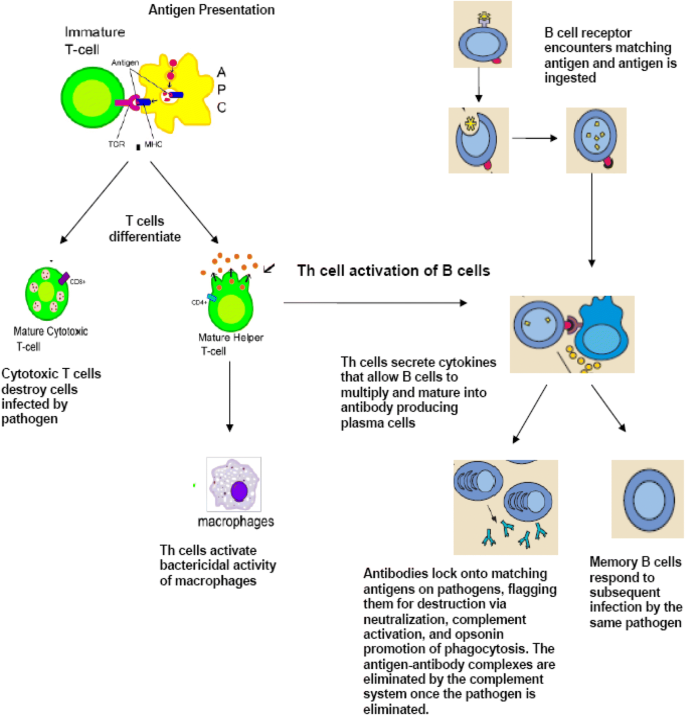
(figure adapted from images available at: http://en.wikipedia.org/wiki/Image:B_cell_activation.png and http://commons.wikimedia.org/wiki/Image:Antigen_presentation.svg )
Adaptive immunity: T-cell and B-cell activation and function. APC antigen-presenting cell, TCR T-cell receptor, MHC major histocompatibility complex
CD4+ Th cells play an important role in establishing and maximizing the immune response. These cells have no cytotoxic or phagocytic activity, and cannot directly kill infected cells or clear pathogens. However, they “mediate” the immune response by directing other cells to perform these tasks and regulate the type of immune response that develops. Th cells are activated through TCR recognition of antigen bound to class II MHC molecules. Once activated, Th cells release cytokines that influence the activity of many cell types, including the APCs that activate them.
Several types of Th cell responses can be induced by an APC, with Th1, Th2 and Th17 being the most frequent. The Th1 response is characterized by the production of IFN-γ which activates the bactericidal activities of macrophages and enhances anti-viral immunity as well as immunity to other intracellular pathogens. Th1-derived cytokines also contribute to the differentiation of B cells to make opsonizing antibodies that enhance the efficiency of phagocytes. An inappropriate Th1 response is associated with certain autoimmune diseases.
The Th2 response is characterized by the release of cytokines (IL-4, 5 and 13) which are involved in the development of immunoglobulin E (IgE) antibody-producing B cells, as well as the development and recruitment of mast cells and eosinophils that are essential for effective responses against many parasites. In addition, they enhance the production of certain forms of IgG that aid in combatting bacterial infection. As mentioned earlier, mast cells and eosinophils are instrumental in the initiation of acute inflammatory responses, such as those seen in allergy and asthma. IgE antibodies are also associated with allergic reactions (see Table 2 ). Therefore, an imbalance of Th2 cytokine production is associated with the development of atopic (allergic) conditions. Th17 cells have been more recently described. They are characterized by the production of cytokines of the IL-17 family, and are associated with ongoing inflammatory responses, particularly in chronic infection and disease. Like cytotoxic T cells, most Th cells will die upon resolution of infection, with a few remaining as Th memory cells [ 2 , 3 ].
A subset of the CD4+ T cell, known as the regulatory T cell (T reg), also plays a role in the immune response. T reg cells limit and suppress immune responses and, thereby, may function to control aberrant responses to self-antigens and the development of autoimmune disease. T reg cells may also help in the resolution of normal immune responses, as pathogens or antigens are eliminated. These cells also play a critical role in the development of “immune tolerance” to certain foreign antigens, such as those found in food.
B cells arise from hematopoietic stem cells in the bone marrow and, following maturation, leave the marrow expressing a unique antigen-binding receptor on their membrane. Unlike T cells, B cells can recognize antigens directly, without the need for APCs, through unique antibodies expressed on their cell surface. The principal function of B cells is the production of antibodies against foreign antigens which requires their further differentiation [ 2 , 3 ]. Under certain circumstances, B cells can also act as APCs.
When activated by foreign antigens to which they have an appropriate antigen specific receptor, B cells undergo proliferation and differentiate into antibody-secreting plasma cells or memory B cells (see Fig. 2 ). Memory B cells are “long-lived” survivors of past infection and continue to express antigen-binding receptors. These cells can be called upon to respond quickly by producing antibodies and eliminating an antigen upon re-exposure. Plasma cells, on the other hand, are relatively short-lived cells that often undergo apoptosis when the inciting agent that induced the immune response is eliminated. However, these cells produce large amounts of antibody that enter the circulation and tissues providing effective protection against pathogens.
Given their function in antibody production, B cells play a major role in the humoral or antibody-mediated immune response (as opposed to the cell-mediated immune response, which is governed primarily by T cells) [ 2 , 3 ].
Antibody-mediated vs. cell-mediated immunity
Antibody-mediated immunity is the branch of the acquired immune system that is mediated by B-cell-antibody production. The antibody-production pathway begins when the B cell’s antigen-binding receptor recognizes and binds to antigen in its native form. Local Th cells secrete cytokines that help the B cell multiply and direct the type of antibody that will be subsequently produced. Some cytokines, such as IL-6, help B-cells to mature into antibody-secreting plasma cells. The secreted antibodies bind to antigens on the surface of pathogens, flagging them for destruction through complement activation, opsonin promotion of phagocytosis and pathogen elimination by immune effector cells. Upon elimination of the pathogen, the antigen–antibody complexes are cleared by the complement cascade (see Fig. 2 ) [ 2 ].
Five major types of antibodies are produced by B cells: IgA, IgD, IgE, IgG and IgM. IgG antibodies can be further subdivided into structurally distinct subclasses with differing abilities to fix complement, act as opsonins, etc. The major classes of antibodies have substantially different biological functions and recognize and neutralize specific pathogens. Table 2 summarizes the various functions of the five Ig antibodies [ 5 ].
Antibodies play an important role in containing virus proliferation during the acute phase of infection. However, they are not generally capable of eliminating a virus once infection has occurred. Once an infection is established, cell-mediated immune mechanisms are most important in host defense against most intracellular pathogens.
Cell-mediated immunity does not involve antibodies, but rather protects an organism through [ 2 ]:
The activation of antigen-specific cytotoxic T cells that induce apoptosis of cells displaying foreign antigens or derived peptides on their surface, such as virus-infected cells, cells with intracellular bacteria, and cancer cells displaying tumour antigens;
The activation of macrophages and NK cells, enabling them to destroy intracellular pathogens; and
The stimulation of cytokine (such as IFNγ) production that further mediates the effective immune response.
Cell-mediated immunity is directed primarily at microbes that survive in phagocytes as well as those that infect non-phagocytic cells. This type of immunity is most effective in eliminating virus-infected cells and cancer cells, but can also participate in defending against fungi, protozoa, cancers, and intracellular bacteria. Cell-mediated immunity also plays a major role in transplant rejection.
Passive vs. active immunization
Acquired immunity is attained through either passive or active immunization. Passive immunization refers to the transfer of active humoral immunity, in the form of “ready-made” antibodies, from one individual to another. It can occur naturally by transplacental transfer of maternal antibodies to the developing fetus, or it can be induced artificially by injecting a recipient with exogenous antibodies that are usually manufactured for this purpose and that are targeted to a specific pathogen or toxin. The latter is used when there is a high risk of infection and insufficient time for the body to develop its own immune response, or to reduce the symptoms of chronic or immunosuppressive diseases.
Active immunization refers to the production of antibodies against a specific antigen or pathogen after exposure to the antigen. It can be acquired through either natural infection with a microbe or through administration of a vaccine that can consist of attenuated (weakened) pathogens, inactivated organisms or specific proteins or carbohydrates known to induce immunity. Effective active immunization often requires the use of “adjuvants” which improve the ability of the immune system to respond to antigen injection.
Immunopathology
As mentioned earlier, defects or malfunctions in either the innate or adaptive immune response can provoke illness or disease. Such disorders are generally caused by an overactive immune response (known as hypersensitivity reactions), an inappropriate reaction to self (known as autoimmunity) or ineffective immune responses (known as immunodeficiency).
Hypersensitivity reactions
Hypersensitivity reactions refer to undesirable responses produced by the normal immune system. There are four types of hypersensitivity reactions [ 6 , 7 ]:
Type I: immediate hypersensitivity.
Type II: cytotoxic or antibody-dependent hypersensitivity.
Type III: immune complex disease.
Type IV: delayed-type hypersensitivity.
Type I hypersensitivity is the most common type of hypersensitivity reaction. It is an allergic reaction provoked by re-exposure to a specific type of antigen, referred to as an allergen. Unlike the normal immune response, the type I hypersensitivity response is characterized by the secretion of IgE by plasma cells. IgE antibodies bind to receptors on the surface of tissue mast cells and blood basophils, causing them to be “sensitized”. Later exposure to the same allergen cross-links the bound IgE on sensitized cells resulting in degranulation and the secretion of active mediators such as histamine, leukotrienes, and prostaglandins that cause vasodilation and smooth-muscle contraction of the surrounding tissue. Common environmental allergens inducing IgE-mediated allergies include pet (e.g., cat, dog, horse) epithelium, pollen, house dust mites, and molds. Food allergens are also a common cause of type I hypersensitivity reactions, however, these types of reactions are more frequently seen in children than adults. Treatment of type I reactions generally involves trigger avoidance, and in the case of inhaled allergens, pharmacological intervention with bronchodilators, antihistamines and anti-inflammatory agents. Some types of allergic disease can be treated with immunotherapy (see Allergen-specific Immunotherapy article in this supplement). Severe cases of type 1 hypersensitivity (anaphylaxis) may require immediate treatment with epinephrine.
Type II hypersensitivity reactions are rare and take anywhere from 2 to 24 h to develop. These types of reactions occur when IgG and IgM antibodies bind to the patient’s own cell-surface molecules, forming complexes that activate the complement system. This, in turn, leads to opsonization, red blood cell agglutination (process of agglutinating or “clumping together”), cell lysis and death. Some examples of type II hypersensitivity reactions include: erythroblastosis fetalis, Goodpasture syndrome, and autoimmune anemias.
Type III hypersensitivity reactions occur when IgG and IgM antibodies bind to soluble proteins (rather than cell surface molecules as in type II hypersensitivity reactions) forming immune complexes that can deposit in tissues, leading to complement activation, inflammation, neutrophil influx and mast cell degranulation. This type of reaction can take days, or even weeks, to develop and treatment generally involves anti-inflammatory agents and corticosteroids. Examples of type III hypersensitivity reactions include systemic lupus erythematosus (SLE), serum sickness and reactive arthritis.
Unlike the other types of hypersensitivity reactions, type IV reactions are cell-mediated and antibody-independent. They are the second most common type of hypersensitivity reaction and usually take 2 or more days to develop. These types of reactions are caused by the overstimulation of T cells and monocytes/macrophages which leads to the release of cytokines that cause inflammation, cell death and tissue damage. In general, these reactions are easily resolvable through trigger avoidance and the use of topical corticosteroids. An example of this is the skin response to poison ivy.
A brief summary of the four types of hypersensitivity reactions is provided in Table 3 .
Autoimmunity
Autoimmunity involves the loss of normal immune homeostasis such that the organism produces an abnormal response to its own tissue. The hallmark of autoimmunity is the presence of self-reactive T cells, auto-antibodies, and inflammation. Prominent examples of autoimmune diseases include: Celiac disease, type 1 diabetes mellitus, Addison’s disease and Graves’ disease [ 8 ].
Inflammation
Poorly regulated inflammatory responses and tissue damage as a result of inflammation are often immunopathological features. Defects in immune regulation are associated with many chronic inflammatory diseases, including: rheumatoid arthritis, psoriasis, inflammatory bowel disease and asthma. Classical features of inflammation are heat, redness, swelling and pain. Inflammation can be part of the normal host response to infection and a required process to rid the body of pathogens, or it may become uncontrolled and lead to chronic inflammatory disease. The overproduction of inflammatory cytokines (such as TNF, IL-1 and IL-6) as well as the recruitment of inflammatory cells (such as neutrophils and monocytes) through the function of chemokines are important drivers of the inflammatory process. Additional mediators produced by recruited and activated immune cells induce changes in vascular permeability and pain sensitivity.
Immunodeficiency
Immunodeficiency refers to a state in which the immune system’s ability to fight infectious disease is compromised or entirely absent. Immunodeficiency disorders may result from a primary genetic defect (primary immunodeficiency—see Primary Immunodeficiency article in this supplement) which can effect either innate or acquired immune function through inhibition of selected immune cells or pathways, or it may be acquired from a secondary cause (secondary immunodeficiency), such as viral or bacterial infections, malnutrition, autoimmunity or treatment with drugs that induce immunosuppression. Certain diseases can also directly or indirectly impair the immune system such as leukemia and multiple myeloma. Immunodeficiency is also the hallmark of acquired immunodeficiency syndrome (AIDS), caused by the human immunodeficiency virus (HIV). HIV directly infects Th cells and also impairs other immune system responses indirectly [ 9 , 10 ].
Innate immunity is the first immunological, non-specific mechanism for fighting against infections. This immune response is rapid, occurring minutes or hours after aggression and is mediated by numerous cells including phagocytes, mast cells, basophils and eosinophils, as well as the complement system. Adaptive immunity develops in conjunction with innate immunity to eliminate infectious agents; it relies on the tightly regulated interplay between T cells, APCs and B cells. A critical feature of adaptive immunity is the development of immunologic memory or the ability of the system to learn or record its experiences with various pathogens, leading to effective and rapid immune responses upon subsequent exposure to the same or similar pathogens. A brief overview of the defining features of innate and adaptive immunity are presented in Table 4 .
There is a great deal of synergy between the adaptive immune system and its innate counterpart, and defects in either system can lead to immunopathological disorders, including autoimmune diseases, immunodeficiencies and hypersensitivity reactions. The remainder of this supplement will focus on the appropriate diagnosis, treatment and management of some of these more prominent disorders, particularly those associated with hypersensitivity reactions.
Abbreviations
pattern recognition receptors
pathogen associated molecular patterns
lipopolysaccharides
ribonucleic acid
tumour necrosis factor
interleukin
antigen-presenting cells
natural killer
interferon-gamma
innate lymphoid cells
T cell receptor
major histocompatibility complex
human leukocyte antigen
immunoglobulin
regulatory T cell
systemic lupus erythematosus
acquired immunodeficiency syndrome
human immunodeficiency virus
Turvey SE, Broide DH. Innate immunity. J Allergy Clin Immunol. 2010;125(Suppl 2):S24–32.
Article PubMed Google Scholar
Bonilla FA, Oettgen HC. Adaptive immunity. J Allergy Clin Immunol. 2010;125(Suppl 2):S33–40.
Murphy KM, Travers P, Walport M. Janeway’s immunobiology. 7th ed. New York: Garland Science; 2007.
Google Scholar
Stone KD, Prussin C, Metcalfe DD. IgE, mast cells, basophils, and eosinophils. J Allergy Clin Immunol. 2010;125(Suppl 2):S73–80.
Article PubMed PubMed Central Google Scholar
Schroeder HW, Cavacini L. Structure and function of immunoglobulins. J Allergy Clin Immunol. 2010;125(Suppl 2):S41–52.
Gell PGH, Coombs RRA. Clinical aspects of immunology. 1st ed. Oxford: Blackwell; 1963.
Rajan TV. The Gell-Coombs classification of hypersensitivity reactions: a re-interpretation. Trends Immunol. 2003;24:376–9.
Article CAS PubMed Google Scholar
Castro C, Gourley M. Diagnostic testing and interpretation of tests for autoimmunity. J Allergy Clin Immunol. 2010;125(Suppl 2):S238–47.
Notarangelo LD. Primary immunodeficiencies. J Allergy Clin Immunol. 2010;125(Suppl 2):S182–94.
Chinen J, Shearer WT. Secondary immunodeficiencies, including HIV infection. J Allergy Clin Immunol. 2010;125(Suppl 2):S195–203.
Download references
Declarations
Authors’ contributions All authors wrote and/or edited sections of the manuscript. All authors read and approved the final manuscript.
Acknowledgements
The authors would like to extend special thanks to Dr. Francesca Antonetti whose accredited online course entitled “An Introduction to Immunology” provided the foundation and framework for this article. This informative, entry-level course can be accessed through the Excellence in Medical Education (EXCEMED) website at: https://www.excemed.org .
This article is an update to the article entitled, An Introduction to Immunology and Immunopathology, that originally appeared in the supplement, Practical Guide to Allergy and Immunology in Canada, which was published in Allergy, Asthma & Clinical Immunology in 2011 (available at: https://aacijournal.biomedcentral.com/articles/supplements/volume-7-supplement-1 ).
The authors would like to thank Julie Tasso for her editorial services and assistance in the preparation of this manuscript.
Competing interests
Dr. Jean S. Marshall has no competing interests to disclose. Dr. Richard Warrington is the past president of the Canadian Society of Allergy & Clinical Immunology and Editor-in-Chief of Allergy, Asthma & Clinical Immunology. He has received consulting fees and honoraria from Nycomed, CSL Behring, Talecris, Grifols, Novartis and Shire. Dr. Wade Watson is an associate editor of Allergy, Asthma & Clinical Immunology. Dr. Harold Kim is Vice President of the Canadian Society of Allergy and Clinical Immunology, Past President of the Canadian Network for Respiratory Care, and Co-chief Editor of Allergy, Asthma and Clinical Immunology. He has received consulting fees and honoraria for continuing medical education from AstraZeneca, Aralez, Boehringer Ingelheim, CSL Behring, Kaleo, Merck, Novartis, Pediapharm, Sanofi, Shire and Teva.
Availability of data and materials
Data sharing not applicable to this article as no datasets were generated or analyzed during the development of this review.
Consent for publication
Not applicable.
Ethics approval and consent to participate
Ethics approval and consent to participate are not applicable to this review article.
Publication of this supplement has been supported by AstraZeneca, Boehringer Ingelheim, CSL Behring Canada Inc., MEDA Pharmaceuticals Ltd., Merck Canada Inc., Pfizer Canada Inc., Shire Pharma Canada ULC, Stallergenes Greer Canada, Takeda Canada, Teva Canada Innovation, Aralez Tribute and Pediapharm.
About this supplement
This article has been published as part of Allergy, Asthma & Clinical Immunology Volume 14 Supplement 2, 2018: Practical guide for allergy and immunology in Canada 2018. The full contents of the supplement are available online at https://aacijournal.biomedcentral.com/articles/supplements/volume-14-supplement-2 .
Publisher’s Note
Springer Nature remains neutral with regard to jurisdictional claims in published maps and institutional affiliations.
Author information
Authors and affiliations.
Department of Microbiology and Immunology, Dalhousie University, Halifax, NS, Canada
Jean S. Marshall
Section of Allergy & Clinical Immunology, Department of Internal Medicine, University of Manitoba, Winnipeg, MB, Canada
Richard Warrington
Division of Allergy, Department of Pediatrics, IWK Health Centre, Dalhousie University, Halifax, NS, Canada
Wade Watson
Western University, London, ON, Canada
Harold L. Kim
McMaster University, Hamilton, ON, Canada
You can also search for this author in PubMed Google Scholar
Corresponding author
Correspondence to Jean S. Marshall .
Rights and permissions
Open Access This article is distributed under the terms of the Creative Commons Attribution 4.0 International License ( http://creativecommons.org/licenses/by/4.0/ ), which permits unrestricted use, distribution, and reproduction in any medium, provided you give appropriate credit to the original author(s) and the source, provide a link to the Creative Commons license, and indicate if changes were made. The Creative Commons Public Domain Dedication waiver ( http://creativecommons.org/publicdomain/zero/1.0/ ) applies to the data made available in this article, unless otherwise stated.
Reprints and permissions
About this article
Cite this article.
Marshall, J.S., Warrington, R., Watson, W. et al. An introduction to immunology and immunopathology. Allergy Asthma Clin Immunol 14 (Suppl 2), 49 (2018). https://doi.org/10.1186/s13223-018-0278-1
Download citation
Published : 12 September 2018
DOI : https://doi.org/10.1186/s13223-018-0278-1
Share this article
Anyone you share the following link with will be able to read this content:
Sorry, a shareable link is not currently available for this article.
Provided by the Springer Nature SharedIt content-sharing initiative
- Pathogenic Intruders
- Rapid Immune Response
- COUNTERPARTS IN
- Adaptive Immunity
- Connective Tissue Surrounding Blood Vessels
Allergy, Asthma & Clinical Immunology
ISSN: 1710-1492
- General enquiries: [email protected]

An official website of the United States government
The .gov means it’s official. Federal government websites often end in .gov or .mil. Before sharing sensitive information, make sure you’re on a federal government site.
The site is secure. The https:// ensures that you are connecting to the official website and that any information you provide is encrypted and transmitted securely.
- Publications
- Account settings
The PMC website is updating on October 15, 2024. Learn More or Try it out now .
- Advanced Search
- Journal List
- Elsevier - PMC COVID-19 Collection

COVID-19 and the human innate immune system
Joachim l. schultze.
1 Systems Medicine, German Center for Neurodegenerative Diseases (DZNE), Bonn, Germany
2 PRECISE Platform for Single Cell Genomics and Epigenomics at the DZNE and the University of Bonn, Bonn, Germany
3 Genomics and Immunoregulation, Life and Medical Sciences (LIMES) Institute, University of Bonn, Bonn, Germany
Anna C. Aschenbrenner
4 Department of Internal Medicine and Radboud Center for Infectious Diseases (RCI), Radboud University Medical Center, Nijmegen, the Netherlands
The introduction of severe acute respiratory syndrome coronavirus 2 (SARS-CoV-2) into the human population represents a tremendous medical and economic crisis. Innate immunity—as the first line of defense of our immune system—plays a central role in combating this novel virus. Here, we provide a conceptual framework for the interaction of the human innate immune system with SARS-CoV-2 to link the clinical observations with experimental findings that have been made during the first year of the pandemic. We review evidence that variability in innate immune system components among humans is a main contributor to the heterogeneous disease courses observed for coronavirus disease 2019 (COVID-19), the disease spectrum induced by SARS-CoV-2. A better understanding of the pathophysiological mechanisms observed for cells and soluble mediators involved in innate immunity is a prerequisite for the development of diagnostic markers and therapeutic strategies targeting COVID-19. However, this will also require additional studies addressing causality of events, which so far are lagging behind.
Differences in innate immune system function may underpin the curiously variable disease spectrum of COVID-19.
Introduction
Coronavirus disease 2019 (COVID-19) ( Berlin et al., 2020 ; Gandhi et al., 2020 ), caused by severe acute respiratory syndrome coronavirus 2 (SARS-CoV-2) ( Kim et al., 2020 ; Wu et al., 2020 ; Yao et al., 2020 ; Zhang and Holmes, 2020 ) is a rather heterogeneous disease. Disease courses range from mainly asymptomatic and mild courses to more severe and critical courses in 10%–20% of symptomatic patients who are at considerable risk of fatality with many different organ systems involved in differing combinations and with variable symptoms ( Gupta et al., 2020 ) ( Box 1 ). The virus itself, the environment, and the host can contribute to such disease heterogeneity ( Morens and Fauci, 2020 ). Numerous mutations identified so far by thousands of viral sequences obtained during the first 9 months of the pandemic ( Candido et al., 2020 ; Oude Munnink et al., 2020 ) suggest that viral genetic diversity, genetic evolution, variable infectivity, or co-pathogenesis might contribute to infectivity and fatality, but not so much to disease heterogeneity observed for COVID-19. Although many mutations decreased infectivity, the virus variant D614G in the spike protein of SARS-CoV-2 increased infectivity ( Li et al., 2020 ). Two clades, recently emerged in the United Kingdom (B.1.1.7) and South Africa (B.1.1.351), similarly increased infectivity. Overall, however, there is little evidence for the virus to be mainly responsible for disease heterogeneity, which leaves the host and the environment as factors influencing disease course and outcome. Although there is evidence that environmental factors such as seasonality, other natural disasters, environmental degradation, quality of the public health infrastructure, or governance by state authorities have an impact on overall disease burden for society ( Han et al., 2020 ), the host itself seems to be the major factor explaining disease severity, infection rates ( Merad and Martin, 2020 ; Vabret et al., 2020 ), and long-term medical consequences ( Marshall, 2020 ). Disease severity and mortality rates are significantly higher in elderly populations pointing toward lack of widespread long-lasting pre-existing adaptive immunity by T or B cells against SARS-CoV-2 ( Morens and Fauci, 2020 ). This is in stark contrast to the 2009 influenza pandemic, which had much lower incidence rates in the elderly population, indicating partial protection on population level due to earlier exposure to similar influenza viruses ( Xu et al., 2010 ). It is possible that cross-protection via adaptive immune responses against endemic coronaviruses might be in part responsible for mild disease courses in younger individuals ( Lipsitch et al., 2020 ). An effective immune response against SARS-CoV-2 requires both arms of the immune system, the innate immune system ( Box 1 ) including granulocytes, monocytes, and macrophages among other cells of the innate immune system and the adaptive immune system with T and B cells (see Sette and Crotty [2021 ] for adaptive immunity against SARS-CoV-2). A role of the innate immune system in determining disease severity and outcome is supported by recent genetic findings ( van der Made et al., 2020 ; Severe Covid-19 GWAS Group et al., 2020 ; Zhang et al., 2020a ), interaction maps of viral proteins with host factors ( Gordon et al., 2020 ), or high resolution single-cell omics analyses ( Chua et al., 2020 ; Schulte-Schrepping et al., 2020 ). Here, we provide a conceptual framework for determining host-virus interactions of a pandemic threat with an emphasis on the innate immune system, highlight important findings about the innate immune system in COVID-19, and suggest avenues for further research on the most prioritized open questions.
Definition for disease severity, the innate immune system, and systems medicine
COVID-19 disease severity categories. In addition to national categories the WHO disease severity category is widely used. Here, the major categories are mild disease, moderate disease (mainly characterized by pneumonia), severe disease (with severe pneumonia), and critical disease (with acute respiratory distress syndrome [ARDS] and/or sepsis and/or septic shock). A number of other complications have been described, particularly for severe and critical disease such as acute pulmonary embolism, acute coronary syndrome, acute stroke or delirium among many others including Guillain-Barré syndrome.
Innate immune system, innate immune cells, and innate immunity. The immune system is divided into two arms, the adaptive immune system with T and B lymphocytes and the innate immune system with all other immune cell types of which granulocytes, monocytes, macrophages, and NK cells are the more common cells, but many other innate immune cells also exist including different dendritic cells, innate lymphoid cells, or mast cells. Although the majority of all species possess an innate immune system, the conventional adaptive immune system is restricted to jawed vertebrates. Whereas most immune responses involve both the innate immune system and the adaptive immune system evoking innate and adaptive immunity, respectively, the extent to which both arms are recruited to an immune response differs between stimuli.
Systems Medicine. In this review, we use the term Systems Medicine as the implementation of systems biology approaches in medical research and practice. A given hypothesis concerning e.g., the role of an organ system such as the immune system in a disease such as COVID-19 is answered by an iterative circular process between (I) clinical investigations, (II) computational multiscale modeling, followed by (III) experimental validation of (1) pathogenic mechanisms, (2) disease progression versus remission, (3) cure or disease spread, and (4) treatment responses and adverse events, both at the population and the individual patient level. High-resolution, high-throughput, and high-content technologies, particularly the omics technologies, are often used to reach the goals of Systems Medicine. The long-term goal of Systems Medicine is to provide measurable improvements for the patients’ health.
Conceptualizing the interaction between the host immune response and the virus
Looking at the current pandemic entirely from a biological perspective and assuming that the majority of humankind does not have an extended cross-reaction to other viruses of the coronavirus family ( Braun et al., 2020 ; Grifoni et al., 2020 ; Mateus et al., 2020 ), the introduction of SARS-CoV-2 into the human population is one of the biggest evolutionary events in the last hundred years ( Morens and Fauci, 2020 ; Morens et al., 2020 ). In such an evolutionary experiment, the innate immune system must be assigned a very special role in the defense against SARS-CoV-2 ( Amor et al., 2020 ; Mantovani and Netea, 2020 ; Vabret et al., 2020 ). An important part of innate immunity is the cell-autonomous response of the cells that get infected by the virus, which is influenced by the biology of the receptors and co-receptors for viral entry ( Hoffmann et al., 2020 ; Wu et al., 2020 ; Yao et al., 2020 ), as well as all cellular mechanisms that determine the viral life cycle ( Cyranoski, 2020 ). In the SARS-CoV-2 pandemic, the virus encounters the human population as a swarm of genetic variants and the processes of infection and virus replication may still be subject to major genetic changes, by which certain virus variants may achieve an evolutionary advantage. These processes are in full swing and accelerate as more individuals become infected ( Callaway, 2020 ; Cyranoski, 2020 ; Korber et al., 2020 ). It therefore will be important to monitor infected individuals for potential changes in the very early steps of the immune response to the virus, mainly triggered by infected cells and early interactions with adjacent innate immune cells.
Conceptually, when addressing important defense mechanisms of the host, it is important to consider all parameters that may be relevant for such an initial interaction of a new virus with a species ( Figure 1 ). Bearing in mind that most of the steps of the interaction between the virus and the host will follow a normal distribution of attributes or parameters required to describe the outcome of the interaction, it is not entirely surprising that the clinically observed disease courses show an enormous heterogeneity ( Berlin et al., 2020 ; Gandhi et al., 2020 ). For example, the induction of an interferon response by an infected cell might follow a normal distribution in the population with low, intermediate, and high responders thereby triggering different magnitudes of cellular responses and thus very different downstream effects (e.g., in the innate immune system). In Figure 1 , we illustrate important innate immune mechanisms that might be particularly prone to heterogeneous outcomes—due to both environmental and genetic factors—within the human population and therefore these should be a major focus in our efforts to determine the role of the innate immune system for infectivity, viral spreading, and disease course but also long-term outcome. We postulate that such conceptualization of the interplay between the innate immune system and the virus will help to focus on the most critical steps first, which then can be validated in larger studies.

Proposed fields of research along the disease trajectory in five phases that influence pathophysiology with an emphasis on innate immunity. Methodologies suggested to be applied for addressing certain areas are represented as color-coded circles. These reflect frequently used methods in previously published studies on COVID-19. This is only a selection and we make no claim to completeness. The overall concept could be extended to the adaptive immune system and other organ systems. CyTOF, cytometry by time of flight, mass cytometry; ELISA; OLINK, plasma proteome by proximity extension assay; scRNA-seq, single-cell RNA sequencing; seq, sequencing; WGS, whole genome sequencing.
As we will discuss, hypothesis-driven systems medicine approaches ( Box 1 ) have a very high chance to quickly uncover the most critical and variable steps in the interactions between the virus and the host’s immune system to link them to the different clinical phenotypes allowing a better stratification of patients that will foster the derivation of therapeutic procedures ( Rajewsky et al., 2020 ).
The breach—point of entry for SARS-CoV-2
Major determinants of the host’s innate immune response are dictated by the cell tropism of the virus and its ability to circumvent innate immune responses ( Morens and Fauci, 2020 ). With the identification of SARS-CoV-2 ( Hoffmann et al., 2020 ; Wu et al., 2020 ; Yao et al., 2020 ), and based on its close relationship with the SARS coronavirus (SARS-CoV) ( Coronaviridae Study Group of the International Committee on Taxonomy of Viruses, 2020 ), it became quickly clear that the surface receptor for SARS-CoV ( Li et al., 2003 ), the angiotensin-converting enzyme 2 (ACE2), was also a major cellular entry point for SARS-CoV-2 ( Hoffmann et al., 2020 ). Similar to SARS-CoV ( Matsuyama et al., 2010 ), SARS-CoV-2 employs the cellular serine protease TMPRSS2 for S protein priming. Most recently, Neuropilin1 (NRP1) was identified as an important cofactor for entry, particularly in cells with low level ACE2 expression ( Cantuti-Castelvetri et al., 2020 ). Several other proteases including Furin have also been indicated as co-factors ( Ou et al., 2020 ).
In absence of cell-type-specific expression maps of SARS-CoV-2 viral entry-associated genes, the Human Cell Atlas (HCA) Lung Biological Network investigated the prevalence of ACE2 and TMPRSS2 in the body by analyzing their expression in single-cell RNA sequencing data (scRNA-seq) from multiple tissues from healthy human donors ( Sungnak et al., 2020 ). Probably most important for the high efficacy of SARS-CoV-2 transmission is the high co-expression of ACE2 and TMPRSS2 in nasal epithelial cells, particularly in two types of goblet cells and a subset of ciliated cells, showing the highest expression among all cells in the respiratory tree ( Figure 2 A). An interesting observation of this study was the high co-expression of genes involved in early steps of antiviral immune responses in these epithelial cells, which indicated the potential for these specialized nasal cells to play an important role in the initial viral infection, spread, but potentially also, clearance. In the lung, ACE2 and TMPRSS2 expression has been mainly identified in alveolar epithelial type II cells ( Qi et al., 2020 ; Zou et al., 2020 ). TMPRSS2 is more widely expressed than ACE2 , and cells with simultaneous expression were identified in the respiratory tree, the cornea, esophagus, ileum, colon, gallbladder, and common bile duct ( Sungnak et al., 2020 ), yet there was no evidence for simultaneous expression in immune cells. Extending these studies to even larger datasets, including dozens of different scRNA-seq datasets derived from many groups around the world, revealed the power of metadata analysis based on scRNA-seq ( Muus et al., 2021 ). Here, it was even possible to predict the impact of clinical risk factors such as sex, age, and smoking history among others on gene expression level of genes associated with viral entry. A second study from the HCA Lung Biological Network extended the initial findings to tissues from non-human primates and mice, indicating co-expression of ACE2 and TMPRSS2 within nasal goblet secretory cells, lung type II pneumocytes, and ileal absorptive enterocytes ( Ziegler et al., 2020 ). In contrast to earlier reports suggesting ACE2 to be an interferon-stimulated gene (ISG), more recent evidence showed that a truncated form of ACE2 designated deltaACE2 , but not ACE2 itself, is an ISG ( Onabajo et al., 2020 ). DeltaACE2 neither binds the SARS-CoV-2 spike protein nor serves as a carboxypeptidase.

SARS-CoV-2 tropism, infection, and alarming the innate immune system
(A) Major entry sites of SARS-CoV-2 via cells within the nasal cavity and the upper and lower respiratory tract.
(B) Molecular determinants during SARS-CoV-2 infection of a cell.
(C) SARS-CoV-2 is most likely recognized by PRRs recognizing foreign RNA including endosomal TLR3 and TLR7 as well as by cytoplasmic RIG-I and MDA5. Predicted downstream signaling events based on findings from genetic studies, functional and clinical observations, interaction mapping, or CRISPR screens. Interactions between SARS-CoV-2-derived proteins and cellular mechanisms or in case of interaction mapping derived information, detection of direct interaction between viral or host proteins. ORF3b was functionally determined to suppress type I IFN, but a direct target was not identified ( Konno et al., 2020 ). ACE2, angiotensin-converting enzyme 2; IFNAR1, interferon-alpha/beta receptor alpha chain; IκB, inhibitor of κB; IKKα/ꞵ/γ/ε, IκB kinase α/ꞵ/γ/ε; IRAK1/4, interleukin-1 receptor-associated kinase 1/4; IRF3/7/9, interferon regulatory factor 3/7/9; ISG, interferon-stimulated genes; MDA5, melanoma differentiation-associated protein 5, RIG-I-like receptor dsRNA helicase enzyme; MyD88, myeloid differentiation primary response 88; NAP1, NF-κB-activating kinase-associated protein 1; NRP1, neuropilin 1; NSP, non-structural proteins of SARS-CoV-2; ORF, open reading frames of SARS-CoV-2; p50/65, the two subunits of NF-κB; RIG-1, retinoic acid-inducible gene I, a cytoplasmic pattern recognition receptor recognizing double-stranded RNA; RIP1, receptor interacting serine/threonine kinase 1; SARS-CoV-2, severe acute respiratory syndrome coronavirus 2; STAT1/2, signal transducer and activator of transcription 1/2; TAB1/2, TGF-beta activated kinase 1 binding protein 1/2; TAK1, TGF-beta activated kinase (encoded by MAP3K7 ); TBK1, TANK-binding kinase 1; TLR3, Toll-like receptor 3, CD283; TLR7/8, complex of Toll-like receptors 7 and 8, TLR7 also known as CD287 and CD288, respectively; TMPRSS2, transmembrane protease, serine 2; TRAF3/6, TNF receptor-associated factor 3/6; TRIF, TIR-domain-containing adaptor-inducing interferon-β; TYK2, tyrosine kinase 2; UNC93B1, Unc-93 homolog B1.
By applying a newly developed computational pipeline called Viral-Track, viral RNA was detected in single-cell transcriptomes of bronchoalveolar lavage fluid (BALF) samples from COVID-19 patients with severe, but not mild, disease course ( Bost et al., 2020 ), suggestive of a differential progression of infection in the lung. The strongest enrichment of viral RNA was observed in ciliated and epithelial progenitors. Viral RNA was also detected in a subset of macrophages ( SPP1 + macrophages) ( Bost et al., 2020 ; Chua et al., 2020 ). Whether these myeloid cells are directly infected or whether these cells phagocytosed cellular material carrying viral RNA requires further investigation. In vitro monocyte-derived macrophages and dendritic cells (DCs) are susceptible to SARS-CoV-2 infection, which is abortive, because these cells do not support virus replication ( Yang et al., 2020a ). Interestingly, as it has also been suggested that potential co-infections might determine the clinical phenotype and disease outcome, co-infection of monocytes in the BALF with human metapneumovirus was identified in one of the severe COVID-19 patients ( Bost et al., 2020 ), and it was postulated that such co-infections might explain—at least in part—critical COVID-19 disease courses. However, much larger studies are urgently needed to verify these important, but still preliminary, findings.
Information about the role of innate immune cells as potential targets of SARS-CoV-2 or mediating cells for the infection in organs or tissues other than the respiratory tract is still sparse. Within the oral cavity, there is little evidence for expression of ACE2, TMPRSS2, or Furin on any other cells than epithelial cells ( Sakaguchi et al., 2020 ). Furthermore, there has been no evidence for the role of innate immune cells in SARS-CoV-2 gaining access to the retina ( de Figueiredo et al., 2020 ) or the brain ( Song et al., 2021 ), albeit the virus has been found in both organs. Similarly, the human placenta is highly susceptible to SARS-CoV-2 infection, but only non-immune cells including syncytiotrophoblasts in the first trimester and extravillous trophoblasts in the second trimester express ACE2 and TMPRSS2 and have been identified as cellular targets for the virus ( Ashary et al., 2020 ). Interestingly, other coronavirus family member receptors (ANPEP and DPP4) and several proteases (CTSL, CTSB, and Furin) are uniformly expressed within the placenta throughout the first and second trimester, and genes necessary for viral budding and replication were also identified, strongly suggesting that the placenta is a vulnerable target for productive infection ( Ashary et al., 2020 ). Yet, based on clinical observations, there is no evidence for vertical transmission of the virus from infected mothers to the fetus ( Islam et al., 2020 ) arguing for a protective role of the maternal immune response, which requires further exploitation.
An interesting finding is the expression of ACE2 and TMPRSS2 on platelets, considering that patients present with signs of platelet hyperactivation, particularly in severe COVID-19 ( Zhang et al., 2020b ). In vitro exposure to SARS-CoV-2 or its Spike protein potentiated platelet aggregation, release of dense granules, upregulation of activation markers (PAC-1, CD62P), platelet spreading, and clot retraction. Furthermore, the virus facilitates the release of coagulation factors, the secretion of inflammatory cytokines, and the formation of leukocyte-platelet aggregates, which might be important mechanisms leading to thromboembolic complications observed in severe COVID-19 ( Ackermann et al., 2020 ; Gupta et al., 2020 ; Zhang et al., 2020b ). Whether other receptors including CD147 (BSG) can facilitate viral entry remains to be seen. CD147 is widely expressed and receptor expression and viral load does not seem to correlate ( Bost et al., 2020 ) arguing against CD147 as a major entry receptor for SARS-CoV-2.
Collectively, there is still little evidence that innate immune cells are a primary target of productive infection of SARS-CoV-2. The detection of viral RNA in myeloid cells derived from lung or BALF of severe COVID-19 patients still allows several explanations, including phagocytosis of cellular material derived from infected cells, which requires further assessment. Similarly unclear is whether platelets in the blood might serve as a sponge for viruses, which could explain why viruses are rarely detected in blood specimens, yet seem to infect many organs.
Cytokine alterations and innate immune cell responses following infection
Alarming the immune system—recognition, interferon response, and immune evasion.
A common pattern of cell entry via endocytosis following receptor binding was established for coronaviruses, which is followed by viral RNA release into the cytoplasm, production of viral proteins, and formation of viral replication/transcription complexes on double-membrane vesicles ( Snijder et al., 2020 ). Although viral entry mechanisms are well established for SARS-CoV-2 ( Shang et al., 2020 ), the following steps are not yet entirely resolved. Similarly, the pattern recognition receptors (PRRs) involved in recognizing SARS-CoV-2 are not yet directly determined. Based on findings from other coronaviruses, the most likely candidates are Toll-like receptors 3 (TLR3) and TLR7 in the endosome ( Mazaleuskaya et al., 2012 ) or the cytosolic sensors retinoic acid-inducible gene 1 (RIG-I) and melanoma differentiation-associated gene 5 (MDA5) ( Sa Ribero et al., 2020 ) recognizing foreign viruses’ RNA ( Figure 2 B). All these PRRs are linked via signaling cascades to induce strong interferon responses. RIG-I and MDA5 trigger the downstream adaptor mitochondrial antiviral signaling protein (MAVS) on mitochondria followed by activation of tumor necrosis factor receptor-associated factor 3 (TRAF3), TRAF family member-associated nuclear factor κB (NF-κB) activator binding kinase 1 (TBK1), together with inhibitor of NF-κB kinase-ε (IKK), which leads to phosphorylation of the “master regulators” IRF3 and IRF7. Subsequently, transcription of type I IFNs and numerous ISGs are induced ( Loo and Gale, 2011 ). Similar signaling cascades are known downstream of TLR3 and TLR7 ( Figure 2 C). Secreted type I IFNs signal via interferon receptors (IFNAR) to switch on Janus kinase 1 (JAK1) and tyrosine kinase 2 (Tyk2), activating STAT1, STAT2, and IRF9, which form a complex and translocate to the nucleus to bind to interferon-stimulated response elements (ISREs) in the genome. Hundreds of ISGs with various antiviral functions get induced, which can lead to further amplification loops by ISGs. Importantly, the type I IFN response can be rather heterogeneous. Depending on the state of the reacting cell (e.g., with respect to its metabolic or general activation state) ( Talemi and Höfer, 2018 ), it is at least in part stochastic ( Rand et al., 2012 ; Wimmers et al., 2018 ), it varies among different cell types and microenvironments, and it shows interindividual heterogeneity concerning the amplitude and kinetics ( Patil et al., 2015 ). The ability to fine-tune an IFN response is critical because both its overactivation and underactivation are deleterious to the host ( Mesev et al., 2019 ). The role of these fine-tuning mechanisms has not yet been addressed in detail in context of COVID-19.
Keeping the complex regulation of the IFN system in mind, it is critical to understand the dynamics of the type I IFN response in COVID-19. In in vitro infection models utilizing cell lines, SARS-CoV-2 induced only low type I and II IFNs, consequently inducing only moderate levels of ISGs and a unique proinflammatory cytokine signature including IL1B , IL6 , and TNF , as well as many chemokines ( CCL20 , CXCL1 , CXCL2 , CXCL3 , CXCL5 , CXCL6 , and CXCL16 ) ( Blanco-Melo et al., 2020 ). An early study in patients reported that type I IFNs were either not detected (particularly IFNβ) irrespective of disease severity or at lower levels (IFNα) in plasma mainly derived from patients with severe disease ( Hadjadj et al., 2020 ). These observations were further extended by illustrating a lack of expression of genes encoding type I IFNs in peripheral blood mononuclear cells (PBMCs) of COVID-19 patients and an early but transient wave of ISG expression in blood-derived immune cells, which correlated with an early burst of IFNα, likely of lung origin ( Arunachalam et al., 2020 ). An early transient wave of ISG expression in cells of the innate immune system, particularly monocytes, was also determined by scRNA-seq ( Schulte-Schrepping et al., 2020 ). A longitudinal analysis confirmed an early peak with subsequent decline of IFNα and IFNλ in mild-to-moderate COVID-19, whereas levels further increased particularly during the second week in severe patients ( Lucas et al., 2020 ). The dynamics of type I IFNs in severe patients is in line with findings in a murine model of SARS-CoV-2 infection illustrating that type I IFNs do not control SARS-CoV-2 replication in vivo but are significant drivers of pathologic responses ( Israelow et al., 2020 ).
As an immune evasion strategy, SARS-CoV-2 uses a multipronged strategy to antagonize the IFN system with the clinical consequence of an insufficient type I IFN response having been recognized in early COVID-19 studies ( Acharya et al., 2020 ; Blanco-Melo et al., 2020 ; Sa Ribero et al., 2020 ). At least ten SARS-CoV-2 proteins have been identified that counteract the antiviral action of IFN ( Figure 2 C). SARS-CoV-2-derived non-structural protein 16 (NSP16) suppresses global mRNA splicing and diminishes recognition of viral RNA by intracellular helicase receptors, NSP1 leads to global inhibition of mRNA translation by binding to the 18S ribosomal RNA in the mRNA entry channel, and NSP8 and NSP9 interfere with protein trafficking to the cell membrane; all three mechanisms independently leading to reduction of type I IFN production by the affected cell ( Banerjee et al., 2020 ; Gordon et al., 2020 ). Interaction mapping further suggested that NSP13 interacts with TBK1, NSP15 with NRDP1 (an E3 ubiquitin ligase controlling the balance between JAK2-associated cytokine receptor degradation and ectodomain shedding), ORF9b with TOMM70 (an important receptor of the outer mitochondrial membrane), and ORF6 with either KPNA2 or the IFN-induced NUP98-RAE1 nuclear export complex, which are both involved in nuclear translocation of proinflammatory transcription factors such as IRF3, IRF7, or STAT1, a mechanism targeted by numerous other viruses including influenza, all antagonizing IFN signaling ( Gordon et al., 2020 ; Xia et al., 2020 ). Functional testing further supported the counteracting activity of ORF3b, ORF6, NSP1, or NSP13 on type I IFN activation, of which particularly ORF6 suppressed STAT1 and STAT2 phosphorylation and STAT1 nuclear translocation, ( Konno et al., 2020 ; Lei et al., 2020 ; Xia et al., 2020 ).
Accumulating evidence indicates that SARS-CoV-2 is targeting the type I IFN system at multiple steps thereby strongly interfering with a well-orchestrated interplay between antiviral and proinflammatory innate and adaptive defense mechanisms within the immune system. Studies with more in-depth longitudinal profiling and stratification by disease severity are warranted to clarify the questions surrounding the nature of the early IFN response to SARS-CoV-2. Further dissecting this delicate balance at the early steps of a SARS-CoV-2 infection for each patient individually might be critical to better stratify patients and to allow for patient-tailored treatment options thereby preventing the development of immunopathology as the basis for severe disease courses ( Lee and Shin, 2020 ).
The next phase—from “cytokine storm” to immunosuppression
Conceptually, soluble factors including cytokines, chemokines, growth factors, inhibitory factors, hormones, and metabolites influence innate immune cells locally and systemically. Innate immune cells serve both as sender and receiver for soluble mediators. Soluble factors are important mediators shaping disease courses by triggering the release of innate immune cells from the bone marrow into circulation, their recruitment to inflamed and infected tissues, as well as by influencing their local motility and function. The combination, as well as the timing (kinetics) and the concentrations (dynamics) of these factors, guides cellular responses and interactions in COVID-19 ( Figure 3 ).

Soluble mediators during the disease course of COVID-19
Summarizing the principles established by many observational studies addressing the role of soluble mediators of the innate immune system. In red color severe (critical and fatal) disease courses, in yellow mild to moderate disease courses. (1) The term “days from symptom onset” is increasingly used to determine the kinetics of the disease. For this term, it needs to be considered that there exists variance in interpretation as well as in the time from infection to first symptoms, which is illustrated by the fading colors in the graph. (2 and 3) Temporal phases of COVID-19 have not been defined equally in all studies. However, in the majority of studies, “early” (2) describes the period up to 10 days after onset of symptoms, and “late” (3) the period from approximately days 11–25 after onset of symptoms. (4) Admission to hospital had been used initially, because it is a concrete data point. However, the time from viral infection to hospital admission can vary significantly, which complicates comparability of longitudinal studies using admission to hospital as the starting point. (5) Viral detection levels over time summarized following findings reported in Lucas et al. (2020) . (6) The early local innate immune responses are very difficult to capture in a clinical setting since the time of viral infection is not known for most patients. Evidence from model systems indicate that type I and III IFN responses are most important; however, the magnitude and exact kinetic of this response in severe versus mild COVID-19 is still under debate. (7) Local innate immune responses (mainly in upper and lower respiratory tract). In addition to chemokines, some reports also mention proinflammatory mediators. Data for later time points are still rare. (8–10) Most often mentioned soluble markers in plasma or serum of patients with mild (8), moderate to severe (9) disease, or critically ill COVID-19 patients (including fatal outcomes) (10), mainly at the end of the first week and the beginning of the second week, at the end of the first week and the beginning of the second week, and at later time points, respectively. Asymptomatic SARS-CoV-2 infections have been shown to exhibit lower levels of cytokines ( Long et al., 2020 ). Depending on study design and methodology, not all markers have been measured in all studies and at all time points.
Early on, it was suggested that COVID-19, particularly severe disease courses, might be associated with a maladapted induction of an immune response to the infection leading to what has been previously termed a “cytokine storm” or cytokine release syndrome (CRS) ( Mulchandani et al., 2021 ; de la Rica et al., 2020 ). Summarizing many observational studies, a current model suggests that SARS-CoV-2 enters type II pneumocytes via ACE2 in the respiratory system leading to rapid virus replication as well as concomitantly to a proinflammatory state with elevated levels of cytokines such as IL-1, IL-6, CXCL8, and TNF ( Cao, 2020 ; Lucas et al., 2020 ; Wang et al., 2020a ). This finding was recapitulated in vitro in monocyte-derived macrophages, but not DCs, suggesting that these cells might be a major source of the proinflammatory cytokines ( Yang et al., 2020a ). Proinflammatory genes upregulated in innate immune cells in severe or critical patients mainly belonged to the NF-κB pathway ( Hadjadj et al., 2020 ). Accumulation of NF-κB-dependent proinflammatory mediators can provoke an accumulation of pathogenic inflammatory neutrophils and macrophages in the lung, further perpetuating higher proinflammatory cytokines and chemokines in BALF (CCL2, CCL3, CCL4, and CXCL10) ( Xiong et al., 2020 ) but also in the circulation (IL-1, IFNγ, IL-17, TNF, IP-10, MCP-1, G-CSF, GM-CSF, IL-1RA, CCL2, CCL3, CCL5, CCL8, CXCL2, CXCL8, CXCL9, and CXCL16) ( Blanco-Melo et al., 2020 ; Hadjadj et al., 2020 ; Huang et al., 2020 ; Wang et al., 2020a ; Wu and Yang, 2020 ; Yang et al., 2020b ). This was particularly prominent during the second week after disease onset and more pronounced in severe patients ( Lucas et al., 2020 ), resulting in an excessive inflammatory and immune response, especially in the lungs, leading to acute respiratory distress syndrome (ARDS), pulmonary edema, apoptosis of epithelial cells, and finally vascular damage and multi-organ failure ( Berlin et al., 2020 ; Gandhi et al., 2020 ). The elevation of cytokines in severe disease is accompanied by elevated clinical laboratory parameters including alanine aminotransferase, lactate dehydrogenase, C-reactive protein (CRP), ferritin, and D-dimers. Bioactivity of some of these mediators of inflammation, particularly IL-6 and TNF, has been validated by transcriptomics in blood-derived cells, demonstrating corresponding elevated IL-6 and TNF response signatures in circulating immune cells ( Hadjadj et al., 2020 ). Inflammatory cell death in this hyperinflammatory state has been attributed to the concomitant elevation of TNF and IFNγ as being sufficient to trigger pyroptosis, apoptosis, and necrosis (PANoptosis) driving tissue damage and mortality in severe COVID-19 ( Karki et al., 2021 ). Simultaneous upregulation of IFNγ and IL-10 with higher IFNγ levels, particularly in mild patients, is another hallmark of COVID-19 ( Hadjadj et al., 2020 ).
The term cytokine storm has been quickly used to describe the immunopathology in severe COVID-19. Critical voices have since raised concerns whether cytokine storm or CRS reflects the immune response in COVID-19 patients correctly ( Sinha et al., 2020 ), noting that the observed elevated IL-6 levels are considerably lower than e.g., in septic shock or CRS due to other causes ( Kox et al., 2020 ; Monneret et al., 2021 ). Yet, longitudinal profiling also revealed dynamic changes in concentration of many cytokines including IL-6 ( Lucas et al., 2020 ), highlighting the importance of temporal examination of the pathological features of this new disease. On the other hand, the definition of cytokine storm has expanded to conditions beyond sepsis and, irrespective of absolute cytokine concentrations, to include all inflammatory conditions with elevated circulating cytokines that result in systemic inflammation as well as secondary organ dysfunction ( Fajgenbaum and June, 2020 ; Mangalmurti and Hunter, 2020 ), including COVID-19. One further hallmark of immune hyperactivation in cytokine storm is the failure of resolution of the inflammation ( Fajgenbaum and June, 2020 ). Considering the coexistence of CRS in severe disease with immunosuppressive innate immune cells ( Remy et al., 2020 ; Schulte-Schrepping et al., 2020 ), the exact terminology of immunopathology in severe COVID-19 still warrants further investigation. Complex viral sepsis with immune deviation has been proposed as a current alternative description ( Riva et al., 2020 ).
Children often experience no or only mild symptoms on SARS-CoV-2 infection, but can, in rare cases, present with multisystem inflammatory syndrome in children (MIS-C) 1–2 months after infection. Symptoms such as elevated inflammatory markers, fever, and multi-organ dysfunction bear overlap to acute, severe COVID-19 in adults or also Kawasaki disease, a pediatric vasculitis, however, MIS-C has distinct immunological features different from the two with respect to circulating cytokine profiles and composition of the T cell compartment ( Carter et al., 2020 ; Consiglio et al., 2020 ; Gruber et al., 2020 ). Although the pathogenesis is still unknown, the appearance of autoantibodies has been reported ( Consiglio et al., 2020 ; Gruber et al., 2020 ).
Apart from the classical chemokines and cytokines, soluble mediators present in patients’ circulation have been investigated in omics approaches to identify systemic effectors indicating novel aspects of severe COVID-19 pathology and to find novel biomarkers and/or therapeutically targetable factors. Proteomic and metabolomic studies in plasma also revealed molecules previously reported to be linked to immunosuppression, such as kynurenines, are elevated in severe disease whereas numerous lipids were reduced ( Shen et al., 2020 ; Su et al., 2020 ). Whether kynurenines and other potentially immunosuppressive molecules indeed exert such function in COVID-19 requires further functional testing. The profound loss of lipids, especially sphingolipids, glycerophospholipids, cholines, and derivatives, might contribute to loss of immune function of innate immune cells, particularly in monocyte-derived cells, such as signal transduction, growth regulation, cytokine secretion, cell migration, adhesion, apoptosis, senescence, and inflammatory responses. Proteins involved in fatty acid metabolism such as FETUB and CETP, known to suppress inflammation, were reduced in serum, as was PI6, a suppressor of chemotaxis ( Shu et al., 2020 ). Another important class of mediators is the acute phase proteins (APPs), which are involved in early states of immune responses to viral infections. In addition to CRP and the alarmins S100A8/A9 ( Schulte-Schrepping et al., 2020 ; Silvin et al., 2020 ), the serum amyloids A-1 (SAA1), A-2 (SAA2), and A-4 (SAA4), the serum amyloid P-component (SAP/APCS), and alpha-1-antichymotrypsin (SERPINA3) were found to be elevated in severe COVID-19 ( Shen et al., 2020 ; Shu et al., 2020 ). Similarly, the elevation of components and regulators of the complement system (complement 6, complement factor B, properdin, and carboxypeptidase N catalytic chain) point toward complement activation as an early innate immune activation mechanism ( Shen et al., 2020 ). In addition to thrombocytopenia, the platelet-derived chemokines proplatelet basic protein (PPBP; also called macrophage-derived growth factor) and platelet factor 4 (PF4) showed reduced levels in COVID-19 ( Shen et al., 2020 ). Another interplay between metabolite regulation and immune mediators in COVID-19 might occur due to interferon-mediated reduction of amino acid metabolism ( Shen et al., 2020 ).
Collectively, all these findings support a much more complex host-pathogen interaction, particularly in severe COVID-19, which is better summarized as viral sepsis, with the upregulation of proinflammatory mediators being only one part of a derailed innate immune response.
Changes in the innate immune cell compartment
Recognition of the viral infection by tissue-resident immune cells results in a local innate immune response leading to the recruitment of further innate immune cells with the intent to initiate viral clearance ( Figure 4 ). Handling of the infection in the upper respiratory tract (the major entry point of SARS-CoV-2), managing viral clearance, and consequent resolution of the active immune response, is a key aspect for prevention of viral dissemination into the lung and pulmonary injury ( Newton et al., 2016 ).

Cellular changes during COVID-19
Depicted are the COVID-19-associated changes for the innate immune cells stratified by common disease characteristics (middle column) or such associated with either mild or severe disease courses, as well as stratified by location (local versus systemic) and time. ISG, interferon response gene; Mac, macrophage; mDC, myeloid dendritic cell; NKG2A, NK cell receptor (CD159a) encoded by killer cell lectin lectin-like receptor C1, KLRC1; pDC, plasmacytoid dendritic cell; SPP1, secreted phosphoprotein 1 (coding for osteopontin); TGF-B2, transforming growth factor beta 2; TIM3, T cell immunoglobulin and mucin domain-containing 3 (also known as hepatitis A virus cellular receptor 2, HAVCR2); TREM2, triggering receptor expressed on myeloid cells 2.
The local mucosal immune response to SARS-CoV-2 infection has been characterized by cellular and transcriptional changes in the upper respiratory tract ( Chua et al., 2020 ) as well as in BALF assessing the lower, more distal parts of the lung ( Liao et al., 2020 ). COVID-19 patients, in general, showed the expected influx of innate immune cells, particularly neutrophils and monocytes into the nasopharyngeal mucosa on chemokine secretion of the infected epithelial cells (e.g., CXCL1 , CXCL3 , CXCL6 , CXCL15 , CXCL16 , and CXCL17 ). Severe patients expressed elevated levels of chemokines and chemokine receptors and exhibited a marked increase in neutrophils in the tissue ( Chua et al., 2020 ). Further, proinflammatory macrophages were identified in the lungs of critical patients to possibly contribute to excessive inflammation by promoting further granulocyte and monocyte recruitment and differentiation ( Chua et al., 2020 ; Liao et al., 2020 ). Because no further functional analysis was performed, it remains to be determined whether these activated infiltrating monocyte-derived macrophages in addition to recruited neutrophils in critically ill patients are capable of exerting typical functions of phagocytic cells at the viral entry sites and/or to what extent they may contribute to tissue damage. Furthermore, because immune cells in circulation were not studied from the same patients, it also remains to be seen whether changes observed in the circulation are reflective of patient-individual changes in organs with known high viral load and infection such as the lung.
Elevated neutrophil and natural killer (NK) cell numbers, as well as lower proportions of dendritic cells and T cells, were a feature of BALF from severe patients, whereas clonally expanded CD8 + T cells were found in such samples from moderate patients ( Liao et al., 2020 ). Further dissection of the increased monocyte/macrophage compartment in severe patients revealed macrophage subpopulations showing immunoregulatory features indicative of resolution, yet also showing expression of the profibrotic genes TREM2 , TGFB2 , and SPP1 ( Liao et al., 2020 ).
The initial local respiratory SARS-CoV-2 infection entails alterations in the circulating cells in the blood with particular changes in the innate compartment. An increase in circulating neutrophils versus a decrease in lymphocytes has been appreciated as a hallmark of severe COVID-19 ( Hadjadj et al., 2020 ; Mathew et al., 2020 ; Qin et al., 2020 ; Schulte-Schrepping et al., 2020 ).
Mild COVID-19 patients were marked by circulating HLA-DR hi CD11c hi inflammatory monocytes with an interferon-stimulated gene signature, while noticeable amounts of circulating neutrophil precursors appeared in severe cases, indicative of emergency myelopoiesis, as well as the presence of dysfunctional, CD274 (PD-L1)-expressing mature neutrophils ( Schulte-Schrepping et al., 2020 ). Further, loss of non-classical monocytes as well as the appearance of dysfunctional S100 hi classical monocytes with downregulated MHC class II expression were associated with severe COVID-19, reminiscent of immunoparalysis seen in sepsis ( Arunachalam et al., 2020 ; Giamarellos-Bourboulis et al., 2020a ; Kuri-Cervantes et al., 2020 ; Schulte-Schrepping et al., 2020 ; Silvin et al., 2020 ; Su et al., 2020 ; Wilk et al., 2020 ). Further, elevated levels of megakaryocytes and erythroid cells were identified in severe COVID-19, and the associated expression modules correlated with mortality ( Bernardes et al., 2020 ). Neutrophils in COVID-19 patients showed expression profiles associated with activation and neutrophil extracellular trap (NET) formation ( Aschenbrenner et al., 2021 ; Schulte-Schrepping et al., 2020 ) as well as increased NET formation ex vivo ( Middleton et al., 2020 ). These findings go hand in hand with the thrombotic complications and coagulopathy frequently found in severe COVID-19 ( Klok et al., 2020 ), because NET formation can seed immunothrombosis ( Veras et al., 2020 ), and platelets and neutrophils can interact to promote thrombus formation ( Pircher et al., 2019 ). Interestingly, plasmacytoid DC (pDCs), the main source of IFNα, were not only reduced in abundance in the circulation ( Kuri-Cervantes et al., 2020 ) but also impaired in function in COVID-19 patients suggesting the lung as a source for the elevated plasma levels of type I IFNs early on infection ( Arunachalam et al., 2020 ; Lucas et al., 2020 ). Single-cell transcriptomics of circulating innate immune cells strongly support a general IFN response in all COVID-19 patients with a strong temporal component ( Lee et al., 2020 ; Lucas et al., 2020 ; Schulte-Schrepping et al., 2020 ; Wilk et al., 2020 ).
In addition to an increase in circulating neutrophils, lymphopenia has been identified as a hallmark of severe COVID-19 ( Guan et al., 2020 ; Huang et al., 2020 ), and an elevated neutrophil/lymphocyte ratio has been introduced as a biomarker of disease severity ( Kuri-Cervantes et al., 2020 ; Lagunas-Rangel, 2020 ; Liu et al., 2020 ; Qin et al., 2020 ). Although abundance and functional changes in the B and T cell compartment are not part of this review and are discussed in greater detail elsewhere ( Sette and Crotty, 2021 ), NK cells are affected as well ( Giamarellos-Bourboulis et al., 2020a ; Hadjadj et al., 2020 ; Kuri-Cervantes et al., 2020 ; Zheng et al., 2020a ). Reduced abundance of circulating NK cells was noticed in severe COVID-19 cases as well as an increased surface expression of NKG2A associated with a functionally exhausted phenotype, producing lower levels of cytokines on stimulation usually necessary to fight virus-infected cells ( Zheng et al., 2020a ). Increased levels of cytotoxicity ( PRF1 ), DNA replication ( DDIT4 ), and decreased inhibition of NF-κB signaling ( COMMD6 ) in NK cells was associated with recovery from COVID-19 ( Su et al., 2020 ). In early studies on patients during convalescence, an increased frequency of NK cells was reported ( Ni et al., 2020 ; Rodriguez et al., 2020 ).
Concerning the less frequent granulocyte subsets in circulation, elevated levels of eosinophils were found in severe COVID-19 ( Lucas et al., 2020 ) as well as an early transient increase in a CD62L + eosinophil subpopulation, reminiscent of lung eosinophils, albeit the potential role of these proinflammatory cells in the development of ARDS, and sudden clinical deterioration in severe patients still warrants further assessment ( Rodriguez et al., 2020 ). Basophils were found to be reduced in COVID-19 ( Laing et al., 2020 ), which has been noted to correlate with the humoral response ( Rodriguez et al., 2020 ).
Immune deviations in the adaptive immune system observed in severe COVID-19 might be directly linked to the immunosuppressive phenotypes observed in innate immune cells, such as HLA-DR lo monocytes impeding proper antigen presentation, or the expression of PD-L1 on suppressive neutrophil subpopulations possibly supporting lymphopenia by inducing apoptosis of lymphocytes ( Schulte-Schrepping et al., 2020 ). Further, the lack of costimulatory molecules on conventional DCs may contribute to functional impairment of T cell activation leading to delayed receptor binding domain- and nucleocapsid protein-specific T cell responses ( Zhou et al., 2020b ). Interestingly, cellular immune dysregulation of innate immune cells, particularly monocytes and DCs, seems to be sustained during convalescence irrespective of disease severity ( Files et al., 2021 ; Zhou et al., 2020b ). Further, because basophils have been implicated in potentiating the humoral immune response ( Denzel et al., 2008 ), this might constitute another avenue of innate-adaptive crosstalk in COVID-19.
All in all, a scenario emerges with rather differently programmed innate immune cells in mild versus severe disease courses ( Figure 4 ), which is also accompanied with similar differences in the adaptive immune response to SARS-CoV-2 ( Sette and Crotty, 2021 ). Following the initial local innate immune response, the occurrence of a systemic immune response component seems to be independent of disease severity, albeit cellular changes differ dramatically between mild and severe cases. Further, the decision, which kind of innate immune response to this virus will occur, seems to be made very early after infection. As a consequence, follow-up studies in larger cohorts determining molecular mechanisms explaining the different responses and clinical outcomes are urgently needed, because these will likely uncover novel druggable targets to prevent severe, critical, and fatal disease courses. However, we postulate that different molecular mechanisms might all converge toward a detrimental clinical path.

Factors that influence disease severity
Genetic factors impacting the immune response to sars-cov-2.
The clinical observation that otherwise healthy young individuals are among fatal cases suggested that host genetics might contribute to disease susceptibility and severity ( Ovsyannikova et al., 2020 ). Several international consortia (COVID-19 host genetics initiative [ COVID-19 Host Genetics Initiative, 2020 ] or the COVID Human Genetic Effort [ Casanova et al., 2020 ]) address genetic susceptibility either by genome-wide association studies (GWASs) or whole genome sequencing (WGS). It was postulated that signaling pathways identified to infer susceptibility for other viral infections might also be of importance for COVID-19 ( Ovsyannikova et al., 2020 ). An early GWAS conducted in Spain and Italy identified a gene cluster on chromosome 3 as a genetic susceptibility locus in patients with COVID-19 with respiratory failure ( Severe Covid-19 GWAS Group et al., 2020 ). These findings were confirmed by the COVID-19 Host Genetics Initiative ( COVID-19 Host Genetics Initiative, 2020 ) and the Genetics of Mortality in Critical Care (GenOMICC) GWAS ( Pairo-Castineira et al., 2020 ). The COVID-19 risk locus on chromosome 3 harbors immune genes including CCR9 , CXCR6 , XCR1 , CCR1 , and CCR2 . Comparative genomics strongly suggested that this COVID-19 risk locus is inherited from Neanderthals and is carried by around 50% of people in South Asia and around 16% of people in Europe ( Zeberg and Pääbo, 2020 ). CCR1 is a receptor for several chemokines (CCL3, CCL5, CCL7, and CCL23), some of which show altered expression with COVID-19 severity ( Chua et al., 2020 ). Furthermore, knockout of CCR1 in mice supports that this receptor protects from excessive inflammatory response ( Gerard et al., 1997 ) and decreases susceptibility to viruses and fungi ( Blease et al., 2000 ). From the GenOMICC study, it was proposed that critical illness in COVID-19 is associated with genetic variance in IFNAR2 and OAS2 , two genes involved in antiviral defense mechanisms, as well as DPP9 , TYK2 , and CCR2 , three genes previously associated with host-driven inflammatory lung injury, of which IFNAR2 , DPP9 , and CCR2 were also revealed by a transcriptome-wide association study ( Pairo-Castineira et al., 2020 ). It needs to be mentioned that these GWASs have not yet revealed any causative relationships, and the odds ratios were rather low, indicating that genetics might only play a minor influence at these gene loci.
The COVID Human Genetic Effort consortium sequenced genomes from patients with severe COVID-19 and compared them with mild patients. Emphasizing on genes within pathways that were associated with genetic susceptibility for other viral infections, particularly influenza, they identified 13, mainly loss-of-function mutations, associated with severe COVID-19 disease course in the TLR3, IRF7, and IRF9 pathways ( Zhang et al., 2020a ) ( Figure 5 ). pDCs from IRF7-deficient patients produced no type I IFN when infected with SARS-CoV-2, and fibroblasts from patients with deficiencies in TLR3, IRF7, and IFNAR1 were susceptible to SARS-CoV-2 infection suggesting that cell-autonomous innate immune mechanisms were not operational. The overall estimate for such deficiencies among patients with severe disease was 3.5%. These findings strongly point toward a causal relationship between genotype and phenotype and support an important role for type I IFNs in defense against SARS-CoV-2. The role of the type I IFN system was further underlined by the identification of the presence of a B cell autoimmune phenocopy of inborn type I IFN immunodeficiency in more than 10% of patients with severe COVID-19 ( Bastard et al., 2020 ). Autoantibodies against type I IFN were more prevalent in men, which might contribute to the excess of men among patients with severe COVID-19. The understanding of such immune-phenomena will allow better patient stratification in a precision medicine approach to tailor therapeutic options such as plasmapheresis, plasmablast depletion, or early treatment with recombinant type I IFNs for these patients.

Genetic factors for COVID-19 susceptibility support the central role of the innate immune system
The studies highlighted have already identified several loss-of-function mutations in molecules involved in the recognition of SARS-CoV-2 as well as important downstream signaling molecules important for a well-orchestrated and robust type I IFN response. Genes, for which loss-of-function mutations were identified, are depicted in bold pink with a pink circle. The autoimmune phenocopy of inborn type I IFN immunodeficiency is also depicted. For abbreviations, see Figure 2 legend.
Another approach identified variants of the X-chromosomal TLR7 as genetic susceptibility factors by performing genetic variance segregation analysis in two families with young family members (<35 years) admitted to the intensive care unit (ICU) due to severe COVID-19 ( van der Made et al., 2020 ) ( Figure 5 ). In both families, the TLR7 mutations resulted in downregulation of downstream genes including IRF7 , IFNB1 , and ISG15 on stimulation of PBMCs with the TLR7 ligand imiquimod. As a consequence, production of IFNγ was also decreased.
Collectively, these findings establish TLR3, TLR7, type I, and type II IFN responses as important immune mechanisms controlling infection with SARS-CoV-2. Ongoing studies are now addressing whether the assessment of these variants can be integrated in future risk scores used to identify individuals at elevated risk of developing severe COVID-19 or utilized in clinical tests to stratify patients for preventive and novel mechanism-based therapeutic measures.
Age, inflammaging, and COVID-19
Age is the major clinical risk factor for severe, critical, and fatal COVID-19 ( Richardson et al., 2020 ; Williamson et al., 2020 ). Although expression of SARS-CoV-2 entry factors, such as ACE2 and TMPRSS2 , in the airway have been found to increase with age ( Muus et al., 2021 ), there is no evidence so far that SARS-CoV-2 RNA levels differ between young, adult, and aged COVID-19 patients ( Jacot et al., 2020 ), indicating that it is the host immune response facilitating severe disease courses in the elderly. Changes observed within the innate immune system during aging might be related to an unfavorable outcome of COVID-19. In elderly, the number of dendritic cells (including pDCs) and homeostatic alveolar macrophages are reduced and so are the levels of pattern recognition receptors ( Shaw et al., 2013 ). This is accompanied with reduced type I IFN production combined with perturbed inflammatory cytokine responses to viral infections ( Canaday et al., 2010 ; Molony et al., 2017 ). Because autoantibodies to type I IFN are found preferentially in the elderly population, this provides a causal explanation for age as the major clinical risk factor in ∼10% of cases of critical COVID-19 pneumonia ( Bastard et al., 2020 ).
The effect of the age-associated changes seen in both the innate and the adaptive arm of the immune system have been investigated in several animal models in the context of coronavirus infection ( Channappanavar and Perlman, 2020 ; Pence, 2020 ). In aged macaques ( Yu et al., 2020 ) and hamsters ( Imai et al., 2020 ), SARS-CoV-2 infection is associated with more severe respiratory illness and fatal pneumonia compared to young animals. From experiments with SARS-CoV, it has been postulated that particularly altered innate immune responses in aged animals contribute to severe disease courses ( Smits et al., 2010 ; Zhao et al., 2011 ).
Systemically, the aged innate immune system shares another hallmark of severe COVID-19, namely increased myelopoiesis with elevated numbers of myeloid cells including neutrophils and monocytes in circulation and inflammatory macrophages in the tissues ( Ho et al., 2019 ). For all major myeloid cells, a decline or deviation of cellular functions including migration to infectious tissues, phagocytosis, production of prostaglandins, or nitric and superoxide is well established with age ( Shaw et al., 2013 ). For a particularly strong manifestation of all these changes, the term inflammaging was coined ( Boren and Gershwin, 2004 ; Franceschi et al., 2017 ). Inflammaging is considered a chronic, sterile inflammation characterized by elevated levels of inflammatory mediators in the circulation including IL-6, TNF, CXCL8, CCL2, CRP, and PLA2GD (group IID secretory phospholipase A2), which in turn have been directly linked to reduced numbers and function of myeloid DCs and pDCs ( Frasca and Blomberg, 2016 ). Numerous factors inducing this inflammatory state have been suggested including DNA damage, increased epigenetic variations, release of mitochondrial DNA, age-related leakage of intestinal microbiota, persistent viral infections (e.g., with cytomegalovirus), or cellular immunosenescence ( Cheung et al., 2018 ; Cunha et al., 2020 ; Franceschi et al., 2017 ).
Using inflammaging-derived signatures, it was shown that transcriptional changes observed in immune cells from COVID-19 patients are reminiscent of the aging phenotype ( Schulte-Schrepping et al., 2020 ; Zheng et al., 2020b ). Because inflammaging is a significant risk factor for age-related inflammatory conditions including cardiovascular disease, diabetes, cancer, autoimmune conditions, or Alzheimer’s disease ( Boren and Gershwin, 2004 ; Frasca et al., 2017 ), it is likely to be one of the most important molecular risk factors for COVID-19. In this setting, with defective functionality in the myeloid compartment along an increase in proinflammatory monocytes and higher baseline levels of many proinflammatory mediators such as IL-6 ( Shaw et al., 2013 ), it can be hypothesized that the initiated innate immune response during COVID-19 exacerbates the already existing disbalance of the immune system even further toward a proinflammatory and dysfunctional state. A recent study illustrated that age has an impact on the inflammatory mediators present during COVID-19 ( Angioni et al., 2020 ). Here, CXCL8, IL-10, IL-15, IL-27, and TNF positively correlated with age, longer hospitalization, and more severe disease courses. Based on the identification of large numbers of dysfunctional immune cells, we postulate that the higher severity in aged individuals is caused by dysregulated, rather than impaired, host innate immunity.
Adaptation of innate immune cells: A role for trained immunity?
A specialized form of innate immune adaptation is the induction of immune memory (also termed trained immunity) ( Netea et al., 2020a ), best characterized for BCG vaccination ( Giamarellos-Bourboulis et al., 2020b ; Moorlag et al., 2020 ) and β-glucan stimulation of monocytes ( Dos Santos et al., 2019 ; Kleinnijenhuis et al., 2012 ). In principle, exposure of innate immune cells and their precursors to either BCG or β-glucan leads to their reprogramming, inducing heterologous protection against other pathogens including viruses ( Arts et al., 2018 ).
In context of COVID-19, it has been postulated that trained immunity might be a means to adapt the homeostatic state of the innate immune system toward a more favorable outcome in case of SARS-CoV-2 infection, particularly in those groups at higher risk for severe disease courses ( Netea et al., 2020b ; O’Neill and Netea, 2020 ). Early retrospective studies on the impact of BCG vaccination in childhood resulted in contradictory results concerning a beneficial role for fighting SARS-CoV-2 infection ( de Chaisemartin and de Chaisemartin, 2020 ; Escobar et al., 2020 ; Hamiel et al., 2020 ; Kinoshita and Tanaka, 2020 ; Lindestam Arlehamn et al., 2020 ). However, more recent epidemiological observations from the first wave of the pandemic indicate that the prevalence of individuals with positive tuberculin sensitivity tests—either due to exposure to Mycobacterium spp. or BCG vaccination—inversely correlates with the incidence of COVID-19 ( Singh et al., 2020 ). Although not providing any causal relationship for trained immunity and protection from COVID-19, such correlations triggered randomized clinical trials addressing whether trained immunity adapts the innate immune system toward a more favorable response to SARS-CoV-2 infections ( Netea et al., 2020b ; O’Neill and Netea, 2020 ). In late 2020, 21 newly initiated trials assessing protective effects of BCG vaccination against COVID-19 and 3 trials assessing VPM1002, a further development of the old BCG vaccine, were registered at https://ClinicalTrials.gov (accessed 2021/01/19) ( Nowill and de Campos-Lima, 2020 ). First results are expected in the middle of 2021 (M. Netea, personal communication). In this context, it will also be important to see whether boosting the potential of proinflammatory cytokine production (IL-1, IL-6, and TNF) by the induction of trained immunity ( Kleinnijenhuis et al., 2012 ) will exaggerate the observed hyperinflammatory cytokine responses in severe COVID-19, or whether trained immunity would reprogram innate immune cells, so that these cells can react more robustly during the early hours after infection thereby disrupting the path toward the vicious cycle of viral sepsis ( Netea et al., 2020b ; O’Neill and Netea, 2020 ). Further studies identifying the molecular mechanisms that could prevent reprogramming of innate immune cells toward a suppressive phenotype in severe COVID-19 are urgently needed.
Immune parameters as biomarkers
High-throughput, high-resolution, and high-content technologies assessing cellular or soluble mediators of the immune response are ideal to discover new potential biomarkers addressing important clinical questions concerning diagnostics, monitoring, outcome prediction, or therapeutic options for COVID-19. From a clinical perspective, actionable measurements of soluble factors are focused on technologies assessing serum or plasma samples, yet different combinations of soluble factors were assessed in different studies in context of COVID-19 with only a few choosing comprehensive approaches ( Messner et al., 2020 ; Su et al., 2020 ).
Multi-omics analysis of plasma proteins including cytokines and chemokines distinguished mild and moderate COVID-19 ( Su et al., 2020 ) suggesting that immune parameters might be used for disease classification or outcome prediction. In a study by the Mount Sinai Health System in New York, four cytokines (IL-6, CXCL8, TNF, and IL-1β) were assessed in serum from 1,484 COVID-19 patients ( Del Valle et al., 2020 ) of which TNF, IL-1β, and IL-6 were elevated in COVID-19 patients, and TNF and IL-6 turned out to be independent predictors of disease severity and fatal outcome. In another study, a machine learning-based classifier of eleven plasma proteins, including orosomucoid-1/alpha-1-acid glycoprotein-1 (ORM1), orosomucoid-1/alpha-1-acid glycoprotein-2 (ORM2), fetuin-B (FETUB), and cholesteryl ester transfer protein (CETP), was generated to determine and predict disease outcome ( Shu et al., 2020 ). Several other classifiers, including immune mediators, ( Lucas et al., 2020 ) were developed for predicting fatality or convalescence, further indicating that immune parameters from the circulation could be utilized in the clinics. The currently ongoing and future studies need to address which of the many suggested biomarkers are best suited for diagnosis, disease outcome prediction, or therapy response. This is critical when considering the development of stratified therapies ( Buszko et al., 2020 ).
Therapeutic options targeting immune deviation
Three major concepts emerge concerning therapeutic and preventive options in COVID-19: (1) targeting the virus and its cellular life cycle by antiviral drugs, (2) developing vaccines that can either prevent or mitigate disease symptoms after infection, and (3) targeting the deviation of the immune response to avoid or mitigate severe and fatal disease outcome ( Riva et al., 2020 ; Vabret et al., 2020 ). Targeting the innate immune system might play a role in all three areas.
The antivirals are divided in direct and indirect acting antivirals, targeting molecules and mechanisms of the virus itself or host cell proteins, respectively. Because SARS-CoV-2 antagonizes the type I IFN system, drugs strengthening this cellular defense system might improve early innate immune responses. Type I IFN therapy in patients with genetic deficits in the IFN system ( Zhang et al., 2020a ), but not in those with autoimmune phenocopies of these deficits ( Bastard et al., 2020 ), might be beneficial if provided sufficiently early ( Hadjadj et al., 2020 ; Wang et al., 2020b ). More than 20 clinical trials are currently evaluating the efficacy of type I IFN treatment ( https://www.clinicaltrials.gov , accessed 2021/01/19) ( Wang et al., 2020b ; Zhou et al., 2020a ), the best time window, and benefits versus risks of IFN therapy. Alternatives such as IFNλ (type III IFN) only targeting receptors on epithelial cells without the broader effects of type I IFNs ( Prokunina-Olsson et al., 2020 ) are also under clinical evaluation. Other indirect antivirals blocking viral cell entry (e.g., by targeting proteases such as TMPRSS2) have been suggested as potential therapies ( Kaur et al., 2021 ), yet definitive proof for clinical efficacy is still lacking. Antiviral protein interaction mapping revealed further promising sets of antivirals: those that affect translation and those that modulate the sigma-1 and sigma-2 receptors, the cellular interaction partners of SARS-CoV-2 NSP6 and ORF9c ( Gordon et al., 2020 ).
Based on results from phase-III vaccine trials utilizing mRNA vaccines ( Anderson et al., 2020 ; Polack et al., 2020 ), two vaccines have been approved in the United States, United Kingdom, Israel, and the European Union, and several million individuals have been vaccinated since late 2020. A most surprising finding in light of age-related changes in the adaptive and the innate immune system is the similarly high efficiency of these vaccines in the elderly population. This unexpected success requires further mechanistic and molecular evaluations about the elicited immune response because this might give insights how age-related alterations of the immune system are overcome by this type of vaccination.
The third strategy is targeting the deviation of the immune response to avoid or mitigate severe and fatal disease outcomes. A starting point for many therapeutic strategies has been the hyperinflammatory state in severe disease ( Tang et al., 2020 ; Wang et al., 2020a ). Not surprisingly, trials targeting cytokines such as IL-6, IL-1, IFNγ, IL-1R, TNF, CXCL8, GM-CSF, GM-CSF receptor, or IL-37 have been reported, as well as strategies attempting to achieve disruption of chemokine signaling (e.g., via CCR1, CCR2, and CCR5) to prevent overt innate immune cell recruitment into the lung ( Wang et al., 2020a ). Because COVID-19 presents with such heterogeneity, a certain drug might be beneficial in one setting, while having no effect in another. For example, inconsistent results have been reported from large clinical trials when trying to inhibit IL-6 associated with hyperinflammation ( Huang and Jordan, 2020 ). A randomized clinical trial assessing tocilizumab, an anti-IL-6 antibody ( Stone et al., 2020 ), showed no benefit for moderately ill patients concerning effectiveness of preventing intubation or death. In contrast, among critically ill patients, the risk of in-hospital mortality was lower in patients treated with tocilizumab in the first 2 days of ICU admission ( Gupta et al., 2021 ), which has also been reported in earlier trials ( Huang and Jordan, 2020 ). It cannot yet be ruled out that targeting IL-6 might be beneficial for a group of patients in specific clinical settings. This might be similarly true for targeting IL-1 with anakinra for which several smaller studies were reporting beneficial effects ( Iglesias-Julián et al., 2020 ; Kooistra et al., 2020 ), yet results from larger randomized trials are still missing.
Whether targeting single effector molecules will be efficient in disrupting the COVID-19-associated immune deviation awaits the report of ongoing clinical trials. Targeting several of these pathways simultaneously (e.g., by inhibiting Janus kinases) might overcome such limitations ( McCreary and Pogue, 2020 ; Wu and Yang, 2020 ). In early clinical trials, the use of the JAK inhibitor baricitinib showed a reduction in serum levels of IL-6, IL-1β, and TNF ( Bronte et al., 2020 ), suppressed the production of proinflammatory cytokines in lung macrophages, and the recruitment of neutrophils to the lung ( Hoang et al., 2021 ). Randomized clinical trials have to be initiated to validate these promising results. Along these lines, therapies targeting the kallikrein-kinin system with icatibant ( van de Veerdonk et al., 2020 ), or the coagulation system with antibodies against C5a and C3a ( Mastellos et al., 2020 ), have been introduced as additional options to ameliorate clinical symptoms and reduce mortality rates. Further, these therapeutic strategies might be considered in combination with immunotherapies such as anti-IL-6 or anti-IL-1 in severe COVID-19.
Reverse transcriptomics of blood immune cells derived from COVID-19 patients predicted the broadly anti-inflammatory drug dexamethasone and other corticosteroids as potentially beneficial for a subgroup of severely ill COVID-19 patients ( Aschenbrenner et al., 2021 ). Indeed, dexamethasone was shown to be beneficial, particularly in patients with severe disease courses where it reduced 28-day mortality in randomized clinical trials ( RECOVERY Collaborative Group et al., 2021 ; Tomazini et al., 2020 ; WHO Rapid Evidence Appraisal for COVID-19 Therapies (REACT) Working Group et al., 2020 ). Further, molecular prediction of drug responses may guide the way for precision medicine approaches following patient stratification.
Summary and outlook
Valuable descriptive and correlative information about COVID-19 has been gathered during the first months of the pandemic ( Figure 6 ). COVID-19 can be divided in at least five phases ( Figure 1 ), starting with the infection of ACE2 + epithelial cells of the respiratory tract leading to cell-autonomous defense mechanisms of the infected cells. The second, local immune response phase is characterized by an atypical and heterogeneous type I IFN response due to SARS-CoV-2 attacking the IFN system. Further mechanistic insights into the regulation of the heterogeneity of the type I IFN response combined with the elevated expression of NF-κB-associated inflammatory genes mainly in severe COVID-19 are urgently needed. Organ involvement, magnitude of clinical symptoms, and length of this phase is highly variable, and whether the heterogeneity of innate immunity is causally related to the clinical heterogeneity also requires further work. Here, sex differences concerning innate immunity in COVID-19 need to be considered ( Takahashi et al., 2020 ) and molecularly characterized. This is similarly true for a potential role of innate immune cells such as NK cells, innate lymphoid cells, mast cells, basophils, or eosinophils, which have not yet been studied in sufficient detail in COVID-19.

Summary of current knowledge, outlook, and open questions
Established disease courses describing cell and organ involvement during different phases of COVID-19 including the possibility of not only returning to homeostasis (convalescence) but also the potential chronification of the disease (“Long COVID-19”). Possible COVID-19 disease courses are depicted as differently colored curves of disease severity over time. Major open questions and future tasks concerning the innate immune response to SARS-CoV-2 are outlined along the five phases at the bottom of the figure.
To further improve our knowledge on COVID-19 and, in particular, on innate immunity against SARS-CoV-2 infection, future studies need to be tailored to address important questions for each disease phase. To better determine individuals at risk for an incomplete innate immune response during infection and local immunity, risk scores based on genetic findings ( Zhang et al., 2020a ) but also integrating environmental conditions (e.g., microbiome, mucosal infections, inflammaging, immunosenescence, and comorbidities) need to be developed that would predict insufficient first line defense against SARS-CoV-2. Parameters measurable at earlier phases of the disease would be useful to predict the outcome of viral sepsis. Recent attempts have explored this in smaller studies ( Bernardes et al., 2020 ; Del Valle et al., 2020 ; Shu et al., 2020 ; Silvin et al., 2020 ), however, joining forces to address some of the suggested early predictors and validate them in larger multicenter trials ( Arunachalam et al., 2020 ; Su et al., 2020 ) utilizing high-content, high-throughput, high-resolution technologies is the next step to study COVID-19 systematically. A better understanding of the molecular immune mechanisms, particularly at the transition from a local to a systemic response phase and during viral sepsis, is a prerequisite to introduce novel therapies. For example, the vicious cycle of viral sepsis developing in an affected individual already suffering from chronic obstructive pulmonary disease (COPD) might differ from the disease course observed in an obese person and therefore might require other therapies. Large enough observational validation studies with longitudinal sampling will ensure sufficiently covered molecular subtypes of COVID-19, which subsequently need to be supported by mechanistic studies in animal models ( Mulchandani et al., 2021 ).
More evidence accumulates suggesting that a yet unknown fraction of patients will suffer from what is described as Long COVID-19, a chronic illness with very heterogeneous symptoms ( Marshall, 2020 ). These include chronic fatigue syndrome ( Salisbury, 2020 ) and a spectrum of psychiatric disorders ranging from cognitive decline, depression, to even neurodegeneration ( Taquet et al., 2021 ). A better description of the heterogeneity of Long COVID-19 and the underlying molecular mechanisms need to be established to provide therapeutic options for these patients. It will be interesting to see how the immune deviations seen in acute COVID-19 extend or further develop in these patients. It will be important to generate large enough registries of individuals suffering from Long COVID-19 to address the mechanisms involved in this heterogeneous syndrome.
Acknowledgments
We want to apologize to all those colleagues whose excellent work we could not reference here. A.C.A. was supported by an intramural grant from the Department of Genomics & Immunoregulation at the LIMES Institute and by an ImmunoSensation2 Women in Science grant. The work of J.L.S. was supported by the German Research Foundation (DFG) (INST 37/1049-1, INST 216/981-1, INST 257/605-1, INST 269/768-1, INST 217/988-1, INST 217/577-1, SCHU 950/8-1, GRK 2168, and TP11) and under Germany’s Excellence Strategy (DFG) (EXC2151; 390873048), the EU project SYSCID (733100), and the BMBF-funded grant iTREAT (01ZX1902B).
Declaration of interests
The authors declare no competing interests.
- Acharya D., Liu G., Gack M.U. Dysregulation of type I interferon responses in COVID-19. Nat. Rev. Immunol. 2020; 20 :397–398. [ PMC free article ] [ PubMed ] [ Google Scholar ]
- Ackermann M., Verleden S.E., Kuehnel M., Haverich A., Welte T., Laenger F., Vanstapel A., Werlein C., Stark H., Tzankov A., et al. Pulmonary Vascular Endothelialitis, Thrombosis, and Angiogenesis in Covid-19. N. Engl. J. Med. 2020; 383 :120–128. [ PMC free article ] [ PubMed ] [ Google Scholar ]
- Amor S., Fernández Blanco L., Baker D. Innate immunity during SARS-CoV-2: evasion strategies and activation trigger hypoxia and vascular damage. Clin. Exp. Immunol. 2020; 202 :193–209. [ PMC free article ] [ PubMed ] [ Google Scholar ]
- Anderson E.J., Rouphael N.G., Widge A.T., Jackson L.A., Roberts P.C., Makhene M., Chappell J.D., Denison M.R., Stevens L.J., Pruijssers A.J., et al.mRNA-1273 Study Group Safety and Immunogenicity of SARS-CoV-2 mRNA-1273 Vaccine in Older Adults. N. Engl. J. Med. 2020; 383 :2427–2438. [ PMC free article ] [ PubMed ] [ Google Scholar ]
- Angioni R., Sánchez-Rodríguez R., Munari F., Bertoldi N., Arcidiacono D., Cavinato S., Marturano D., Zaramella A., Realdon S., Cattelan A., et al. Age-severity matched cytokine profiling reveals specific signatures in Covid-19 patients. Cell Death Dis. 2020; 11 :957. [ PMC free article ] [ PubMed ] [ Google Scholar ]
- Arts R.J.W., Moorlag S.J.C.F.M., Novakovic B., Li Y., Wang S.-Y., Oosting M., Kumar V., Xavier R.J., Wijmenga C., Joosten L.A.B., et al. BCG Vaccination Protects against Experimental Viral Infection in Humans through the Induction of Cytokines Associated with Trained Immunity. Cell Host Microbe. 2018; 23 :89–100.e5. [ PubMed ] [ Google Scholar ]
- Arunachalam P.S., Wimmers F., Mok C.K.P., Perera R.A.P.M., Scott M., Hagan T., Sigal N., Feng Y., Bristow L., Tak-Yin Tsang O., et al. Systems biological assessment of immunity to mild versus severe COVID-19 infection in humans. Science. 2020; 369 :1210–1220. [ PMC free article ] [ PubMed ] [ Google Scholar ]
- Aschenbrenner A.C., Mouktaroudi M., Krämer B., Oestreich M., Antonakos N., Nuesch-Germano M., Gkizeli K., Bonaguro L., Reusch N., Baßler K., et al.German COVID-19 Omics Initiative (DeCOI) Disease severity-specific neutrophil signatures in blood transcriptomes stratify COVID-19 patients. Genome Med. 2021; 13 :7. [ PMC free article ] [ PubMed ] [ Google Scholar ]
- Ashary N., Bhide A., Chakraborty P., Colaco S., Mishra A., Chhabria K., Jolly M.K., Modi D. Single-Cell RNA-seq Identifies Cell Subsets in Human Placenta That Highly Expresses Factors Driving Pathogenesis of SARS-CoV-2. Front. Cell Dev. Biol. 2020; 8 :783. [ PMC free article ] [ PubMed ] [ Google Scholar ]
- Banerjee A.K., Blanco M.R., Bruce E.A., Honson D.D., Chen L.M., Chow A., Bhat P., Ollikainen N., Quinodoz S.A., Loney C., et al. SARS-CoV-2 Disrupts Splicing, Translation, and Protein Trafficking to Suppress Host Defenses. Cell. 2020; 183 :1325–1339.e21. [ PMC free article ] [ PubMed ] [ Google Scholar ]
- Bastard P., Rosen L.B., Zhang Q., Michailidis E., Hoffmann H.H., Zhang Y., Dorgham K., Philippot Q., Rosain J., Béziat V., et al.HGID Lab. NIAID-USUHS Immune Response to COVID Group. COVID Clinicians. COVID-STORM Clinicians. Imagine COVID Group. French COVID Cohort Study Group. Milieu Intérieur Consortium. CoV-Contact Cohort. Amsterdam UMC Covid-19 Biobank. COVID Human Genetic Effort Autoantibodies against type I IFNs in patients with life-threatening COVID-19. Science. 2020; 370 :eabd4585. [ PMC free article ] [ PubMed ] [ Google Scholar ]
- Berlin D.A., Gulick R.M., Martinez F.J. Severe Covid-19. N. Engl. J. Med. 2020; 383 :2451–2460. [ PubMed ] [ Google Scholar ]
- Bernardes J.P., Mishra N., Tran F., Bahmer T., Best L., Blase J.I., Bordoni D., Franzenburg J., Geisen U., Josephs-Spaulding J., et al. Longitudinal Multi-omics Analyses Identify Responses of Megakaryocytes, Erythroid Cells, and Plasmablasts as Hallmarks of Severe COVID-19. Immunity. 2020; 53 :1296–1314.e9. [ PMC free article ] [ PubMed ] [ Google Scholar ]
- Blanco-Melo D., Nilsson-Payant B.E., Liu W.-C., Uhl S., Hoagland D., Møller R., Jordan T.X., Oishi K., Panis M., Sachs D., et al. Imbalanced Host Response to SARS-CoV-2 Drives Development of COVID-19. Cell. 2020; 181 :1036–1045.e9. [ PMC free article ] [ PubMed ] [ Google Scholar ]
- Blease K., Mehrad B., Standiford T.J., Lukacs N.W., Kunkel S.L., Chensue S.W., Lu B., Gerard C.J., Hogaboam C.M. Airway remodeling is absent in CCR1-/- mice during chronic fungal allergic airway disease. J. Immunol. 2000; 165 :1564–1572. [ PubMed ] [ Google Scholar ]
- Boren E., Gershwin M.E. Inflamm-aging: autoimmunity, and the immune-risk phenotype. Autoimmun. Rev. 2004; 3 :401–406. [ PubMed ] [ Google Scholar ]
- Bost P., Giladi A., Liu Y., Bendjelal Y., Xu G., David E., Blecher-Gonen R., Cohen M., Medaglia C., Li H., et al. Host-Viral Infection Maps Reveal Signatures of Severe COVID-19 Patients. Cell. 2020; 181 :1475–1488.e12. [ PMC free article ] [ PubMed ] [ Google Scholar ]
- Braun J., Loyal L., Frentsch M., Wendisch D., Georg P., Kurth F., Hippenstiel S., Dingeldey M., Kruse B., Fauchere F., et al. SARS-CoV-2-reactive T cells in healthy donors and patients with COVID-19. Nature. 2020; 587 :270–274. [ PubMed ] [ Google Scholar ]
- Bronte V., Ugel S., Tinazzi E., Vella A., De Sanctis F., Canè S., Batani V., Trovato R., Fiore A., Petrova V., et al. Baricitinib restrains the immune dysregulation in patients with severe COVID-19. J. Clin. Invest. 2020; 130 :6409–6416. [ PMC free article ] [ PubMed ] [ Google Scholar ]
- Buszko M., Park J.-H., Verthelyi D., Sen R., Young H.A., Rosenberg A.S. The dynamic changes in cytokine responses in COVID-19: a snapshot of the current state of knowledge. Nat. Immunol. 2020; 21 :1146–1151. [ PubMed ] [ Google Scholar ]
- Callaway E. The coronavirus is mutating - does it matter? Nature. 2020; 585 :174–177. [ PubMed ] [ Google Scholar ]
- Canaday D.H., Amponsah N.A., Jones L., Tisch D.J., Hornick T.R., Ramachandra L. Influenza-induced production of interferon-alpha is defective in geriatric individuals. J. Clin. Immunol. 2010; 30 :373–383. [ PMC free article ] [ PubMed ] [ Google Scholar ]
- Candido D.S., Claro I.M., de Jesus J.G., Souza W.M., Moreira F.R.R., Dellicour S., Mellan T.A., du Plessis L., Pereira R.H.M., Sales F.C.S., et al.Brazil-UK Centre for Arbovirus Discovery, Diagnosis, Genomics and Epidemiology (CADDE) Genomic Network Evolution and epidemic spread of SARS-CoV-2 in Brazil. Science. 2020; 369 :1255–1260. [ PMC free article ] [ PubMed ] [ Google Scholar ]
- Cantuti-Castelvetri L., Ojha R., Pedro L.D., Djannatian M., Franz J., Kuivanen S., van der Meer F., Kallio K., Kaya T., Anastasina M., et al. Neuropilin-1 facilitates SARS-CoV-2 cell entry and infectivity. Science. 2020; 370 :856–860. [ PMC free article ] [ PubMed ] [ Google Scholar ]
- Cao X. COVID-19: immunopathology and its implications for therapy. Nat. Rev. Immunol. 2020; 20 :269–270. [ PMC free article ] [ PubMed ] [ Google Scholar ]
- Carter M.J., Fish M., Jennings A., Doores K.J., Wellman P., Seow J., Acors S., Graham C., Timms E., Kenny J., et al. Peripheral immunophenotypes in children with multisystem inflammatory syndrome associated with SARS-CoV-2 infection. Nat. Med. 2020; 26 :1701–1707. [ PubMed ] [ Google Scholar ]
- Casanova J.-L., Su H.C., COVID Human Genetic Effort A Global Effort to Define the Human Genetics of Protective Immunity to SARS-CoV-2 Infection. Cell. 2020; 181 :1194–1199. [ PMC free article ] [ PubMed ] [ Google Scholar ]
- de Chaisemartin C., de Chaisemartin L. BCG vaccination in infancy does not protect against COVID-19. Evidence from a natural experiment in Sweden. Clin. Infect. Dis. 2020 doi: 10.1093/cid/ciaa1223. Published online August 23, 2020. [ PMC free article ] [ PubMed ] [ CrossRef ] [ Google Scholar ]
- Channappanavar R., Perlman S. Age-related susceptibility to coronavirus infections: role of impaired and dysregulated host immunity. J. Clin. Invest. 2020; 130 :6204–6213. [ PMC free article ] [ PubMed ] [ Google Scholar ]
- Cheung P., Vallania F., Warsinske H.C., Donato M., Schaffert S., Chang S.E., Dvorak M., Dekker C.L., Davis M.M., Utz P.J., et al. Single-Cell Chromatin Modification Profiling Reveals Increased Epigenetic Variations with Aging. Cell. 2018; 173 :1385–1397.e14. [ PMC free article ] [ PubMed ] [ Google Scholar ]
- Chua R.L., Lukassen S., Trump S., Hennig B.P., Wendisch D., Pott F., Debnath O., Thürmann L., Kurth F., Völker M.T., et al. COVID-19 severity correlates with airway epithelium-immune cell interactions identified by single-cell analysis. Nat. Biotechnol. 2020; 38 :970–979. [ PubMed ] [ Google Scholar ]
- Consiglio C.R., Cotugno N., Sardh F., Pou C., Amodio D., Rodriguez L., Tan Z., Zicari S., Ruggiero A., Pascucci G.R., et al.CACTUS Study Team The Immunology of Multisystem Inflammatory Syndrome in Children with COVID-19. Cell. 2020; 183 :968–981.e7. [ PMC free article ] [ PubMed ] [ Google Scholar ]
- Coronaviridae Study Group of the International Committee on Taxonomy of Viruses The species Severe acute respiratory syndrome-related coronavirus: classifying 2019-nCoV and naming it SARS-CoV-2. Nat. Microbiol. 2020; 5 :536–544. [ PMC free article ] [ PubMed ] [ Google Scholar ]
- COVID-19 Host Genetics Initiative The COVID-19 Host Genetics Initiative, a global initiative to elucidate the role of host genetic factors in susceptibility and severity of the SARS-CoV-2 virus pandemic. Eur. J. Hum. Genet. 2020; 28 :715–718. [ PMC free article ] [ PubMed ] [ Google Scholar ]
- Cunha L.L., Perazzio S.F., Azzi J., Cravedi P., Riella L.V. Remodeling of the Immune Response With Aging: Immunosenescence and Its Potential Impact on COVID-19 Immune Response. Front. Immunol. 2020; 11 :1748. [ PMC free article ] [ PubMed ] [ Google Scholar ]
- Cyranoski D. Profile of a killer: the complex biology powering the coronavirus pandemic. Nature. 2020; 581 :22–26. [ PubMed ] [ Google Scholar ]
- Del Valle D.M., Kim-Schulze S., Huang H.-H., Beckmann N.D., Nirenberg S., Wang B., Lavin Y., Swartz T.H., Madduri D., Stock A., et al. An inflammatory cytokine signature predicts COVID-19 severity and survival. Nat. Med. 2020; 26 :1636–1643. [ PMC free article ] [ PubMed ] [ Google Scholar ]
- Denzel A., Maus U.A., Rodriguez Gomez M., Moll C., Niedermeier M., Winter C., Maus R., Hollingshead S., Briles D.E., Kunz-Schughart L.A., et al. Basophils enhance immunological memory responses. Nat. Immunol. 2008; 9 :733–742. [ PubMed ] [ Google Scholar ]
- Dos Santos J.C., Barroso de Figueiredo A.M., Teodoro Silva M.V., Cirovic B., de Bree L.C.J., Damen M.S.M.A., Moorlag S.J.C.F.M., Gomes R.S., Helsen M.M., Oosting M., et al. β-Glucan-Induced Trained Immunity Protects against Leishmania braziliensis Infection: a Crucial Role for IL-32. Cell Rep. 2019; 28 :2659–2672.e6. [ PubMed ] [ Google Scholar ]
- Escobar L.E., Molina-Cruz A., Barillas-Mury C. BCG vaccine protection from severe coronavirus disease 2019 (COVID-19) Proc. Natl. Acad. Sci. USA. 2020; 117 :17720–17726. [ PMC free article ] [ PubMed ] [ Google Scholar ]
- Fajgenbaum D.C., June C.H. Cytokine Storm. N. Engl. J. Med. 2020; 383 :2255–2273. [ PMC free article ] [ PubMed ] [ Google Scholar ]
- de Figueiredo C.S., Raony Í., Giestal-de-Araujo E. SARS-CoV-2 Targeting the Retina: Host-virus Interaction and Possible Mechanisms of Viral Tropism. Ocul. Immunol. Inflamm. 2020; 28 :1301–1304. [ PubMed ] [ Google Scholar ]
- Files J.K., Boppana S., Perez M.D., Sarkar S., Lowman K.E., Qin K., Sterrett S., Carlin E., Bansal A., Sabbaj S., et al. Sustained cellular immune dysregulation in individuals recovering from SARS-CoV-2 infection. J. Clin. Invest. 2021; 131 :140491. [ PMC free article ] [ PubMed ] [ Google Scholar ]
- Franceschi C., Salvioli S., Garagnani P., de Eguileor M., Monti D., Capri M. Immunobiography and the heterogeneity of immune responses in the elderly: A focus on inflammaging and trained immunity. Front. Immunol. 2017; 8 :982. [ PMC free article ] [ PubMed ] [ Google Scholar ]
- Frasca D., Blomberg B.B. Inflammaging decreases adaptive and innate immune responses in mice and humans. Biogerontology. 2016; 17 :7–19. [ PMC free article ] [ PubMed ] [ Google Scholar ]
- Frasca D., Blomberg B.B., Paganelli R. Aging, Obesity, and Inflammatory Age-Related Diseases. Front. Immunol. 2017; 8 :1745. [ PMC free article ] [ PubMed ] [ Google Scholar ]
- Gandhi R.T., Lynch J.B., Del Rio C. Mild or Moderate Covid-19. N. Engl. J. Med. 2020; 383 :1757–1766. [ PubMed ] [ Google Scholar ]
- Gerard C., Frossard J.L., Bhatia M., Saluja A., Gerard N.P., Lu B., Steer M. Targeted disruption of the beta-chemokine receptor CCR1 protects against pancreatitis-associated lung injury. J. Clin. Invest. 1997; 100 :2022–2027. [ PMC free article ] [ PubMed ] [ Google Scholar ]
- Giamarellos-Bourboulis E.J., Netea M.G., Rovina N., Akinosoglou K., Antoniadou A., Antonakos N., Damoraki G., Gkavogianni T., Adami M.-E., Katsaounou P., et al. Complex Immune Dysregulation in COVID-19 Patients with Severe Respiratory Failure. Cell Host Microbe. 2020; 27 :992–1000.e3. [ PMC free article ] [ PubMed ] [ Google Scholar ]
- Giamarellos-Bourboulis E.J., Tsilika M., Moorlag S., Antonakos N., Kotsaki A., Domínguez-Andrés J., Kyriazopoulou E., Gkavogianni T., Adami M.E., Damoraki G., et al. Activate: Randomized Clinical Trial of BCG Vaccination against Infection in the Elderly. Cell. 2020; 183 :315–323.e9. [ PMC free article ] [ PubMed ] [ Google Scholar ]
- Gordon D.E., Jang G.M., Bouhaddou M., Xu J., Obernier K., White K.M., O’Meara M.J., Rezelj V.V., Guo J.Z., Swaney D.L., et al. A SARS-CoV-2 protein interaction map reveals targets for drug repurposing. Nature. 2020; 583 :459–468. [ PMC free article ] [ PubMed ] [ Google Scholar ]
- Grifoni A., Weiskopf D., Ramirez S.I., Mateus J., Dan J.M., Moderbacher C.R., Rawlings S.A., Sutherland A., Premkumar L., Jadi R.S., et al. Targets of T Cell Responses to SARS-CoV-2 Coronavirus in Humans with COVID-19 Disease and Unexposed Individuals. Cell. 2020; 181 :1489–1501.e15. [ PMC free article ] [ PubMed ] [ Google Scholar ]
- Gruber C.N., Patel R.S., Trachtman R., Lepow L., Amanat F., Krammer F., Wilson K.M., Onel K., Geanon D., Tuballes K., et al. Mapping Systemic Inflammation and Antibody Responses in Multisystem Inflammatory Syndrome in Children (MIS-C) Cell. 2020; 183 :982–995.e14. [ PMC free article ] [ PubMed ] [ Google Scholar ]
- Guan W.-J., Ni Z.-Y., Hu Y., Liang W.-H., Ou C.-Q., He J.-X., Liu L., Shan H., Lei C.-L., Hui D.S.C., et al.China Medical Treatment Expert Group for Covid-19 Clinical characteristics of coronavirus disease 2019 in China. N. Engl. J. Med. 2020; 382 :1708–1720. [ PMC free article ] [ PubMed ] [ Google Scholar ]
- Gupta A., Madhavan M.V., Sehgal K., Nair N., Mahajan S., Sehrawat T.S., Bikdeli B., Ahluwalia N., Ausiello J.C., Wan E.Y., et al. Extrapulmonary manifestations of COVID-19. Nat. Med. 2020; 26 :1017–1032. [ PubMed ] [ Google Scholar ]
- Gupta S., Wang W., Hayek S.S., Chan L., Mathews K.S., Melamed M.L., Brenner S.K., Leonberg-Yoo A., Schenck E.J., Radbel J., et al.STOP-COVID Investigators Association Between Early Treatment With Tocilizumab and Mortality Among Critically Ill Patients With COVID-19. JAMA Intern. Med. 2021; 181 :41–51. [ PMC free article ] [ PubMed ] [ Google Scholar ]
- Hadjadj J., Yatim N., Barnabei L., Corneau A., Boussier J., Smith N., Péré H., Charbit B., Bondet V., Chenevier-Gobeaux C., et al. Impaired type I interferon activity and inflammatory responses in severe COVID-19 patients. Science. 2020; 369 :718–724. [ PMC free article ] [ PubMed ] [ Google Scholar ]
- Hamiel U., Kozer E., Youngster I. SARS-CoV-2 Rates in BCG-Vaccinated and Unvaccinated Young Adults. JAMA. 2020; 323 :2340–2341. [ PMC free article ] [ PubMed ] [ Google Scholar ]
- Han E., Tan M.M.J., Turk E., Sridhar D., Leung G.M., Shibuya K., Asgari N., Oh J., García-Basteiro A.L., Hanefeld J., et al. Lessons learnt from easing COVID-19 restrictions: an analysis of countries and regions in Asia Pacific and Europe. Lancet. 2020; 396 :1525–1534. [ PMC free article ] [ PubMed ] [ Google Scholar ]
- Hoang T.N., Pino M., Boddapati A.K., Viox E.G., Starke C.E., Upadhyay A.A., Gumber S., Nekorchuk M., Busman-Sahay K., Strongin Z., et al. Baricitinib treatment resolves lower-airway macrophage inflammation and neutrophil recruitment in SARS-CoV-2-infected rhesus macaques. Cell. 2021; 184 :460–475.e21. [ PMC free article ] [ PubMed ] [ Google Scholar ]
- Hoffmann M., Kleine-Weber H., Schroeder S., Krüger N., Herrler T., Erichsen S., Schiergens T.S., Herrler G., Wu N.-H., Nitsche A., et al. SARS-CoV-2 Cell Entry Depends on ACE2 and TMPRSS2 and Is Blocked by a Clinically Proven Protease Inhibitor. Cell. 2020; 181 :271–280.e8. [ PMC free article ] [ PubMed ] [ Google Scholar ]
- Ho Y.-H., Del Toro R., Rivera-Torres J., Rak J., Korn C., García-García A., Macías D., González-Gómez C., Del Monte A., Wittner M., et al. Remodeling of Bone Marrow Hematopoietic Stem Cell Niches Promotes Myeloid Cell Expansion during Premature or Physiological Aging. Cell Stem Cell. 2019; 25 :407–418.e6. [ PMC free article ] [ PubMed ] [ Google Scholar ]
- Huang E., Jordan S.C. Tocilizumab for Covid-19 - The Ongoing Search for Effective Therapies. N. Engl. J. Med. 2020; 383 :2387–2388. [ PMC free article ] [ PubMed ] [ Google Scholar ]
- Huang C., Wang Y., Li X., Ren L., Zhao J., Hu Y., Zhang L., Fan G., Xu J., Gu X., et al. Clinical features of patients infected with 2019 novel coronavirus in Wuhan, China. Lancet. 2020; 395 :497–506. [ PMC free article ] [ PubMed ] [ Google Scholar ]
- Iglesias-Julián E., López-Veloso M., de-la-Torre-Ferrera N., Barraza-Vengoechea J.C., Delgado-López P.D., Colazo-Burlato M., Ubeira-Iglesias M., Montero-Baladía M., Lorenzo-Martín A., Minguito-de-la-Iglesia J., et al. High dose subcutaneous Anakinra to treat acute respiratory distress syndrome secondary to cytokine storm syndrome among severely ill COVID-19 patients. J. Autoimmun. 2020; 115 :102537. [ PMC free article ] [ PubMed ] [ Google Scholar ]
- Imai M., Iwatsuki-Horimoto K., Hatta M., Loeber S., Halfmann P.J., Nakajima N., Watanabe T., Ujie M., Takahashi K., Ito M., et al. Syrian hamsters as a small animal model for SARS-CoV-2 infection and countermeasure development. Proc. Natl. Acad. Sci. USA. 2020; 117 :16587–16595. [ PMC free article ] [ PubMed ] [ Google Scholar ]
- Islam M.M., Poly T.N., Walther B.A., Yang H.C., Wang C.-W., Hsieh W.-S., Atique S., Salmani H., Alsinglawi B., Lin M.C., et al. Clinical Characteristics and Neonatal Outcomes of Pregnant Patients With COVID-19: A Systematic Review. Front. Med. (Lausanne) 2020; 7 :573468. [ PMC free article ] [ PubMed ] [ Google Scholar ]
- Israelow B., Song E., Mao T., Lu P., Meir A., Liu F., Alfajaro M.M., Wei J., Dong H., Homer R.J., et al. Mouse model of SARS-CoV-2 reveals inflammatory role of type I interferon signaling. J. Exp. Med. 2020; 217 :e20201241. [ PMC free article ] [ PubMed ] [ Google Scholar ]
- Jacot D., Greub G., Jaton K., Opota O. Viral load of SARS-CoV-2 across patients and compared to other respiratory viruses. Microbes Infect. 2020; 22 :617–621. [ PMC free article ] [ PubMed ] [ Google Scholar ]
- Karki R., Sharma B.R., Tuladhar S., Williams E.P., Zalduondo L., Samir P., Zheng M., Sundaram B., Banoth B., Malireddi R.K.S., et al. Synergism of TNF-α and IFN-γ Triggers Inflammatory Cell Death, Tissue Damage, and Mortality in SARS-CoV-2 Infection and Cytokine Shock Syndromes. Cell. 2021; 184 :149–168.e17. [ PMC free article ] [ PubMed ] [ Google Scholar ]
- Kaur U., Chakrabarti S.S., Ojha B., Pathak B.K., Singh A., Saso L., Chakrabarti S. Targeting host cell proteases to prevent SARS-CoV-2 invasion. Curr. Drug Targets. 2021; 22 :192–201. [ PubMed ] [ Google Scholar ]
- Kim D., Lee J.-Y., Yang J.-S., Kim J.W., Kim V.N., Chang H. The Architecture of SARS-CoV-2 Transcriptome. Cell. 2020; 181 :914–921.e10. [ PMC free article ] [ PubMed ] [ Google Scholar ]
- Kinoshita M., Tanaka M. Impact of Routine Infant BCG Vaccination on COVID-19. J. Infect. 2020; 81 :625–633. [ PMC free article ] [ PubMed ] [ Google Scholar ]
- Kleinnijenhuis J., Quintin J., Preijers F., Joosten L.A.B., Ifrim D.C., Saeed S., Jacobs C., van Loenhout J., de Jong D., Stunnenberg H.G., et al. Bacille Calmette-Guerin induces NOD2-dependent nonspecific protection from reinfection via epigenetic reprogramming of monocytes. Proc. Natl. Acad. Sci. USA. 2012; 109 :17537–17542. [ PMC free article ] [ PubMed ] [ Google Scholar ]
- Klok F.A., Kruip M.J.H.A., van der Meer N.J.M., Arbous M.S., Gommers D.A.M.P.J., Kant K.M., Kaptein F.H.J., van Paassen J., Stals M.A.M., Huisman M.V., Endeman H. Incidence of thrombotic complications in critically ill ICU patients with COVID-19. Thromb. Res. 2020; 191 :145–147. [ PMC free article ] [ PubMed ] [ Google Scholar ]
- Konno Y., Kimura I., Uriu K., Fukushi M., Irie T., Koyanagi Y., Sauter D., Gifford R.J., Nakagawa S., Sato K., USFQ-COVID19 Consortium SARS-CoV-2 ORF3b Is a Potent Interferon Antagonist Whose Activity Is Increased by a Naturally Occurring Elongation Variant. Cell Rep. 2020; 32 :108185. [ PMC free article ] [ PubMed ] [ Google Scholar ]
- Kooistra E.J., Waalders N.J.B., Grondman I., Janssen N.A.F., de Nooijer A.H., Netea M.G., van de Veerdonk F.L., Ewalds E., van der Hoeven J.G., Kox M., Pickkers P., RCI-COVID-19 Study Group Anakinra treatment in critically ill COVID-19 patients: a prospective cohort study. Crit. Care. 2020; 24 :688. [ PMC free article ] [ PubMed ] [ Google Scholar ]
- Korber B., Fischer W.M., Gnanakaran S., Yoon H., Theiler J., Abfalterer W., Hengartner N., Giorgi E.E., Bhattacharya T., Foley B., et al.Sheffield COVID-19 Genomics Group Tracking Changes in SARS-CoV-2 Spike: Evidence that D614G Increases Infectivity of the COVID-19 Virus. Cell. 2020; 182 :812–827.e19. [ PMC free article ] [ PubMed ] [ Google Scholar ]
- Kox M., Waalders N.J.B., Kooistra E.J., Gerretsen J., Pickkers P. Cytokine Levels in Critically Ill Patients With COVID-19 and Other Conditions. JAMA. 2020; 324 :1565–1567. [ PMC free article ] [ PubMed ] [ Google Scholar ]
- Kuri-Cervantes L., Pampena M.B., Meng W., Rosenfeld A.M., Ittner C.A.G., Weisman A.R., Agyekum R.S., Mathew D., Baxter A.E., Vella L.A., et al. Comprehensive mapping of immune perturbations associated with severe COVID-19. Sci. Immunol. 2020; 5 :eabd7114. [ PMC free article ] [ PubMed ] [ Google Scholar ]
- Lagunas-Rangel F.A. Neutrophil-to-lymphocyte ratio and lymphocyte-to-C-reactive protein ratio in patients with severe coronavirus disease 2019 (COVID-19): A meta-analysis. J. Med. Virol. 2020; 92 :1733–1734. [ PMC free article ] [ PubMed ] [ Google Scholar ]
- Laing A.G., Lorenc A., Del Molino Del Barrio I., Das A., Fish M., Monin L., Muñoz-Ruiz M., McKenzie D.R., Hayday T.S., Francos-Quijorna I., et al. A dynamic COVID-19 immune signature includes associations with poor prognosis. Nat. Med. 2020; 26 :1623–1635. [ PubMed ] [ Google Scholar ]
- Lee J.S., Shin E.-C. The type I interferon response in COVID-19: implications for treatment. Nat. Rev. Immunol. 2020; 20 :585–586. [ PMC free article ] [ PubMed ] [ Google Scholar ]
- Lee J.S., Park S., Jeong H.W., Ahn J.Y., Choi S.J., Lee H., Choi B., Nam S.K., Sa M., Kwon J.-S., et al. Immunophenotyping of COVID-19 and influenza highlights the role of type I interferons in development of severe COVID-19. Sci. Immunol. 2020; 5 :eabd1554. [ PMC free article ] [ PubMed ] [ Google Scholar ]
- Lei X., Dong X., Ma R., Wang W., Xiao X., Tian Z., Wang C., Wang Y., Li L., Ren L., et al. Activation and evasion of type I interferon responses by SARS-CoV-2. Nat. Commun. 2020; 11 :3810. [ PMC free article ] [ PubMed ] [ Google Scholar ]
- Liao M., Liu Y., Yuan J., Wen Y., Xu G., Zhao J., Cheng L., Li J., Wang X., Wang F., et al. Single-cell landscape of bronchoalveolar immune cells in patients with COVID-19. Nat. Med. 2020; 26 :842–844. [ PubMed ] [ Google Scholar ]
- Lindestam Arlehamn C.S., Sette A., Peters B. Lack of evidence for BCG vaccine protection from severe COVID-19. Proc. Natl. Acad. Sci. USA. 2020; 117 :25203–25204. [ PMC free article ] [ PubMed ] [ Google Scholar ]
- Lipsitch M., Grad Y.H., Sette A., Crotty S. Cross-reactive memory T cells and herd immunity to SARS-CoV-2. Nat. Rev. Immunol. 2020; 20 :709–713. [ PMC free article ] [ PubMed ] [ Google Scholar ]
- Liu Y., Du X., Chen J., Jin Y., Peng L., Wang H.H.X., Luo M., Chen L., Zhao Y. Neutrophil-to-lymphocyte ratio as an independent risk factor for mortality in hospitalized patients with COVID-19. J. Infect. 2020; 81 :e6–e12. [ PMC free article ] [ PubMed ] [ Google Scholar ]
- Li Q., Wu J., Nie J., Zhang L., Hao H., Liu S., Zhao C., Zhang Q., Liu H., Nie L., et al. The Impact of Mutations in SARS-CoV-2 Spike on Viral Infectivity and Antigenicity. Cell. 2020; 182 :1284–1294.e9. [ PMC free article ] [ PubMed ] [ Google Scholar ]
- Li W., Moore M.J., Vasilieva N., Sui J., Wong S.K., Berne M.A., Somasundaran M., Sullivan J.L., Luzuriaga K., Greenough T.C., et al. Angiotensin-converting enzyme 2 is a functional receptor for the SARS coronavirus. Nature. 2003; 426 :450–454. [ PMC free article ] [ PubMed ] [ Google Scholar ]
- Long Q.-X., Tang X.-J., Shi Q.-L., Li Q., Deng H.-J., Yuan J., Hu J.-L., Xu W., Zhang Y., Lv F.-J., et al. Clinical and immunological assessment of asymptomatic SARS-CoV-2 infections. Nat. Med. 2020; 26 :1200–1204. [ PubMed ] [ Google Scholar ]
- Loo Y.-M., Gale M., Jr. Immune signaling by RIG-I-like receptors. Immunity. 2011; 34 :680–692. [ PMC free article ] [ PubMed ] [ Google Scholar ]
- Lucas C., Wong P., Klein J., Castro T.B.R., Silva J., Sundaram M., Ellingson M.K., Mao T., Oh J.E., Israelow B., et al.Yale IMPACT Team Longitudinal analyses reveal immunological misfiring in severe COVID-19. Nature. 2020; 584 :463–469. [ PMC free article ] [ PubMed ] [ Google Scholar ]
- van der Made C.I., Simons A., Schuurs-Hoeijmakers J., van den Heuvel G., Mantere T., Kersten S., van Deuren R.C., Steehouwer M., van Reijmersdal S.V., Jaeger M., et al. Presence of Genetic Variants Among Young Men With Severe COVID-19. JAMA. 2020; 324 :1–11. [ PMC free article ] [ PubMed ] [ Google Scholar ]
- Mangalmurti N., Hunter C.A. Cytokine Storms: Understanding COVID-19. Immunity. 2020; 53 :19–25. [ PMC free article ] [ PubMed ] [ Google Scholar ]
- Mantovani A., Netea M.G. Trained Innate Immunity, Epigenetics, and Covid-19. N. Engl. J. Med. 2020; 383 :1078–1080. [ PubMed ] [ Google Scholar ]
- Marshall M. The lasting misery of coronavirus long-haulers. Nature. 2020; 585 :339–341. [ PubMed ] [ Google Scholar ]
- Mastellos D.C., Pires da Silva B.G.P., Fonseca B.A.L., Fonseca N.P., Auxiliadora-Martins M., Mastaglio S., Ruggeri A., Sironi M., Radermacher P., Chrysanthopoulou A., et al. Complement C3 vs C5 inhibition in severe COVID-19: Early clinical findings reveal differential biological efficacy. Clin. Immunol. 2020; 220 :108598. [ PMC free article ] [ PubMed ] [ Google Scholar ]
- Mateus J., Grifoni A., Tarke A., Sidney J., Ramirez S.I., Dan J.M., Burger Z.C., Rawlings S.A., Smith D.M., Phillips E., et al. Selective and cross-reactive SARS-CoV-2 T cell epitopes in unexposed humans. Science. 2020; 370 :89–94. [ PMC free article ] [ PubMed ] [ Google Scholar ]
- Mathew D., Giles J.R., Baxter A.E., Oldridge D.A., Greenplate A.R., Wu J.E., Alanio C., Kuri-Cervantes L., Pampena M.B., D’Andrea K., et al.UPenn COVID Processing Unit Deep immune profiling of COVID-19 patients reveals distinct immunotypes with therapeutic implications. Science. 2020; 369 :eabc8511. [ PMC free article ] [ PubMed ] [ Google Scholar ]
- Matsuyama S., Nagata N., Shirato K., Kawase M., Takeda M., Taguchi F. Efficient activation of the severe acute respiratory syndrome coronavirus spike protein by the transmembrane protease TMPRSS2. J. Virol. 2010; 84 :12658–12664. [ PMC free article ] [ PubMed ] [ Google Scholar ]
- Mazaleuskaya L., Veltrop R., Ikpeze N., Martin-Garcia J., Navas-Martin S. Protective role of Toll-like Receptor 3-induced type I interferon in murine coronavirus infection of macrophages. Viruses. 2012; 4 :901–923. [ PMC free article ] [ PubMed ] [ Google Scholar ]
- McCreary E.K., Pogue J.M. Coronavirus disease 2019 treatment: A review of early and emerging options. Open Forum Infect. Dis. 2020; 7 :ofaa105. [ PMC free article ] [ PubMed ] [ Google Scholar ]
- Merad M., Martin J.C. Pathological inflammation in patients with COVID-19: a key role for monocytes and macrophages. Nat. Rev. Immunol. 2020; 20 :355–362. [ PMC free article ] [ PubMed ] [ Google Scholar ]
- Mesev E.V., LeDesma R.A., Ploss A. Decoding type I and III interferon signalling during viral infection. Nat. Microbiol. 2019; 4 :914–924. [ PMC free article ] [ PubMed ] [ Google Scholar ]
- Messner C.B., Demichev V., Wendisch D., Michalick L., White M., Freiwald A., Textoris-Taube K., Vernardis S.I., Egger A.-S., Kreidl M., et al. Ultra-High-Throughput Clinical Proteomics Reveals Classifiers of COVID-19 Infection. Cell Syst. 2020; 11 :11–24.e4. [ PMC free article ] [ PubMed ] [ Google Scholar ]
- Middleton E.A., He X.-Y., Denorme F., Campbell R.A., Ng D., Salvatore S.P., Mostyka M., Baxter-Stoltzfus A., Borczuk A.C., Loda M., et al. Neutrophil extracellular traps contribute to immunothrombosis in COVID-19 acute respiratory distress syndrome. Blood. 2020; 136 :1169–1179. [ PMC free article ] [ PubMed ] [ Google Scholar ]
- Molony R.D., Nguyen J.T., Kong Y., Montgomery R.R., Shaw A.C., Iwasaki A. Aging impairs both primary and secondary RIG-I signaling for interferon induction in human monocytes. Sci. Signal. 2017; 10 :eaan2392. [ PMC free article ] [ PubMed ] [ Google Scholar ]
- Monneret G., Benlyamani I., Gossez M., Bermejo-Martin J.F., Martín-Fernandez M., Sesques P., Wallet F., Venet F. COVID-19: What type of cytokine storm are we dealing with? J. Med. Virol. 2021; 93 :197–198. [ PMC free article ] [ PubMed ] [ Google Scholar ]
- Moorlag S.J.C.F.M., van Deuren R.C., van Werkhoven C.H., Jaeger M., Debisarun P., Taks E., Mourits V.P., Koeken V.A.C.M., de Bree L.C.J., Ten Doesschate T., et al. Safety and COVID-19 Symptoms in Individuals Recently Vaccinated with BCG: a Retrospective Cohort Study. Cell Rep. Med. 2020; 1 :100073. [ PMC free article ] [ PubMed ] [ Google Scholar ]
- Morens D.M., Fauci A.S. Emerging Pandemic Diseases: How We Got to COVID-19. Cell. 2020; 182 :1077–1092. [ PMC free article ] [ PubMed ] [ Google Scholar ]
- Morens D.M., Daszak P., Taubenberger J.K. Escaping Pandora’s Box - Another Novel Coronavirus. N. Engl. J. Med. 2020; 382 :1293–1295. [ PubMed ] [ Google Scholar ]
- Mulchandani R., Lyngdoh T., Kakkar A.K. Deciphering the COVID-19 cytokine storm: Systematic review and meta-analysis. Eur. J. Clin. Invest. 2021; 51 :e13429. [ PMC free article ] [ PubMed ] [ Google Scholar ]
- Muus C., Luecken M.D., Eraslan G., Sikkema L., Waghray A., Heimberg G., Kobayashi Y., Vaishnav E.D., Subramanian A., Smillie C., et al. Integrated analyses of single-cell atlases reveal age, gender, and smoking status associations with cell type-specific expression of mediators of SARS-CoV-2 viral entry and highlights inflammatory programs in putative target cells. Nat Med. 2021 doi: 10.1038/s41591-020-01227-z. [ CrossRef ] [ Google Scholar ]
- Netea M.G., Domínguez-Andrés J., Barreiro L.B., Chavakis T., Divangahi M., Fuchs E., Joosten L.A.B., van der Meer J.W.M., Mhlanga M.M., Mulder W.J.M., et al. Defining trained immunity and its role in health and disease. Nat. Rev. Immunol. 2020; 20 :375–388. [ PMC free article ] [ PubMed ] [ Google Scholar ]
- Netea M.G., Giamarellos-Bourboulis E.J., Domínguez-Andrés J., Curtis N., van Crevel R., van de Veerdonk F.L., Bonten M. Trained Immunity: a Tool for Reducing Susceptibility to and the Severity of SARS-CoV-2 Infection. Cell. 2020; 181 :969–977. [ PMC free article ] [ PubMed ] [ Google Scholar ]
- Newton A.H., Cardani A., Braciale T.J. The host immune response in respiratory virus infection: balancing virus clearance and immunopathology. Semin. Immunopathol. 2016; 38 :471–482. [ PMC free article ] [ PubMed ] [ Google Scholar ]
- Ni L., Ye F., Cheng M.-L., Feng Y., Deng Y.-Q., Zhao H., Wei P., Ge J., Gou M., Li X., et al. Detection of SARS-CoV-2-Specific Humoral and Cellular Immunity in COVID-19 Convalescent Individuals. Immunity. 2020; 52 :971–977.e3. [ PMC free article ] [ PubMed ] [ Google Scholar ]
- Nowill A.E., de Campos-Lima P.O. Immune Response Resetting as a Novel Strategy to Overcome SARS-CoV-2-Induced Cytokine Storm. J. Immunol. 2020; 205 :2566–2575. [ PubMed ] [ Google Scholar ]
- O’Neill L.A.J., Netea M.G. BCG-induced trained immunity: can it offer protection against COVID-19? Nat. Rev. Immunol. 2020; 20 :335–337. [ PMC free article ] [ PubMed ] [ Google Scholar ]
- Onabajo O.O., Banday A.R., Stanifer M.L., Yan W., Obajemu A., Santer D.M., Florez-Vargas O., Piontkivska H., Vargas J.M., Ring T.J., et al. Interferons and viruses induce a novel truncated ACE2 isoform and not the full-length SARS-CoV-2 receptor. Nat. Genet. 2020; 52 :1283–1293. [ PMC free article ] [ PubMed ] [ Google Scholar ]
- Oude Munnink B.B., Nieuwenhuijse D.F., Stein M., O’Toole Á., Haverkate M., Mollers M., Kamga S.K., Schapendonk C., Pronk M., Lexmond P., et al.Dutch-Covid-19 response team Rapid SARS-CoV-2 whole-genome sequencing and analysis for informed public health decision-making in the Netherlands. Nat. Med. 2020; 26 :1405–1410. [ PubMed ] [ Google Scholar ]
- Ou X., Liu Y., Lei X., Li P., Mi D., Ren L., Guo L., Guo R., Chen T., Hu J., et al. Characterization of spike glycoprotein of SARS-CoV-2 on virus entry and its immune cross-reactivity with SARS-CoV. Nat. Commun. 2020; 11 :1620. [ PMC free article ] [ PubMed ] [ Google Scholar ]
- Ovsyannikova I.G., Haralambieva I.H., Crooke S.N., Poland G.A., Kennedy R.B. The role of host genetics in the immune response to SARS-CoV-2 and COVID-19 susceptibility and severity. Immunol. Rev. 2020; 296 :205–219. [ PMC free article ] [ PubMed ] [ Google Scholar ]
- Pairo-Castineira E., Clohisey S., Klaric L., Bretherick A.D., Rawlik K., Pasko D., Walker S., Parkinson N., Fourman M.H., Russell C.D., et al. Genetic mechanisms of critical illness in Covid-19. Nature. 2020 doi: 10.1038/s41586-020-03065-y. Published online December 11, 2020. [ PubMed ] [ CrossRef ] [ Google Scholar ]
- Patil S., Fribourg M., Ge Y., Batish M., Tyagi S., Hayot F., Sealfon S.C. Single-cell analysis shows that paracrine signaling by first responder cells shapes the interferon-β response to viral infection. Sci. Signal. 2015; 8 :ra16. [ PubMed ] [ Google Scholar ]
- Pence B.D. Severe COVID-19 and aging: are monocytes the key? Geroscience. 2020; 42 :1051–1061. [ PMC free article ] [ PubMed ] [ Google Scholar ]
- Pircher J., Engelmann B., Massberg S., Schulz C. Platelet-Neutrophil Crosstalk in Atherothrombosis. Thromb. Haemost. 2019; 119 :1274–1282. [ PubMed ] [ Google Scholar ]
- Polack F.P., Thomas S.J., Kitchin N., Absalon J., Gurtman A., Lockhart S., Perez J.L., Pérez Marc G., Moreira E.D., Zerbini C., et al.C4591001 Clinical Trial Group Safety and Efficacy of the BNT162b2 mRNA Covid-19 Vaccine. N. Engl. J. Med. 2020; 383 :2603–2615. [ PMC free article ] [ PubMed ] [ Google Scholar ]
- Prokunina-Olsson L., Alphonse N., Dickenson R.E., Durbin J.E., Glenn J.S., Hartmann R., Kotenko S.V., Lazear H.M., O’Brien T.R., Odendall C., et al. COVID-19 and emerging viral infections: The case for interferon lambda. J. Exp. Med. 2020; 217 :e20200653. [ PMC free article ] [ PubMed ] [ Google Scholar ]
- Qin C., Zhou L., Hu Z., Zhang S., Yang S., Tao Y., Xie C., Ma K., Shang K., Wang W., Tian D.S. Dysregulation of Immune Response in Patients With Coronavirus 2019 (COVID-19) in Wuhan, China. Clin. Infect. Dis. 2020; 71 :762–768. [ PMC free article ] [ PubMed ] [ Google Scholar ]
- Qi F., Qian S., Zhang S., Zhang Z. Single cell RNA sequencing of 13 human tissues identify cell types and receptors of human coronaviruses. Biochem. Biophys. Res. Commun. 2020; 526 :135–140. [ PMC free article ] [ PubMed ] [ Google Scholar ]
- Rajewsky N., Almouzni G., Gorski S.A., Aerts S., Amit I., Bertero M.G., Bock C., Bredenoord A.L., Cavalli G., Chiocca S., et al.LifeTime Community Working Groups LifeTime and improving European healthcare through cell-based interceptive medicine. Nature. 2020; 587 :377–386. [ PMC free article ] [ PubMed ] [ Google Scholar ]
- Rand U., Rinas M., Schwerk J., Nöhren G., Linnes M., Kröger A., Flossdorf M., Kály-Kullai K., Hauser H., Höfer T., Köster M. Multi-layered stochasticity and paracrine signal propagation shape the type-I interferon response. Mol. Syst. Biol. 2012; 8 :584. [ PMC free article ] [ PubMed ] [ Google Scholar ]
- RECOVERY Collaborative Group. Horby P., Lim W.S., Emberson J.R., Mafham M., Bell J.L., Linsell L., Staplin N., Brightling C., Ustianowski A., et al. Dexamethasone in Hospitalized Patients with Covid-19 - Preliminary Report. N. Engl. J. Med. 2021; 384 :693–704. [ PMC free article ] [ PubMed ] [ Google Scholar ]
- Remy K.E., Mazer M., Striker D.A., Ellebedy A.H., Walton A.H., Unsinger J., Blood T.M., Mudd P.A., Yi D.J., Mannion D.A., et al. Severe immunosuppression and not a cytokine storm characterizes COVID-19 infections. JCI Insight. 2020; 5 :e140329. [ PMC free article ] [ PubMed ] [ Google Scholar ]
- de la Rica R., Borges M., Gonzalez-Freire M. COVID-19: In the Eye of the Cytokine Storm. Front. Immunol. 2020; 11 :558898. [ PMC free article ] [ PubMed ] [ Google Scholar ]
- Richardson S., Hirsch J.S., Narasimhan M., Crawford J.M., McGinn T., Davidson K.W., Barnaby D.P., Becker L.B., Chelico J.D., Cohen S.L., et al.the Northwell COVID-19 Research Consortium Presenting Characteristics, Comorbidities, and Outcomes Among 5700 Patients Hospitalized With COVID-19 in the New York City Area. JAMA. 2020; 323 :2052–2059. [ PMC free article ] [ PubMed ] [ Google Scholar ]
- Riva G., Nasillo V., Tagliafico E., Trenti T., Comoli P., Luppi M. COVID-19: more than a cytokine storm. Crit. Care. 2020; 24 :549. [ PMC free article ] [ PubMed ] [ Google Scholar ]
- Rodriguez L., Pekkarinen P.T., Lakshmikanth T., Tan Z., Consiglio C.R., Pou C., Chen Y., Mugabo C.H., Nguyen N.A., Nowlan K., et al. Systems-Level Immunomonitoring from Acute to Recovery Phase of Severe COVID-19. Cell Rep. Med. 2020; 1 :100078. [ PMC free article ] [ PubMed ] [ Google Scholar ]
- Sakaguchi W., Kubota N., Shimizu T., Saruta J., Fuchida S., Kawata A., Yamamoto Y., Sugimoto M., Yakeishi M., Tsukinoki K. Existence of SARS-CoV-2 Entry Molecules in the Oral Cavity. Int. J. Mol. Sci. 2020; 21 :6000. [ PMC free article ] [ PubMed ] [ Google Scholar ]
- Salisbury H. Helen Salisbury: When will we be well again? BMJ. 2020; 369 :m2490. [ PubMed ] [ Google Scholar ]
- Sa Ribero M., Jouvenet N., Dreux M., Nisole S. Interplay between SARS-CoV-2 and the type I interferon response. PLoS Pathog. 2020; 16 :e1008737. [ PMC free article ] [ PubMed ] [ Google Scholar ]
- Schulte-Schrepping J., Reusch N., Paclik D., Baßler K., Schlickeiser S., Zhang B., Krämer B., Krammer T., Brumhard S., Bonaguro L., et al.Deutsche COVID-19 OMICS Initiative (DeCOI) Severe COVID-19 Is Marked by a Dysregulated Myeloid Cell Compartment. Cell. 2020; 182 :1419–1440.e23. [ PMC free article ] [ PubMed ] [ Google Scholar ]
- Sette A., Crotty S. Adaptive immunity to SARS-CoV-2 and COVID-19. Cell. 2021; 184 :861–880. [ PMC free article ] [ PubMed ] [ Google Scholar ]
- Severe Covid-19 GWAS Group. Ellinghaus D., Degenhardt F., Bujanda L., Buti M., Albillos A., Invernizzi P., Fernández J., Prati D., Baselli G., et al. Genomewide Association Study of Severe Covid-19 with Respiratory Failure. N. Engl. J. Med. 2020; 383 :1522–1534. [ PMC free article ] [ PubMed ] [ Google Scholar ]
- Shang J., Wan Y., Luo C., Ye G., Geng Q., Auerbach A., Li F. Cell entry mechanisms of SARS-CoV-2. Proc. Natl. Acad. Sci. USA. 2020; 117 :11727–11734. [ PMC free article ] [ PubMed ] [ Google Scholar ]
- Shaw A.C., Goldstein D.R., Montgomery R.R. Age-dependent dysregulation of innate immunity. Nat. Rev. Immunol. 2013; 13 :875–887. [ PMC free article ] [ PubMed ] [ Google Scholar ]
- Shen B., Yi X., Sun Y., Bi X., Du J., Zhang C., Quan S., Zhang F., Sun R., Qian L., et al. Proteomic and Metabolomic Characterization of COVID-19 Patient Sera. Cell. 2020; 182 :59–72.e15. [ PMC free article ] [ PubMed ] [ Google Scholar ]
- Shu T., Ning W., Wu D., Xu J., Han Q., Huang M., Zou X., Yang Q., Yuan Y., Bie Y., et al. Plasma Proteomics Identify Biomarkers and Pathogenesis of COVID-19. Immunity. 2020; 53 :1108–1122.e5. [ PMC free article ] [ PubMed ] [ Google Scholar ]
- Silvin A., Chapuis N., Dunsmore G., Goubet A.-G., Dubuisson A., Derosa L., Almire C., Hénon C., Kosmider O., Droin N., et al. Elevated Calprotectin and Abnormal Myeloid Cell Subsets Discriminate Severe from Mild COVID-19. Cell. 2020; 182 :1401–1418.e18. [ PMC free article ] [ PubMed ] [ Google Scholar ]
- Singh S., Maurya R.P., Singh R.K. “Trained immunity” from Mycobacterium spp. exposure or BCG vaccination and COVID-19 outcomes. PLoS Pathog. 2020; 16 :e1008969. [ PMC free article ] [ PubMed ] [ Google Scholar ]
- Sinha P., Matthay M.A., Calfee C.S. Is a “Cytokine Storm” Relevant to COVID-19? JAMA Intern. Med. 2020; 180 :1152–1154. [ PubMed ] [ Google Scholar ]
- Smits S.L., de Lang A., van den Brand J.M.A., Leijten L.M., van IJcken W.F., Eijkemans M.J.C., van Amerongen G., Kuiken T., Andeweg A.C., Osterhaus A.D.M.E., Haagmans B.L. Exacerbated innate host response to SARS-CoV in aged non-human primates. PLoS Pathog. 2010; 6 :e1000756. [ PMC free article ] [ PubMed ] [ Google Scholar ]
- Snijder E.J., Limpens R.W.A.L., de Wilde A.H., de Jong A.W.M., Zevenhoven-Dobbe J.C., Maier H.J., Faas F.F.G.A., Koster A.J., Bárcena M. A unifying structural and functional model of the coronavirus replication organelle: Tracking down RNA synthesis. PLoS Biol. 2020; 18 :e3000715. [ PMC free article ] [ PubMed ] [ Google Scholar ]
- Stone J.H., Frigault M.J., Serling-Boyd N.J., Fernandes A.D., Harvey L., Foulkes A.S., Horick N.K., Healy B.C., Shah R., Bensaci A.M., et al.BACC Bay Tocilizumab Trial Investigators Efficacy of Tocilizumab in Patients Hospitalized with Covid-19. N. Engl. J. Med. 2020; 383 :2333–2344. [ PMC free article ] [ PubMed ] [ Google Scholar ]
- Sungnak W., Huang N., Bécavin C., Berg M., Queen R., Litvinukova M., Talavera-López C., Maatz H., Reichart D., Sampaziotis F., et al.HCA Lung Biological Network SARS-CoV-2 entry factors are highly expressed in nasal epithelial cells together with innate immune genes. Nat. Med. 2020; 26 :681–687. [ PMC free article ] [ PubMed ] [ Google Scholar ]
- Su Y., Chen D., Yuan D., Lausted C., Choi J., Dai C.L., Voillet V., Duvvuri V.R., Scherler K., Troisch P., et al.ISB-Swedish COVID19 Biobanking Unit Multi-Omics Resolves a Sharp Disease-State Shift between Mild and Moderate COVID-19. Cell. 2020; 183 :1479–1495.e20. [ PMC free article ] [ PubMed ] [ Google Scholar ]
- Takahashi T., Ellingson M.K., Wong P., Israelow B., Lucas C., Klein J., Silva J., Mao T., Oh J.E., Tokuyama M., et al.Yale IMPACT Research Team Sex differences in immune responses that underlie COVID-19 disease outcomes. Nature. 2020; 588 :315–320. [ PMC free article ] [ PubMed ] [ Google Scholar ]
- Talemi S.R., Höfer T. Antiviral interferon response at single-cell resolution. Immunol. Rev. 2018; 285 :72–80. [ PubMed ] [ Google Scholar ]
- Tang Y., Liu J., Zhang D., Xu Z., Ji J., Wen C. Cytokine Storm in COVID-19: The Current Evidence and Treatment Strategies. Front. Immunol. 2020; 11 :1708. [ PMC free article ] [ PubMed ] [ Google Scholar ]
- Taquet M., Luciano S., Geddes J.R., Harrison P.J. Bidirectional associations between COVID-19 and psychiatric disorder: retrospective cohort studies of 62 354 COVID-19 cases in the USA. Lancet Psychiatry. 2021; 8 :130–140. [ PMC free article ] [ PubMed ] [ Google Scholar ]
- Tomazini B.M., Maia I.S., Cavalcanti A.B., Berwanger O., Rosa R.G., Veiga V.C., Avezum A., Lopes R.D., Bueno F.R., Silva M.V.A.O., et al.COALITION COVID-19 Brazil III Investigators Effect of Dexamethasone on Days Alive and Ventilator-Free in Patients With Moderate or Severe Acute Respiratory Distress Syndrome and COVID-19: The CoDEX Randomized Clinical Trial. JAMA. 2020; 324 :1307–1316. [ PMC free article ] [ PubMed ] [ Google Scholar ]
- Vabret N., Britton G.J., Gruber C., Hegde S., Kim J., Kuksin M., Levantovsky R., Malle L., Moreira A., Park M.D., et al.Sinai Immunology Review Project Immunology of COVID-19: Current State of the Science. Immunity. 2020; 52 :910–941. [ PMC free article ] [ PubMed ] [ Google Scholar ]
- van de Veerdonk F.L., Kouijzer I.J.E., de Nooijer A.H., van der Hoeven H.G., Maas C., Netea M.G., Brüggemann R.J.M. Outcomes Associated With Use of a Kinin B2 Receptor Antagonist Among Patients With COVID-19. JAMA Netw. Open. 2020; 3 :e2017708. [ PMC free article ] [ PubMed ] [ Google Scholar ]
- Veras F.P., Pontelli M.C., Silva C.M., Toller-Kawahisa J.E., de Lima M., Nascimento D.C., Schneider A.H., Caetité D., Tavares L.A., Paiva I.M., et al. SARS-CoV-2-triggered neutrophil extracellular traps mediate COVID-19 pathology. J. Exp. Med. 2020; 217 :e20201129. [ PMC free article ] [ PubMed ] [ Google Scholar ]
- Wang J., Jiang M., Chen X., Montaner L.J. Cytokine storm and leukocyte changes in mild versus severe SARS-CoV-2 infection: Review of 3939 COVID-19 patients in China and emerging pathogenesis and therapy concepts. J. Leukoc. Biol. 2020; 108 :17–41. [ PMC free article ] [ PubMed ] [ Google Scholar ]
- Wang N., Zhan Y., Zhu L., Hou Z., Liu F., Song P., Qiu F., Wang X., Zou X., Wan D., et al. Retrospective Multicenter Cohort Study Shows Early Interferon Therapy Is Associated with Favorable Clinical Responses in COVID-19 Patients. Cell Host Microbe. 2020; 28 :455–464.e2. [ PMC free article ] [ PubMed ] [ Google Scholar ]
- Song E., Zhang C., Israelow B., Lu-Culligan A., Prado A.V., Skriabine S., Lu P., Weizman O.-E., Liu F., Dai Y., et al. Neuroinvasion of SARS-CoV-2 in human and mouse brain. J Exp Med. 2021 doi: 10.1084/jem.20202135. [ PMC free article ] [ PubMed ] [ CrossRef ] [ Google Scholar ]
- WHO Rapid Evidence Appraisal for COVID-19 Therapies (REACT) Working Group. Sterne J.A.C., Murthy S., Diaz J.V., Slutsky A.S., Villar J., Angus D.C., Annane D., Azevedo L.C.P., Berwanger O., et al. Association Between Administration of Systemic Corticosteroids and Mortality Among Critically Ill Patients With COVID-19: A meta-analysis. JAMA. 2020; 324 :1330–1341. [ PMC free article ] [ PubMed ] [ Google Scholar ]
- Wilk A.J., Rustagi A., Zhao N.Q., Roque J., Martínez-Colón G.J., McKechnie J.L., Ivison G.T., Ranganath T., Vergara R., Hollis T., et al. A single-cell atlas of the peripheral immune response in patients with severe COVID-19. Nat. Med. 2020; 26 :1070–1076. [ PMC free article ] [ PubMed ] [ Google Scholar ]
- Williamson E.J., Walker A.J., Bhaskaran K., Bacon S., Bates C., Morton C.E., Curtis H.J., Mehrkar A., Evans D., Inglesby P., et al. Factors associated with COVID-19-related death using OpenSAFELY. Nature. 2020; 584 :430–436. [ PMC free article ] [ PubMed ] [ Google Scholar ]
- Wimmers F., Subedi N., van Buuringen N., Heister D., Vivié J., Beeren-Reinieren I., Woestenenk R., Dolstra H., Piruska A., Jacobs J.F.M., et al. Single-cell analysis reveals that stochasticity and paracrine signaling control interferon-alpha production by plasmacytoid dendritic cells. Nat. Commun. 2018; 9 :3317. [ PMC free article ] [ PubMed ] [ Google Scholar ]
- Wu D., Yang X.O. TH17 responses in cytokine storm of COVID-19: An emerging target of JAK2 inhibitor Fedratinib. J. Microbiol. Immunol. Infect. 2020; 53 :368–370. [ PMC free article ] [ PubMed ] [ Google Scholar ]
- Wu F., Zhao S., Yu B., Chen Y.-M., Wang W., Song Z.-G., Hu Y., Tao Z.-W., Tian J.-H., Pei Y.-Y., et al. A new coronavirus associated with human respiratory disease in China. Nature. 2020; 579 :265–269. [ PMC free article ] [ PubMed ] [ Google Scholar ]
- Xia H., Cao Z., Xie X., Zhang X., Chen J.Y.-C., Wang H., Menachery V.D., Rajsbaum R., Shi P.-Y. Evasion of Type I Interferon by SARS-CoV-2. Cell Rep. 2020; 33 :108234. [ PMC free article ] [ PubMed ] [ Google Scholar ]
- Xiong Y., Liu Y., Cao L., Wang D., Guo M., Jiang A., Guo D., Hu W., Yang J., Tang Z., et al. Transcriptomic characteristics of bronchoalveolar lavage fluid and peripheral blood mononuclear cells in COVID-19 patients. Emerg. Microbes Infect. 2020; 9 :761–770. [ PMC free article ] [ PubMed ] [ Google Scholar ]
- Xu R., Ekiert D.C., Krause J.C., Hai R., Crowe J.E., Jr., Wilson I.A. Structural basis of preexisting immunity to the 2009 H1N1 pandemic influenza virus. Science. 2010; 328 :357–360. [ PMC free article ] [ PubMed ] [ Google Scholar ]
- Yang D., Chu H., Hou Y., Chai Y., Shuai H., Lee A.C.-Y., Zhang X., Wang Y., Hu B., Huang X., et al. Attenuated Interferon and Proinflammatory Response in SARS-CoV-2-Infected Human Dendritic Cells Is Associated With Viral Antagonism of STAT1 Phosphorylation. J. Infect. Dis. 2020; 222 :734–745. [ PMC free article ] [ PubMed ] [ Google Scholar ]
- Yang Y., Shen C., Li J., Yuan J., Wei J., Huang F., Wang F., Li G., Li Y., Xing L., et al. Plasma IP-10 and MCP-3 levels are highly associated with disease severity and predict the progression of COVID-19. J. Allergy Clin. Immunol. 2020; 146 :119–127.e4. [ PMC free article ] [ PubMed ] [ Google Scholar ]
- Yao H., Song Y., Chen Y., Wu N., Xu J., Sun C., Zhang J., Weng T., Zhang Z., Wu Z., et al. Molecular Architecture of the SARS-CoV-2 Virus. Cell. 2020; 183 :730–738.e13. [ PMC free article ] [ PubMed ] [ Google Scholar ]
- Yu P., Qi F., Xu Y., Li F., Liu P., Liu J., Bao L., Deng W., Gao H., Xiang Z., et al. Age-related rhesus macaque models of COVID-19. Animal Model. Exp. Med. 2020; 3 :93–97. [ PMC free article ] [ PubMed ] [ Google Scholar ]
- Zeberg H., Pääbo S. The major genetic risk factor for severe COVID-19 is inherited from Neanderthals. Nature. 2020; 587 :610–612. [ PubMed ] [ Google Scholar ]
- Zhang Y.-Z., Holmes E.C. A Genomic Perspective on the Origin and Emergence of SARS-CoV-2. Cell. 2020; 181 :223–227. [ PMC free article ] [ PubMed ] [ Google Scholar ]
- Zhang Q., Bastard P., Liu Z., Le Pen J., Moncada-Velez M., Chen J., Ogishi M., Sabli I.K.D., Hodeib S., Korol C., et al.COVID-STORM Clinicians. COVID Clinicians. Imagine COVID Group. French COVID Cohort Study Group. CoV-Contact Cohort. Amsterdam UMC Covid-19 Biobank. COVID Human Genetic Effort. NIAID-USUHS/TAGC COVID Immunity Group Inborn errors of type I IFN immunity in patients with life-threatening COVID-19. Science. 2020; 370 :eabd4570. [ PMC free article ] [ PubMed ] [ Google Scholar ]
- Zhang S., Liu Y., Wang X., Yang L., Li H., Wang Y., Liu M., Zhao X., Xie Y., Yang Y., et al. SARS-CoV-2 binds platelet ACE2 to enhance thrombosis in COVID-19. J. Hematol. Oncol. 2020; 13 :120. [ PMC free article ] [ PubMed ] [ Google Scholar ]
- Zhao J., Zhao J., Legge K., Perlman S. Age-related increases in PGD(2) expression impair respiratory DC migration, resulting in diminished T cell responses upon respiratory virus infection in mice. J. Clin. Invest. 2011; 121 :4921–4930. [ PMC free article ] [ PubMed ] [ Google Scholar ]
- Zheng M., Gao Y., Wang G., Song G., Liu S., Sun D., Xu Y., Tian Z. Functional exhaustion of antiviral lymphocytes in COVID-19 patients. Cell. Mol. Immunol. 2020; 17 :533–535. [ PMC free article ] [ PubMed ] [ Google Scholar ]
- Zheng Y., Liu X., Le W., Xie L., Li H., Wen W., Wang S., Ma S., Huang Z., Ye J., et al. A human circulating immune cell landscape in aging and COVID-19. Protein Cell. 2020; 11 :740–770. [ PMC free article ] [ PubMed ] [ Google Scholar ]
- Zhou Q., Chen V., Shannon C.P., Wei X.-S., Xiang X., Wang X., Wang Z.-H., Tebbutt S.J., Kollmann T.R., Fish E.N. Interferon-α2b Treatment for COVID-19. Front. Immunol. 2020; 11 :1061. [ PMC free article ] [ PubMed ] [ Google Scholar ]
- Zhou R., To K.K.-W., Wong Y.-C., Liu L., Zhou B., Li X., Huang H., Mo Y., Luk T.-Y., Lau T.T.-K., et al. Acute SARS-CoV-2 Infection Impairs Dendritic Cell and T Cell Responses. Immunity. 2020; 53 :864–877.e5. [ PMC free article ] [ PubMed ] [ Google Scholar ]
- Ziegler C.G.K., Allon S.J., Nyquist S.K., Mbano I.M., Miao V.N., Tzouanas C.N., Cao Y., Yousif A.S., Bals J., Hauser B.M., et al.HCA Lung Biological Network SARS-CoV-2 Receptor ACE2 Is an Interferon-Stimulated Gene in Human Airway Epithelial Cells and Is Detected in Specific Cell Subsets across Tissues. Cell. 2020; 181 :1016–1035.e19. [ PMC free article ] [ PubMed ] [ Google Scholar ]
- Zou X., Chen K., Zou J., Han P., Hao J., Han Z. Single-cell RNA-seq data analysis on the receptor ACE2 expression reveals the potential risk of different human organs vulnerable to 2019-nCoV infection. Front. Med. 2020; 14 :185–192. [ PMC free article ] [ PubMed ] [ Google Scholar ]
- Biology Article
What is Immunity?
Immunity is the ability of the body to defend itself against disease-causing organisms. Everyday our body comes in contact with several pathogens, but only a few results into diseases. The reason is, our body has the ability to release antibodies against these pathogens and protects the body against diseases. This defence mechanism is called immunity.
Table Of Contents
- Types Of Immunity
Innate Immunity
Acquired immunity.
- Autoimmunity
Immune System
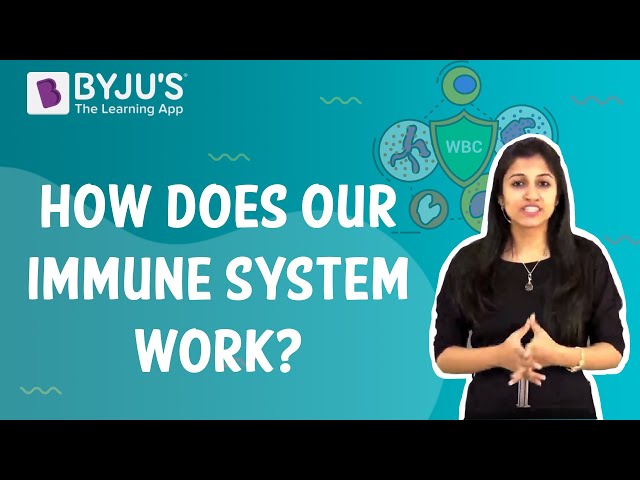
Types of Immunity
There are two major types of immunity:
Innate Immunity or Natural or Non-specific Immunity.
Acquired Immunity or Adaptive Immunity.
This type of immunity is present in an organism by birth.
This is activated immediately when the pathogen attacks. Innate immunity includes certain barriers and defence mechanisms that keep foreign particles out of the body.
Innate immunity refers to the body’s defence system.
This immunity helps us by providing the natural resistance components including salivary enzymes, natural killer cells, intact skin and neutrophils, etc. which produce an initial response against the infections at birth prior to exposure to a pathogen or antigens.
It is a long-term immunity in which our body produces the antibodies on its own. Our body has few natural barriers to prevent the entry of pathogens.
Also Read: Antimicrobial Resistance
Types of Barriers
The four types of barriers are:
Physical barrier
These include the skin, body hair, cilia, eyelashes, the respiratory tract, and the gastrointestinal tract. These form the first line of defence.
The skin does more than providing us with fair or dark complexions. Our skin acts as a physical barrier to the entry of pathogens. The mucus coating in our nose and ear is a protective barrier which traps the pathogen before it gets inside.
Physiological barriers
We know that our stomach uses hydrochloric acid to break down the food molecules. Due to such a strongly acidic environment, most of the germs that enter our body along with the food are killed before the further process is carried on.
Saliva in our mouth and tears in our eyes also have the antibiotic property that does not allow the growth of pathogens even though they are exposed all day.
Cellular barriers
In spite of the physical and physiological barriers, certain pathogens manage to enter our body. The cells involved in this barrier are leukocytes (WBC), neutrophils, lymphocytes, basophil, eosinophil, and monocytes. All these cells are all present in the blood and tissues.
Cytokine barriers
The cells in our body are smarter than we give them credit for. For instance, in case a cell in our body experiences a virus invasion, it automatically secretes proteins called interferons which forms a coating around the infected cell and prevents the cells around it from further infections.
Cells Involved In Innate Immunity
Phagocytes : These circulate through the body and look for any foreign substance. They engulf and destroy it defending the body against that pathogen.
Macrophages : These have the ability to move across the walls of the circulatory system. They release certain signals as cytokines to recruit other cells at the site of infections.
Mast Cells : These are important for healing wounds and defence against infections.
Neutrophils : These contain granules that are toxic in nature and kill any pathogen that comes in contact.
Eosinophils : These contain highly toxic proteins that kill any bacteria or parasite in contact.
Basophils : These attack multicellular parasites. Like the mast cells, these release histamine.
Natural Killer Cells : These stop the spread of infections by destroying the infected host cells.
Dendritic Cells : These are located in the tissues that are the points for initial infections. These cells sense the infection and send the message to the rest of the immune system by antigen presentation.
Also Read: Diseases
Acquired immunity or adaptive immunity is the immunity that our body acquires or gains over time. Unlike the innate immunity, this is not present by birth.
The ability of the immune system to adapt itself to disease and to generate pathogen-specific immunity is termed as acquired immunity. It is also known as adaptive immunity.
An individual acquires the immunity after the birth, hence is called as the acquired immunity.
It is specific and mediated by antibodies or lymphocytes which make the antigen harmless.
The main function of acquired immunity is to relieve the victim of the infectious disease and also prevent its attack in future.
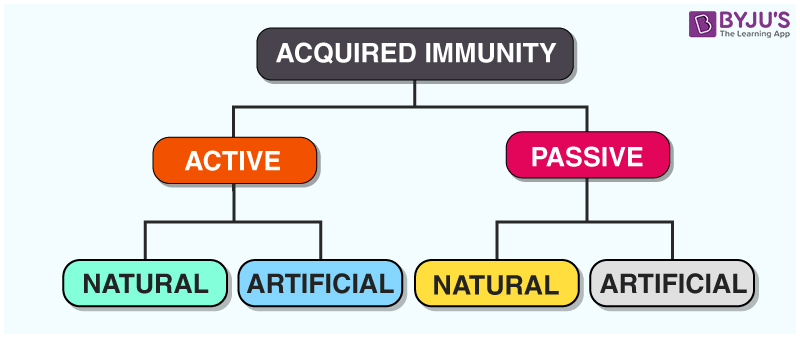
The immune system of our body identifies the pathogens which have encountered in the past. It is mainly caused when a person comes in contact with the pathogen or its antigen.
Our body starts producing antibodies to engulf the pathogen and destroy its antigen.
When it encounters for the first time, it is called a primary response. Once a body gets used to these pathogens, antibodies are ready to attack them for the second time and are known as naturally acquired immunity.
The acquired immunity in our body has certain special features.
Features of Acquired Immunity
Specificity : Our body has the ability to differentiate between different types of pathogens, whether it is harmful or not, and devise ways to destroy them.
Diversity : Our body can detect vast varieties of pathogens, ranging from protozoa to viruses.
Differentiate between self and non-self : Our body has the unique ability to differentiate between its own cells and foreign cells. It immediately starts rejecting any foreign cell in the body.
Memory : Once our body encounters a pathogen, it activates the immune system to destroy it. It also remembers what antibodies were released in response to that pathogen, so that, the next time it enters, a similar procedure is followed by the body to eliminate it.
Cells Involved in Acquired Immunity
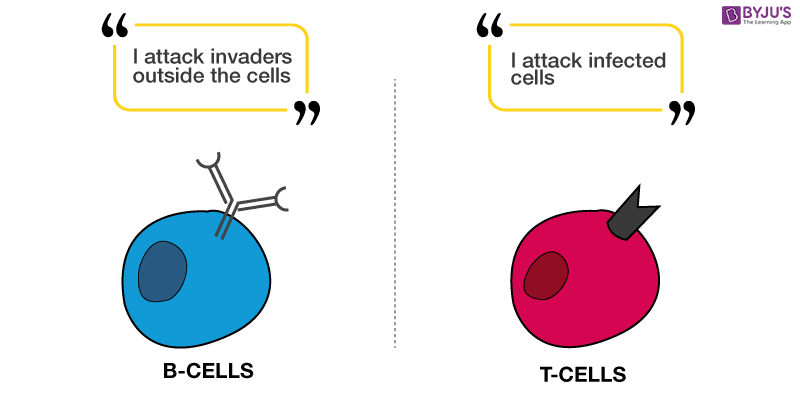
They develop in the bone marrow.
- These cells are activated on their encounter with foreign agents. These foreign particles act as foreign markers.
The B-cells immediately differentiate into plasma cells which produce antibodies specific to that foreign particle or so-called antigen.
These antibodies attach to the surface of the antigen/foreign agent.
These antibodies detect any antigen in the body and destroy it.
The immunity dependent on B-cells is called humoral immunity.
They originate in the bone marrow and develop in the thymus.
T-cells differentiate into helper cells, cytotoxic cells, and regulatory cells. These cells are released into the bloodstream.
When these cells are triggered by an antigen, helper T-cells release cytokines that act as messengers.
These cytokines initiate the differentiation of B-cells into plasma cells which release antibodies against the antigens.
The cytotoxic T-cells kills the cancer cells.
Regulatory T-cells regulate immune reactions.
- Suppressor T-cells
- Allergies and Autoimmunity
Types of Acquired Immune Response
Humoral immune response.
The antibodies produced by B-lymphocytes are present in the blood cells and they are transported all over the body. This is why it is called the humoral immune response as it consists of an antibody produced by the lymphocytes.
It depends upon the action of antibodies circulating in the body. When an antibody on a B-cell binds with an antigen, humoral immunity comes into play. The antigen is internalized by the B cell and presented on the helper T cell. This activates the B-cell.
The activated B cells grow and produce plasma cells.
These plasma cells release antibodies in the bloodstream. The memory B cells retain the information about the pathogen to prevent any disease caused by that pathogen in the near future.
Cell-mediated Immune Response
Cell-mediated immunity is initiated by the T helper cells.
The cytotoxic T cells eliminate the infected cells from the body by releasing toxins, thereby, promoting apoptosis or programmed cell death.
The T helper cells help to activate other immune cells. Cell-mediated immunity becomes clear in the case of transplant patients.
When any of our sense organs stop functioning, it can be transplanted to replace the malfunctioning organs. But it is not that simple with the immune response. It appears that T-lymphocytes are capable of recognizing whether tissue or an organ is from our body or foreign bodies. This is the reason why we cannot transplant and implant the organs into our body even if we find the donor with the same blood group because our body might reject the transplanted organ. The T-cells quickly recognize that the tissue or an organ as a foreign and do not allow it to become a part of the body. This is why transplant receivers have to take immunosuppressant medication for the rest of their lives. This response is controlled by the T-lymphocytes.
Types of Acquired Immunity
Active immunity.
Active immunity involves the direct response to a foreign antigen within the body. In the case of the acquired or adaptive immune system, the body remembers the pathogens it has encountered in the past. This is a direct result of the active immune system.
Active immunity occurs when we are in contact with the pathogen or its antigen.
Antigens stand for antibody generator. It is with the help of antigens released by the pathogen that our body tackles the pathogen.
So what our body does is, it starts producing antibodies to attack the pathogen based on its antigen. When this happens for the first time, it is called a primary response. Once a body experiences a pathogen for the first time, it keeps a few of the antibodies that attacked the pathogen just in case it attacks for the second time. This is known as natural active immunity.
Passive Immunity
Passive immunity involves the immune response by the antibodies attained from outside the body. The primary response by the body to a pathogen it encounters for the first time is rather feeble, so the first encounter is always a little harsh on the body.
What if we could immunize everyone without the need for them ever getting sick?
Biotechnology has grown tremendously in the last decade or two and now we are capable of manufacturing antibodies for diseases. These ready-made antibodies protect the body even if the body hasn’t yet experienced a primary response.
While active immunity may protect us from a disease for a lifetime, passive immunity is the more short term.
Passive immunity develops immediately and our body could begin its attack on the pathogen right away.
There are two types of passive immunity:
Natural Passive Immunity
Artificial Passive Immunity
AutoImmunity
Sometimes the immune system attacks its own tissues and organs instead of the foreign agents. This is called autoimmunity. Type I diabetes is an example of autoimmune disease.
A vaccine is made up of the antigens of the pathogen that cause the disease. For eg., the smallpox vaccine contains the antigens of the pathogen causing smallpox disease. When a person is vaccinated with the smallpox vaccine the antibody-producing cells are stimulated that produce smallpox antibodies. Thus, the body is protected against the disease occurring in future.
Vaccinating pathogenic microbes into our body deliberately produces a similar response and is termed as artificially acquired immunity.
Immunization is a process providing resistant to pathogenic microbes and other infectious diseases by the administration of a vaccine into the body. By immunization, it stimulates the body’s immune system to protect against subsequent infection or disease.
Also Read: Difference between active and passive immunity
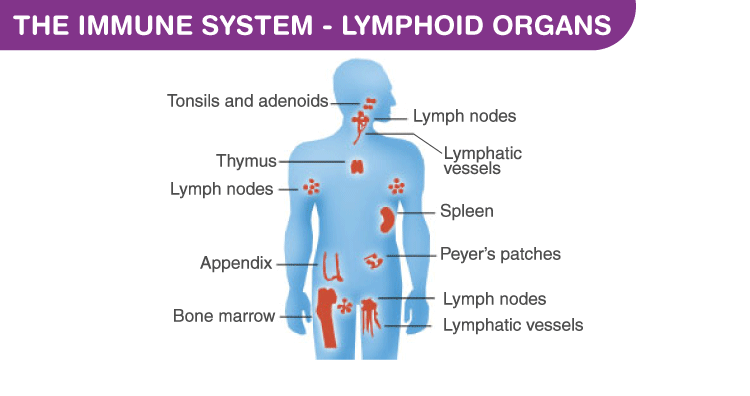
The immune system is our body’s best defensive system. It functions against infringing microorganisms and keeps us healthy.
Immunology is a branch of biology which deals with complex body functions of the immune system. The ability to tackle antigens or pathogens and being healthy is referred to as immunity.
The immune system is composed of cells, tissues, and organs that work unitedly in protecting our body. This system defends the human body from the trespassing pathogens in a variety of ways. They work based on memory, some are innate, and some are acquired. Hence, they function in allergies, autoimmunity and organ transplantation.
The most important cells involved in the immune system are white blood cells (or) leukocytes, which are involved in destroying disease-causing organisms or substances. Apart from the leukocytes, lymphoid organs, tissues, and proteinaceous molecules antibodies are also involved in the defensive system.
Read more: Cytokines: Meaning, Functions and FAQs
Lymphoid Organs
The organs of the immune system which are involved in defending the body against invading pathogens causing infections or spread of tumours is termed as Lymphoid organs. It includes bone marrow, blood vessels, lymph nodes, lymphatic vessels, thymus, spleen, and various other clusters of lymphoid tissue.
Lymphoid organs are the site of origin, maturation, and proliferation of lymphocytes. They exist as primary, secondary or tertiary and these are based on their stage of development and maturation.
These organs consist of fluid connective tissues with different types of leukocytes or white blood cells. The highest percentage of Lymphocytes are present in the white blood cells or leukocytes.
Primary lymphoid organs
The primary lymphoid organs produce and allow the maturation of lymphocytes. It also serves by generating lymphocytes from immature progenitor cells. Therefore it is referred to as the central lymphoid organs. Examples of primary lymphoid organs include thymus and the bone marrow.3
Secondary lymphoid organs
The secondary lymphoid organs are referred to as the peripheral lymphoid organs as they are involved in promoting the sites for the interaction of lymphocytes with the antigen to become effector cells. They initiate an adaptive immune response. The secondary lymphoid organs Examples of secondary lymphoid organs spleen, tonsils, lymph nodes, appendix, etc. are secondary lymphoid organs.
Tertiary lymphoid organs
The tertiary lymphoid organs usually contain very less number of lymphocytes. It plays an important role during the inflammation process.
Also Read: Principles of Prevention
This is about immunity, their types- innate and acquired immunity, B cells, T cells, Humoral and cell-mediated immune response and the immune system.
Learn more in detail about immunity, their functions and other related topics at BYJU’S Biology .
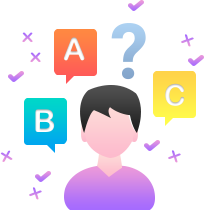
Put your understanding of this concept to test by answering a few MCQs. Click ‘Start Quiz’ to begin!
Select the correct answer and click on the “Finish” button Check your score and answers at the end of the quiz
Visit BYJU’S for all Biology related queries and study materials
Your result is as below
Request OTP on Voice Call
BIOLOGY Related Links | |
Leave a Comment Cancel reply
Your Mobile number and Email id will not be published. Required fields are marked *
Post My Comment

How to download this article
Class 10 selection tes

Register with BYJU'S & Download Free PDFs
Register with byju's & watch live videos.
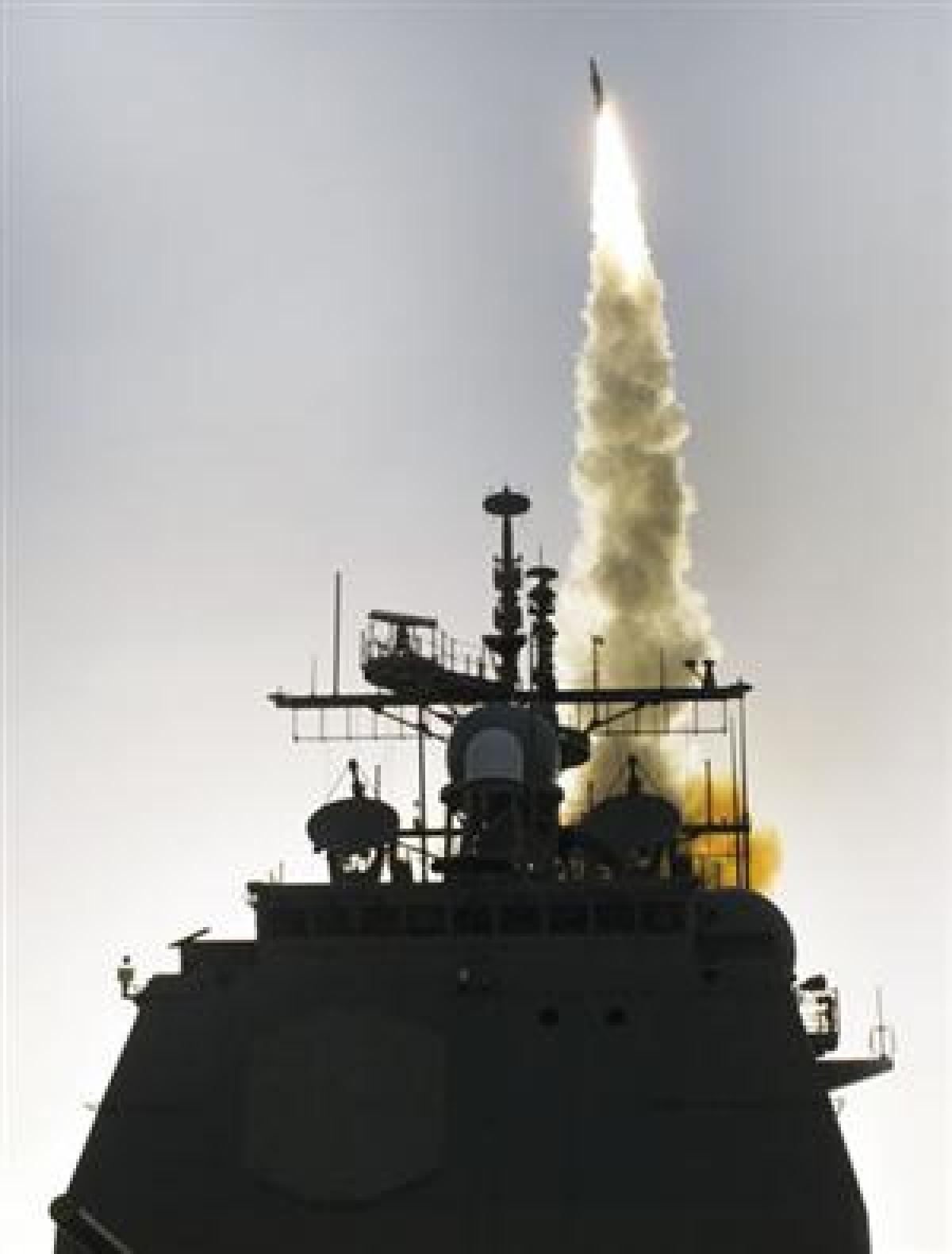
The First Line of Defense
On 17 September 2009 the White House announced a decision to terminate the Bush administration's plan for ten ground-based ballistic-missile interceptors in Poland and a new advanced ballistic-missile defense radar site in the Czech Republic in favor of a "phased, adaptive approach" for missile defense of Europe. 1 This policy change is one of the most significant decisions in international arms control policy since President George W. Bush's decision on 31 December 2001 to withdraw from the Anti-Ballistic Missile Treaty. President Barack Obama's decision has significant implications for U.S. Navy operations and force structure.
Justification for the new policy included an acceleration of ballistic-missile defense (BMD) capability as well as Iran's focus on short- and medium-range ballistic-missile programs rather than the long-range ballistic missiles the Bush-era BMD plan was designed to defeat. International politics play a role in the policy. Russia had strongly objected to establishing any new BMD capability in Europe. While there is no doubt that the ten interceptors envisioned by the Bush administration plan posed no significant military threat to the very large Russian ballistic-missile force, governments and populations in both Eastern Europe and Russia viewed the Bush plan as a statement of support for Eastern Europe and against Russia. Since the Obama administration announcement to cancel the plan for BMD radar sites and interceptors in Eastern Europe, Russia has praised the decision, and the Secretary General of NATO renewed earlier offers to Russia for cooperation in missile defense. 2
The Bush-era European Ballistic Missile Defense proposal was scheduled to be operational by 2015, but Polish and Czech ratification processes were expected to extend that schedule by at least two years. 3 Instead, President Obama approved recommendations by Secretary of Defense Robert Gates and the administration's national security team to accelerate BMD capability for Europe to 2011 by using currently available SM-3 ballistic-missile interceptors deployed on U.S. Navy ships. The sea-based BMD capability is the first phase of the President's plan. The second phase is scheduled to be operational in 2015 and would include upgraded SM-3s at ground sites in Southern and Central Europe. 4
While the September policy announcement focused on BMD for Europe, there is also a significant ballistic-missile threat in the Pacific, primarily from North Korea. There is no reason to believe that threat will diminish in the near term, nor that the BMD needs of Europe will justify disregarding BMD threats in the Pacific. Allies, most notably Japan in the Pacific and possibly other NATO countries in Europe, could contribute to meeting BMD requirements.
Today, the Navy has 19 Aegis BMD combatants. The USS The Sullivans (DDG-68) was the most recent ship to be certified in September 2009. Three are Ticonderoga -class guided-missile cruisers and 16 are Arleigh Burke guided-missile destroyers. The last Ticonderoga was completed in 1994, while the Burke s are still in production. Currently 16 of the 19 ships are assigned to the Pacific and only three to the Atlantic. 5 One of the near-term implications of the new policy will be to either change the homeports of some the Pacific BMD-capable ships to the Atlantic or to greatly increase the number of Atlantic BMD-capable ships through near-term combat system upgrades.
The Missile Defense Agency had planned to upgrade two more CGs by 2010. The Fiscal Year 2010 budget proposal added six BMD upgrades, which would raise the total of BMD-capable Navy combatants to 27. The FY 10 budget proposal also requested 26 more SM-3 missiles for a total of 80 missile interceptors. 6 The unit cost of an SM-3 missile is about $10 million which compares favorably with the approximate unit cost of $70 million for a ground-based interceptor envisioned in the Bush-era plan. Note that an SM-3 inventory of 80 missiles implies an average load of fewer than three SM-3 missiles per ship. Another implication of the new policy is that SM-3 procurement will have to be increased.
How many BMD-capable ships the Navy needs and how to pay for them may be contentious issues. Since the 1980s, the United States has spent more than $150 billion on missile defense. 7 Prior to the recent policy announcement, the FY 10 total budget request for BMD spending was $10.3 billion which included about $1 billion for the Space-Based Infrared System High which provides BMD missile-launch detection. 8 Prior to the policy decision, the Secretary of Defense announced termination of the airborne laser, the multiple-kill vehicle, and the kinetic energy interceptor. 9 The Wall Street Journal quoted a senior administration official who stated that cost concerns were a major factor in the policy decision. 10 Rising U.S. budget deficits suggest that additional funding for accelerated Navy BMD capability will be challenged by those who argue for reducing that deficit. Without additional funding, any increase in Navy BMD capability will have to come at the expense of other programs.
The answer to the question of how many BMD ships the Navy needs should consider alternative BMD-capable ships. The Littoral Combat Ship, with a displacement of about 3,000 tons, does not appear to have the space and displacement necessary to support Aegis radar as well as SM-3 launchers. 11 The Missile Defense Agency funded a semi-submersible sea-based X-Band radar platform, which displaces 50,000 tons. A BMD alternative to consider might be adding SM-3 launch capability to that very large platform. Other large-hull designs such as San Antonio-class amphibious transport docks with 25,000-ton displacement could also be considered.
Balance and Trade-Offs
If sea-based BMD is a long-term requirement, designing a platform to focus on this mission without adding additional warfighting capabilities and minimizing operating and life-cycle costs could be considered. It is difficult to say how this might be funded. The Missile Defense Agency might pay for unique missile-defense design costs and most of the combat system hardware costs, but the Navy would likely be required to pay for hull, personnel, and operating costs. International cooperation is an alternative to share the development costs and reduce unit procurement costs with larger-quantity orders. The Missile Defense Agency has worked closely with Japan since 1999, has memoranda of understanding with the United Kingdom and Australia, and has exchanged information with The Netherlands, Germany, and South Korea.
If the much-delayed Space-Based Infrared System and X-Band radars, combined with near real-time command and control data links, could be used for not only launch detection, but also interceptor guidance, perhaps smaller ships such as the LCS could be considered as BMD launch platforms. However, that is not a near-term option for sea-based BMD, which currently requires upgraded Aegis radar.
Regardless of the sea-based BMD force structure it is fair to assume that the per-unit cost will be significantly higher than the same alternative based on land. This appears to be reflected in Phase 2 of the administration's plan. If land-based systems are preferred over sea-based capability because of lower life-cycle cost, the Navy should minimize its investment in sea-based BMD capability to what is needed until that capability is diminished by land-based systems.
Operational Challenges
In addition to the cost to increase the Navy's capability, the policy decision is also likely to have a significant impact on operations. One operational alternative would be to surge BMD-capable ships to the Eastern Mediterranean during periods of increased tension. That would not meet the 24/7 BMD capability against up to five incoming ballistic missiles envisioned in the Bush-era plan. As long as Iran continues to reject international arms-control inspections, conducts an aggressive nuclear fuel enrichment program including covert facilities, and develops a variety of ballistic missiles, it is likely that a permanent Navy BMD presence will be required to defend Europe. 12
Some have suggested that one reason for the new policy was the proven success of sea-based ballistic-missile interceptors. In February 2008 the Navy successfully used a sea-based interceptor to destroy a decaying orbit satellite in the Pacific. While this impressive accomplishment demonstrated the ability of a sea-based interceptor to hit a target 250 kilometers above the earth, in this case the target trajectory was known months in advance, and the firing ship could be positioned for optimal engagement. That is certainly not the case in most likely real-world ballistic-missile threat scenarios. The uncertainty of their targets and launch points greatly complicates the fire-control challenges of mid-course ballistic-missile intercept.
Another operational challenge for the ships assigned to BMD missions is the very short reaction time required after launch. Even if a ship is optimally positioned for intercept, the time to detect, track, and launch an SM-3 interceptor capable of successfully engaging the target is minutes. If the ship is off the missile's flight path, that time is reduced. Even with the best command and control communications, it is likely that interceptor launch decisions will have to be governed by rules of engagement. There will not be sufficient time for decision makers off the ship to review and approve specific interceptor launch decisions. There is also a political dimension to the intercept decision because the ballistic missile being engaged is very likely to have a weapon of mass destruction (WMD) warhead. The fallout pattern of the warhead could include populated land masses of neutral or friendly states.
An additional operational impact of the policy decision is on the combat readiness of the ships that execute this mission. Navy BMD-capable combatants are multimission warships. When assigned a 24/7 BMD mission, their ability to maneuver over large areas will likely be restricted. It is also likely that the effectiveness of the ship's other combat capabilities will tend to degrade. While that may not have a direct impact on the ship's ability to execute the BMD mission, the deterioration certainly has a negative impact on other missions to which she may be assigned. There is also a possible adverse impact on crew morale caused by the boredom of continuous defensive patrols. Good leadership can overcome many of these challenges, but they must be addressed and carefully considered in operational planning. If the Navy assigns a large number of ships to the BMD mission, they will not be available for combating piracy off East Africa, supporting drug interdiction off South America, or any of the other important missions these combatants have traditionally supported.
A Strategic Mission
The ballistic-missile defense mission could be viewed as a strategic mission similar to the submarine-launched ballistic-missile program the Navy has successfully executed since the USS George Washington (SSBN-598) was launched 50 years ago. In this case, the Navy decided to maximize the operational availability of these strategic assets by assigning two crews to each. This could be considered for BMD ships as well. Dual crews for large combatants, however, significantly increase personnel costs, including not just salaries, but recruiting, training, equipping, health care, housing, and retirement costs.
Another alternative to increase operational availability would be to use crew swaps to eliminate transit times to and from BMD-patrol stations. Crew-swap alternatives have been used to increase ship operational availability, but implementation for platforms not nearing decommissioning poses challenges that also require good leadership to overcome. The crew-swap alternative does not solve the problem of completing ship maintenance requirements beyond the ship's force at-sea capability.
It is every Sailors' duty to execute the mission they are given to the best of their ability, and there is no doubt that the Navy will do exactly that in the BMD mission or any other operational assignment. It is important to remember, however, that BMD is part of a larger security concern. The threats to United States and allied security today have greatly changed since the Cold War. In some ways it was much easier to plan military operations then. We knew who the adversaries were expected to be and understood much about their weapons and tactics. Today, security threats span a wide range of potential adversaries, some of which are not even states and include diverse weapons and tactics to constitute irregular warfare.
We must carefully consider where the threat from ballistic missiles fits in national defense planning and priorities. As Secretary Gates recently said, some proponents of placing a very high priority on BMD programs take an almost theological view. To do anything less than maximum effort regardless of cost in the name of BMD is a breach of faith. 13 Discretionary defense spending has always been limited and is likely to become more so as personnel costs rise and national budget deficits increase. It is very important that the Navy, the nation, and our allies not make the threat from ballistic missiles greater than it actually is.
Terrorists, WMDs, and Ballistic Missiles
To illustrate this point about the ballistic-missile threat, put yourself in the shoes of a rogue state leader who has ballistic-missile and WMD capability. It makes little sense to use the missile without a WMD warhead. The WMDless missiles could be used to terrorize civilian populations, as Hezbollah recently did in Israel with many short-range missile attacks. But such weapons do not have the capability to have a major battlefield impact. A WMD-armed ballistic missile can have a very large impact, but its use needs to be very carefully considered by those who might employ it. While intercept of a ballistic missile is very challenging, it is easy to determine its launch point. Unless the missile is launched from a submarine in international waters—a capability rogue states and terrorist organizations do not currently have—massive retaliation against a ballistic-missile WMD attack should be expected.
Therefore, given that a rogue state or terrorist organization has a WMD capability and the will to use it, why would a ballistic missile with an obvious fingerprint of its origin be used to deliver the attack and incur the very high risk of massive retaliation? It makes more sense to deliver the WMD by means that leave some doubt about who was responsible for the attack, thereby complicating the retaliation decision-making process. It is much easier and far less expensive to deliver a WMD by container, truck, or other covert means than by a ballistic-missile system. The warhead's delivery is also greatly simplified in over-land/sea covert delivery as compared to terminal target guidance and warhead fuzing at Mach 7 or higher speed.
When considering BMD or any other military mission, it is important to understand the WMD concept of operations across the military capabilities of friendly and hostile forces and the environment in which they may be used. The airborne laser is a negative example of this point. While the technology of using a very large aircraft to employ a line-of-sight weapon against a ballistic missile is interesting, what is the survivability of that non-stealthy airborne platform in a period of hostility commenced at the adversary's choice of time? How many targets can an airborne platform engage before running out of stored or generated electrical power?
If all you have is a hammer, every problem looks like a nail. The implication of this for BMD draws from antisubmarine warfare. The best way to defend against enemy submarines is destroy them before they leave port. In the case of BMD, the best defense against ballistic missiles is to destroy the missiles before they are launched. This has the added advantage of leaving the effects of their warheads at the point of origin, not strewn over neutral or friendly countries. The Navy's rail gun, with a theoretical range of 400 miles, a very short flight time, and sufficient accuracy to destroy a ballistic missile with a single round, has great potential in BMD warfare.
The advantages of naval power, including persistence, access, and mobility combined with proven SM-3 intercept capability, offer near-term ballistic-missile defense options important to national and international security. The Navy must balance BMD with its other missions that carry many responsibilities in providing maritime security. While some Navy capabilities such as strike warfare are also supported by other services, only the Navy can execute certain missions, such as antisubmarine warfare.
1. White House Press Release, "Fact Sheet on U.S. Missile Defense Policy, A Phased Adaptive Approach for Missile Defense in Europe," 17 September 2009.
2. Stephen Castle, "NATO Proposes Link With Russia's Missile Defense," The New York Times, 18 September 2009.
3. Robert M. Gates, "A Better Missile Defense for a Safer Europe," The New York Times, 19 September 2009.
5. MDA, "Aegis Ballistic Missile Defense," Missile Defense Agency Fact Sheet, 18 August 2009.
6. Jenny Shin, Overview of the Fiscal Year 2010 Ballistic Missile Defense Budget Request, Center for Defense Information, 20 May 2009.
7. Fareed Zakaria, "US did the right thing the wrong way," CNN, 11 September 2009.
8. Shin, Overview.
9. Gates, "Better Missile Defense."
10. Jonathan Weisman and Peter Spiegel, "Cost Concerns Propelled U.S. Missile Policy," The Wall Street Journal, p. A5, 19-20 September 2009.
11. Milan Vego, "No Need for High Speed," U.S. Naval Institute Proceedings, September 2009. Vego describes the LCS mission payload limit as about 180 tons.
12. William Branin, Walter Pincus, and Karen DeYoung, "Iran Test Fires Most Advanced Missiles," The Washington Post, 28 September 2009.
13. Gates, "Better Missile Defense."
View the discussion thread.
Receive the Newsletter
Sign up to get updates about new releases and event invitations.
You've read 1 out of 5 free articles of Proceedings this month.
Non-members can read five free Proceedings articles per month. Join now and never hit a limit.
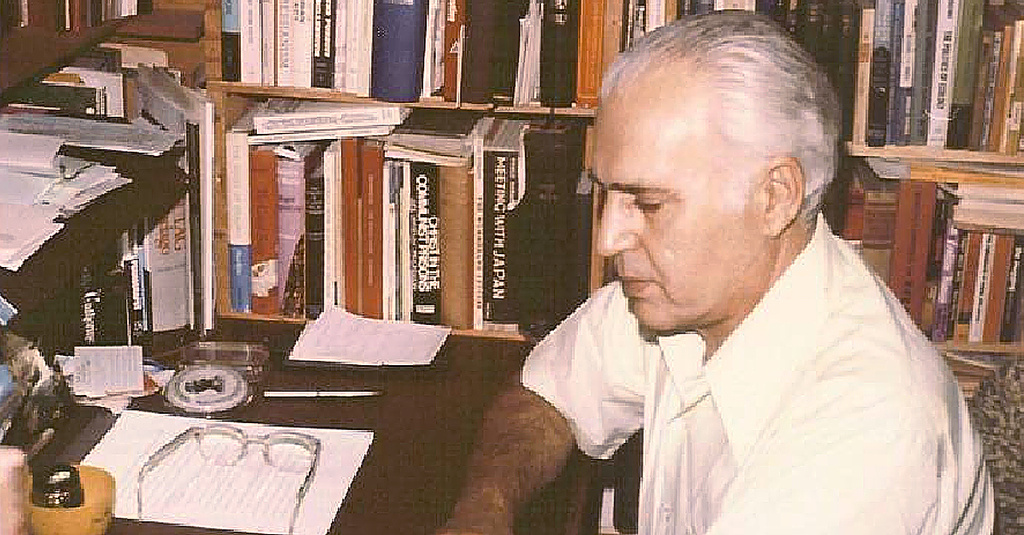
First Line of Defense
Impotent men give impotent answers. Leave them alone and pass them by. God’s regenerating power and His law give man power, estate, and calling. To be a redeemed man and to have God’s law is to have the plan of conquest and dominion and the power to execute it.
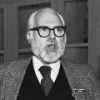
- R. J. Rushdoony
Chalcedon Report No. 121, September 1975
Communism does not need to defend itself militarily in the same way as do other forms of politics, because it is usually on both sides of every border. It is on the march in enemy territory as a militant faith. Its real strength is its religious appeal. However, as a false religion, unable to deliver on its promises, its defeat begins wherever it is victorious, in that a disillusioned people then must be kept in suppression by force. It is thus destined to become one of the biggest failures of the twentieth century.
Wherever a people rely on the military as their first line of defense, they are lost. Military strength is a necessity, but a reliance on it for security is a disaster. If men rely on the sword for their defense, our Lord made clear, they shall perish by the sword (Matt. 26:52), because “man shall not live by bread alone, but by every word that proceedeth out of the mouth of God” (Matt. 4:4).
The first line of defense is a true and living faith. In the nineteenth century, when the United States had little military power except in wartime, U.S. power moved men all over the world, and America was the dream and ideal of millions. In those years, the U.S. peacetime army numbered from 200 to a maximum of 20,000 shortly before World War I, yet its influence made European and Asian autocracies afraid because of the “subversive” infiltration of American beliefs and practices. In every situation of need, American aid, not from the federal government but from the people, was a decisive factor in every area of the world. As against defense by military power, the American strength then was a strong offensive by means of a sense of Christian mission. Earlier, Christian Europe had commanded the world with that sense of mission and power, then America.
A primary reliance on military (or police) defense is the last resort of impotent men. Where men’s minds and passions see force as the essential answer, it means that faith, while professed, is lost. The cry of, “kill the (black, white, yellow, Communist, Fascist, or what-have-you) bastards,” is the mark of impotent men, with no sense of mission and no faith to command themselves or others.
When, a century ago, Sir Samuel Baker took his beautiful and protected bride into the heart of Africa to search for the sources of the Nile, his companions were all pagan and murderous Arabs and blacks who determined to rob and kill the Bakers at the first opportunity. They never did. Baker’s sublime sense of mission and command held them in awe. At the least sign of trouble, he lectured them like an earnest Sunday school teacher putting a disorderly class in its place. His aura of power was enough to command them.
Western man now has instead an aura of fear and of greed. He thinks of himself only, and his only mission is self-security. He wants to be left alone, to have privacy, his pleasures, and his own way. He cannot command himself, let alone a world. He can be in the majority in a country and still lose. Before he acts to defend anything, he asks himself, “Will they come after me if I lose?” When this is true, a man is already dead within, and already a prisoner.
The Puritans, as against the usurping power of the king, Charles I, made their standard, “The crown rights of King Jesus.” The Puritans at their maximum strength were 4 percent of England, but the crown rights of the monarchy fell before them. When they began to think more of the rights of their church and their interests than of Christ the King, the Puritans also failed.
The key, thus, is return to a sense of Christian mission and to a faithful application of God’s law to every area of life. St. Patrick’s greatness was that, in an age when the enemy was overrunning the land, St. Patrick overran the enemy. He set out to convert his enslavers and enemies, and he made of pagan Ireland one of the greatest Christian cultures the world has known and the great missionary force on the continent. More able men than St. Patrick failed because they hated and bewailed the savage enemy. St. Patrick converted and commanded them.
Remember, too, before you call yourself a Christian that God has no impotent sons. He has suffering and sometimes martyred sons, but never impotent, and ultimately always victorious sons.
There are hundreds of millions of peoples in Communist countries who hate Marxists and wish them dead: such people, impotent and self-destructive in their hatred, are easily cowed and controlled. The underground church is a far greater problem: it is busy trying to convert its oppressors, and often succeeding. The Communists realize that they have little to fear from hatred: it is too deeply grounded in fear to be other than impotent. It is Christian faith which is for them the menace. “Holy fools” are aggressive and confident, and everywhere at work.
Well, where do you stand in all of this? Have you made it your mission to fear and to hate? (We may hear from you then, an angry, hateful, and, of course, anonymous note!) Or is it your estate and calling to believe and obey the Lord, and to exercise dominion in His name?
Rev. R.J. Rushdoony (1916–2001), was a leading theologian, church/state expert, and author of numerous works on the application of Biblical law to society. He started the Chalcedon Foundation in 1965. His Institutes of Biblical Law (1973) began the contemporary theonomy movement which posits the validity of Biblical law as God’s standard of obedience for all. He therefore saw God’s law as the basis of the modern Christian response to the cultural decline, one he attributed to the church’s false view of God’s law being opposed to His grace. This broad Christian response he described as “Christian Reconstruction.” He is credited with igniting the modern Christian school and homeschooling movements in the mid to late 20th century. He also traveled extensively lecturing and serving as an expert witness in numerous court cases regarding religious liberty. Many ministry and educational efforts that continue today, took their philosophical and Biblical roots from his lectures and books.
- Search Menu
Sign in through your institution
- Advance Articles
- Author Guidelines
- Submission Site
- Open Access Policy
- Self-Archiving Policy
- Why Submit?
- About Political Science Quarterly
- About the Academy of Political Science
- Editorial Board
- Advertising & Corporate Services
- Journals on Oxford Academic
- Books on Oxford Academic

- < Previous
First Line of Defense: The U.S. Navy Since 1945, by Paul B. Ryan
- Article contents
- Figures & tables
- Supplementary Data
Dov S. Zakheim, First Line of Defense: The U.S. Navy Since 1945, by Paul B. Ryan , Political Science Quarterly , Volume 96, Issue 3, Fall 1981, Pages 499–500, https://doi.org/10.2307/2150571
- Permissions Icon Permissions
Article PDF first page preview
The academy of political science members, personal account.
- Sign in with email/username & password
- Get email alerts
- Save searches
- Purchase content
- Activate your purchase/trial code
- Add your ORCID iD
Institutional access
Sign in with a library card.
- Sign in with username/password
- Recommend to your librarian
- Institutional account management
- Get help with access
Access to content on Oxford Academic is often provided through institutional subscriptions and purchases. If you are a member of an institution with an active account, you may be able to access content in one of the following ways:
IP based access
Typically, access is provided across an institutional network to a range of IP addresses. This authentication occurs automatically, and it is not possible to sign out of an IP authenticated account.
Choose this option to get remote access when outside your institution. Shibboleth/Open Athens technology is used to provide single sign-on between your institution’s website and Oxford Academic.
- Click Sign in through your institution.
- Select your institution from the list provided, which will take you to your institution's website to sign in.
- When on the institution site, please use the credentials provided by your institution. Do not use an Oxford Academic personal account.
- Following successful sign in, you will be returned to Oxford Academic.
If your institution is not listed or you cannot sign in to your institution’s website, please contact your librarian or administrator.
Enter your library card number to sign in. If you cannot sign in, please contact your librarian.
Society Members
Society member access to a journal is achieved in one of the following ways:
Sign in through society site
Many societies offer single sign-on between the society website and Oxford Academic. If you see ‘Sign in through society site’ in the sign in pane within a journal:
- Click Sign in through society site.
- When on the society site, please use the credentials provided by that society. Do not use an Oxford Academic personal account.
If you do not have a society account or have forgotten your username or password, please contact your society.
Sign in using a personal account
Some societies use Oxford Academic personal accounts to provide access to their members. See below.
A personal account can be used to get email alerts, save searches, purchase content, and activate subscriptions.
Some societies use Oxford Academic personal accounts to provide access to their members.
Viewing your signed in accounts
Click the account icon in the top right to:
- View your signed in personal account and access account management features.
- View the institutional accounts that are providing access.
Signed in but can't access content
Oxford Academic is home to a wide variety of products. The institutional subscription may not cover the content that you are trying to access. If you believe you should have access to that content, please contact your librarian.
For librarians and administrators, your personal account also provides access to institutional account management. Here you will find options to view and activate subscriptions, manage institutional settings and access options, access usage statistics, and more.
Short-term Access
To purchase short-term access, please sign in to your personal account above.
Don't already have a personal account? Register
Month: | Total Views: |
---|---|
December 2023 | 1 |
March 2024 | 1 |
Email alerts
Citing articles via.
- Recommend to Your Librarian
- Advertising & Corporate Services
- Journals Career Network
Affiliations
- Online ISSN 1538-165X
- Print ISSN 0032-3195
- Copyright © 2024 The Academy of Political Science
- About Oxford Academic
- Publish journals with us
- University press partners
- What we publish
- New features
- Open access
- Rights and permissions
- Accessibility
- Advertising
- Media enquiries
- Oxford University Press
- Oxford Languages
- University of Oxford
Oxford University Press is a department of the University of Oxford. It furthers the University's objective of excellence in research, scholarship, and education by publishing worldwide
- Copyright © 2024 Oxford University Press
- Cookie settings
- Cookie policy
- Privacy policy
- Legal notice
This Feature Is Available To Subscribers Only
Sign In or Create an Account
This PDF is available to Subscribers Only
For full access to this pdf, sign in to an existing account, or purchase an annual subscription.
Ask the publishers to restore access to 500,000+ books.
Internet Archive Audio
- This Just In
- Grateful Dead
- Old Time Radio
- 78 RPMs and Cylinder Recordings
- Audio Books & Poetry
- Computers, Technology and Science
- Music, Arts & Culture
- News & Public Affairs
- Spirituality & Religion
- Radio News Archive
- Flickr Commons
- Occupy Wall Street Flickr
- NASA Images
- Solar System Collection
- Ames Research Center
- All Software
- Old School Emulation
- MS-DOS Games
- Historical Software
- Classic PC Games
- Software Library
- Kodi Archive and Support File
- Vintage Software
- CD-ROM Software
- CD-ROM Software Library
- Software Sites
- Tucows Software Library
- Shareware CD-ROMs
- Software Capsules Compilation
- CD-ROM Images
- ZX Spectrum
- DOOM Level CD

- Smithsonian Libraries
- FEDLINK (US)
- Lincoln Collection
- American Libraries
- Canadian Libraries
- Universal Library
- Project Gutenberg
- Children's Library
- Biodiversity Heritage Library
- Books by Language
- Additional Collections
- Prelinger Archives
- Democracy Now!
- Occupy Wall Street
- TV NSA Clip Library
- Animation & Cartoons
- Arts & Music
- Computers & Technology
- Cultural & Academic Films
- Ephemeral Films
- Sports Videos
- Videogame Videos
- Youth Media
Search the history of over 866 billion web pages on the Internet.
Mobile Apps
- Wayback Machine (iOS)
- Wayback Machine (Android)
Browser Extensions
Archive-it subscription.
- Explore the Collections
- Build Collections
Save Page Now
Capture a web page as it appears now for use as a trusted citation in the future.
Please enter a valid web address
- Donate Donate icon An illustration of a heart shape
First line of defense, 1755-56 : beginnings of the frontier forts / by William A. Hunter.
Bookreader item preview, share or embed this item, flag this item for.
- Graphic Violence
- Explicit Sexual Content
- Hate Speech
- Misinformation/Disinformation
- Marketing/Phishing/Advertising
- Misleading/Inaccurate/Missing Metadata
plus-circle Add Review comment Reviews
2 Favorites
DOWNLOAD OPTIONS
For users with print-disabilities
IN COLLECTIONS
Uploaded by Unknown on January 23, 2017
SIMILAR ITEMS (based on metadata)
- Police and Court Reports
- Neighborhood News
- National News
- International News
- Local Sports
- Ohio Sports
- National Sports
- Local Columns
Letters to the Editor
- Engagements
- Anniversaries
- Births/Birthdays
- Classifieds
- Garage Sales
- Terms of Service
- Submit News
- Browse notices
- Place a notice

- Today's Paper
Subscribe Today
Parents are first line of defense.
To the editor:
In my years serving Wellsville, I’ve seen firsthand that strong families are the foundation of our town. As a former councilman and school board member, I understand parents’ unique challenges in raising children in today’s digital age. While the online world offers immense opportunities, it also presents significant risks that parents must navigate to protect their children.
Across Ohio, parents are the first line of defense for their children’s safety, teaching right from wrong and guiding them toward success. The same standard should apply online, but the tools to protect children on social media are often inadequate or confusing, leaving parents powerless against the content that their teens consume on social media. The internet can be dangerous, especially for teenagers, who are vulnerable to inappropriate content, cyberbullying, and other online risks.
Family values, responsibility, and the care we provide for our children are at the heart of our community. Ohio has rightly acknowledged the gap in online safety for our teens, making strides with the Social Media Parental Notification Act, which mandates parental consent before minors can create social media accounts. However, to truly uphold the family values that are the bedrock of our society, we must simplify the process to put parents in control. Parents should not be left navigating a patchwork of confusing rules for different platforms that vary from state to state.
Parents must push for federal legislation requiring app stores to obtain parental consent before allowing teens under 16 to download apps. A parental consent requirement at the app-store level would streamline the process, allowing parents to monitor teens’ digital interactions without managing each account individually. By restoring parents’ control, we can provide a consistent, nationwide standard that protects every child in America, no matter where they live.
Leaders like Senator JD Vance and Representative Jim Jordan, who have tirelessly advocated for protecting family values, should champion a cause that safeguards our teens online. A consistent, nationwide policy can guarantee that the authority to decide what’s best for children remains firmly in the hands of parents rather than being dictated by tech corporations or government agencies. While the digital age has brought remarkable advancements, it has also introduced significant challenges for parents. We must empower parents to defend these values in the digital age. By enacting federal legislation that requires parental consent for app-store downloads, we can take a critical step toward securing a safer online environment for our teens. Now is the time for Congress to act and for all of us to work together to protect the future of our families.
JOHN MORROW,
Liverpool Township
How about drilling for a solution?
To the editor: As a lifelong resident of the Salem area, I have been concerned and upset with our city’s policy ...
Ohioans cheated, deceived by elected leaders
To the editor: A new record: twice in one week Ohioans were both cheated and deceived by their elected ...
To the editor: In my years serving Wellsville, I’ve seen firsthand that strong families are the foundation of ...
Mayor offers thanks for July 4th support
To the editor: As Mayor of the City of Salem, I want to thank the following citizens and organizations for their ...
Faith in our judicial system
To the editor: We must have faith in our judicial system. I shed tears following the results of the Trump trial. ...
Starting at $2.99/week.
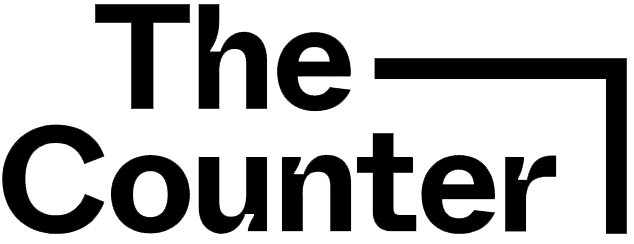
An unlikely line of defense during heat waves: Food banks
Food pantries and meals-on-wheels organizations are taking on a new role during climate emergencies..
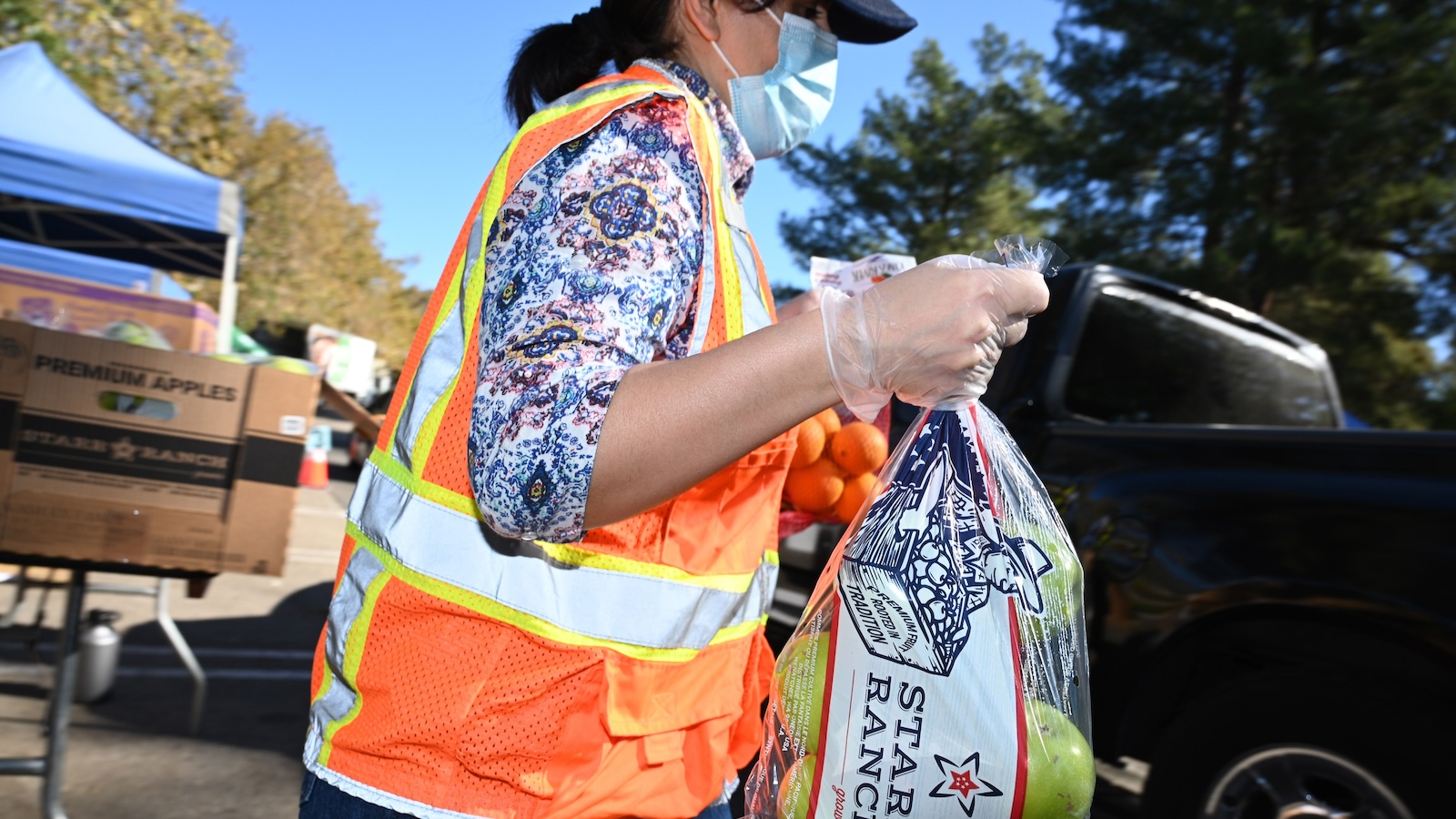
On a Tuesday morning in mid-August, Mary Hynes was blasting her air conditioning. Hynes has walking problems, and she said she’s passed out “a couple of times” in the past. The issue went away after having her pacemaker adjusted, but it was enough to make her nervous about leaving her apartment in the Chelsea neighborhood of Manhattan. And then New York experienced a particularly hot summer with multiple heat waves , making her even less likely to go outside.
“Thank God for these guys,” she said, referring to the volunteer dropping off her weekly meals. Hynes is one of more than 20,000 New Yorkers 60 and older who receive meal deliveries from Citymeals on Wheels, a nonprofit organization founded in 1981 to fill the gaps in the city’s public meal assistance program for older, homebound New Yorkers. Her experience is an example of how, during heat emergencies, food assistance can provide a lifeline to vulnerable communities. “I didn’t think they were going to do deliveries because of the heat” at one point this summer, she said, “and I would’ve understood.”
For older adults living with disabilities or mobility issues, heat can compound the challenges of getting outside, getting groceries, and cooking for oneself. By delivering meals during bouts of extreme weather, including heat waves, food distribution organizations like Citymeals serve as a line of defense against the worst of climate impacts.
“Emergencies have become a sweet spot for us,” said Beth Shapiro, the CEO of Citymeals.
Grist thanks its sponsors. Become one .
To support our nonprofit environmental journalism, please consider disabling your ad-blocker to allow ads on Grist. Here's How
New York City’s Department for the Aging facilitates home-delivered meals for older New Yorkers who aren’t able to prepare their own meals, but only on weekdays. “The city funds meals Monday through Friday, and our founder believed that people should eat every day of the week, as we still do,” said Shapiro. In addition to weekend and holiday service, Citymeals, which partners with 30 senior centers in all five boroughs of New York City, also delivers food to older adults during localized emergencies. To qualify for Citymeals, recipients must be 60 or older and have a physical or mental health condition that prevents them from shopping for food or cooking regularly for themselves.
Citymeals volunteers and staff have delivered meals during major disasters — for example, 2012’s Hurricane Sandy, which flooded lower Manhattan, damaged buildings, and left hundreds of thousands without power. But extreme heat acts on vulnerable populations in ways that are more subtle, but just as dangerous. For adults 65 and older, living with certain illnesses, or taking certain medications, may impact the body’s ability to tolerate increases in temperature . Older adults with cardiovascular problems and other illnesses that can cause weakness are more susceptible to heat-related illness . Additionally, the social isolation that many older adults experience can become life-threatening during a heat wave . Home meal deliveries are one way to check in on older folks during heat emergencies — and as climate change makes summers hotter and hotter, the need for such high-touch services is growing.
“One of the things we talk about during heat events is checking on your neighbor,” said Heidi Brown, professor and program director of epidemiology at the University of Arizona’s college of public health. Volunteers bringing food to homebound people can help to break up the social isolation that they face. For example, volunteers can observe how a meal recipient appears on a hot day, check for signs of heat-related illness, and ask if they have enough water or any trouble cooling their home. (Citymeals instructs volunteers who observe serious problems to contact the meal recipient’s case manager.) Checking in may not be the explicit “mandate” of meal on wheels organizations, said Brown, “but that is a piece of what they’re doing. They’re bringing resources, making sure that that person is OK. And if not, reaching out and getting them to medical resources, getting them to a cooling station, or making sure they know where cooling stations are.”
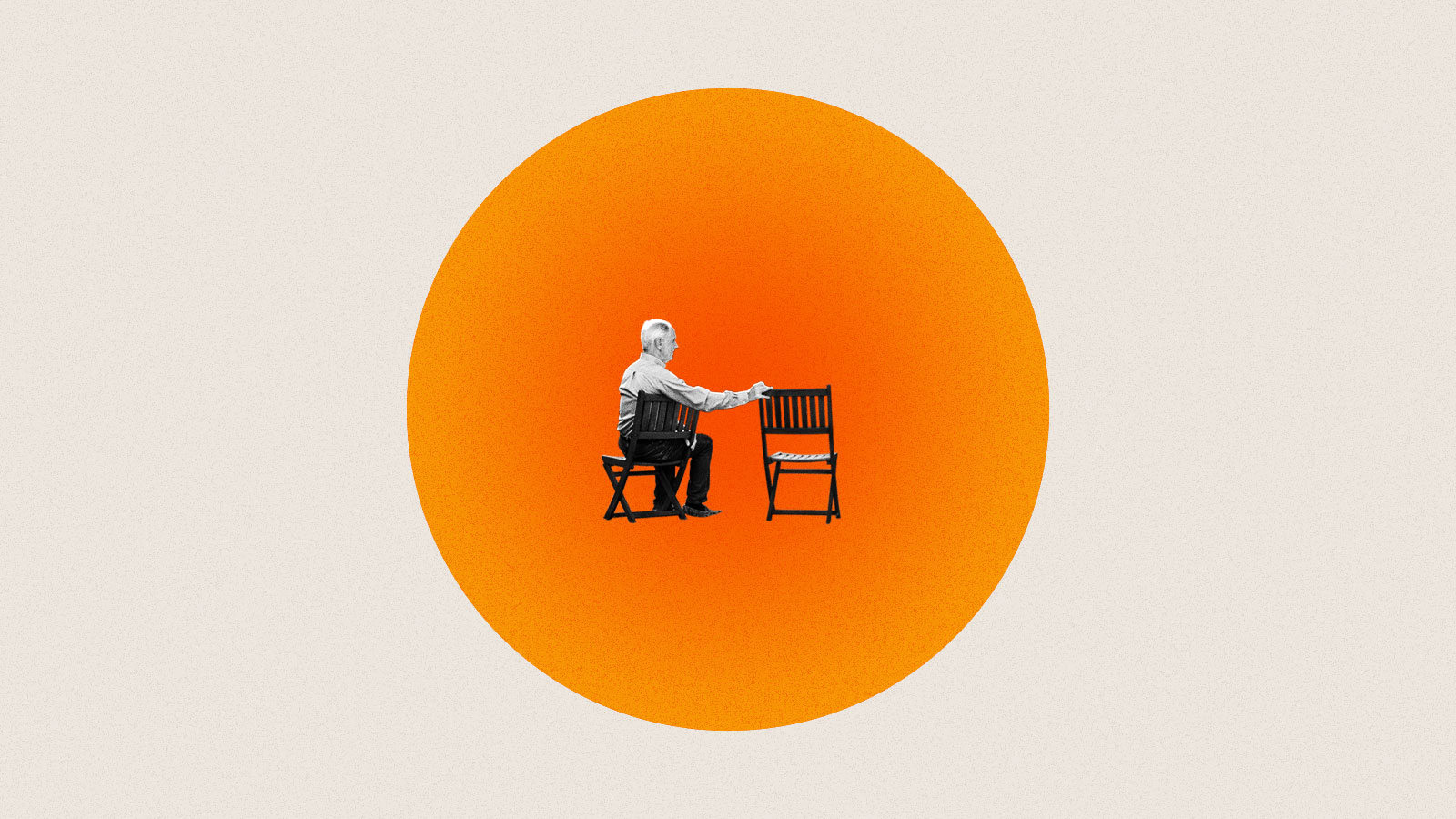
The volunteer serving Hynes’ building said one thing he might pay attention to is whether a meal recipient has AC running or not. Even during a heat wave, it isn’t a given: Citymeals recently partnered with the City University of New York’s Urban Food Policy Institute to survey recipients of meal assistance services for older adults , including Citymeals. Almost half of the respondents reported experiencing food insecurity in the last 12 months, and over 60 percent were living on less than $15,000 a year.
“If I’m living on that, am I able to buy food, pay for medicine, turn on an air conditioner if I have it?” said Shapiro.
In addition to exacerbating financial burdens, extreme heat can complicate mobility issues for older people facing hunger. “Which makes sense,” said Michael Flood, the president and CEO of the Los Angeles Regional Food Bank. “None of us want to go out when it’s 98 degrees.” Across California, the heat in July shattered records and became the state’s hottest month on record . For Los Angeles, the most brutal heat of the summer is yet to come: This week, Southern California is expecting its longest and hottest heat wave of the summer .
The COVID-19 pandemic made the Southern California food bank more aware of how public health crises impact older adults’ mobility and ability to access food distribution hubs. Now, the organization delivers groceries to low-income people 60 or older .
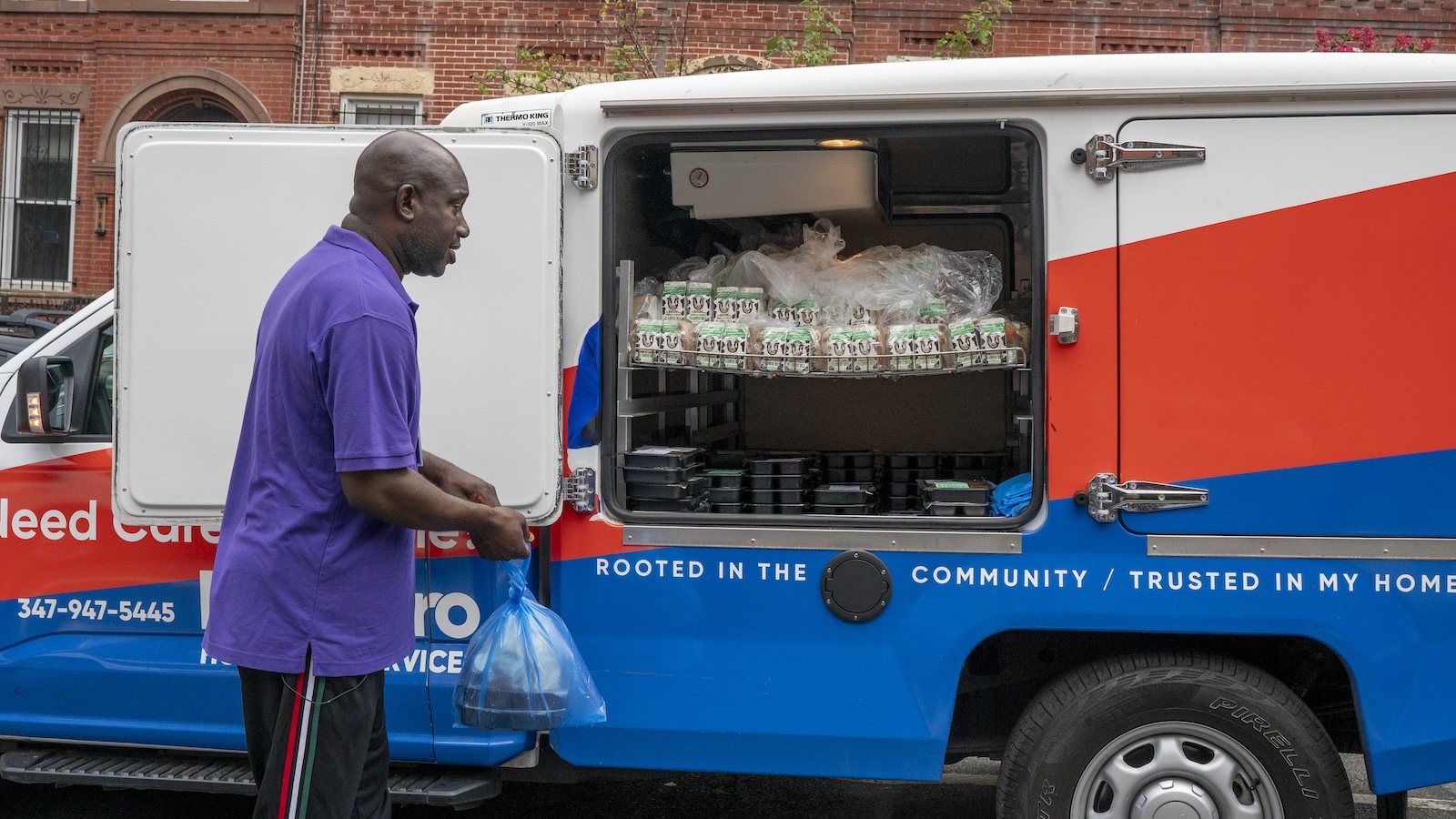
Older adults aren’t the only population who experience heightened need for food banks and meal delivery services during extreme weather events. Unhoused people and families with children may also come to rely on food assistance programs more when there is a heat wave.
The Silver Lake Community Church in L.A. has a food pantry program that was designed with the needs of homeless folks in mind. When it’s really hot outside, unhoused people may decide not to come to the church for food assistance, said office administrator Stephanie Young. But volunteers will also pick up folks from wherever they’re staying and bring them to the church for programming, whether that’s the food pantry or the church’s shower program.
Homeless individuals who attend a free breakfast program at the St. Francis Center in downtown L.A. might stay and chat with other community members on particularly hot days, enjoying the center’s AC, according to programs director Cesar Argueta.
The summer also presents a twofold challenge for food-insecure families, especially those with children. “We see hunger spike a lot in the summer,” said Celia Cole, the CEO of Feeding Texas, a nonprofit dedicated to fighting hunger in the country’s second-largest state by population, “because what happens is those kids get out of school where they would have access to free breakfast and free lunch.” During the COVID-19 pandemic, Congress granted waivers that expanded schools’ and community groups’ ability to offer free meals to young people during the summer months, but those waivers expired in 2022. There are currently no sites in Texas offering free meals and snacks to children via the U.S. Agriculture Department’s Summer Food Service Program , according to the latest data listed on the program’s website.
“At the same time,” Cole added, “people’s utility bills are soaring,” which reduces the income they have available for groceries.
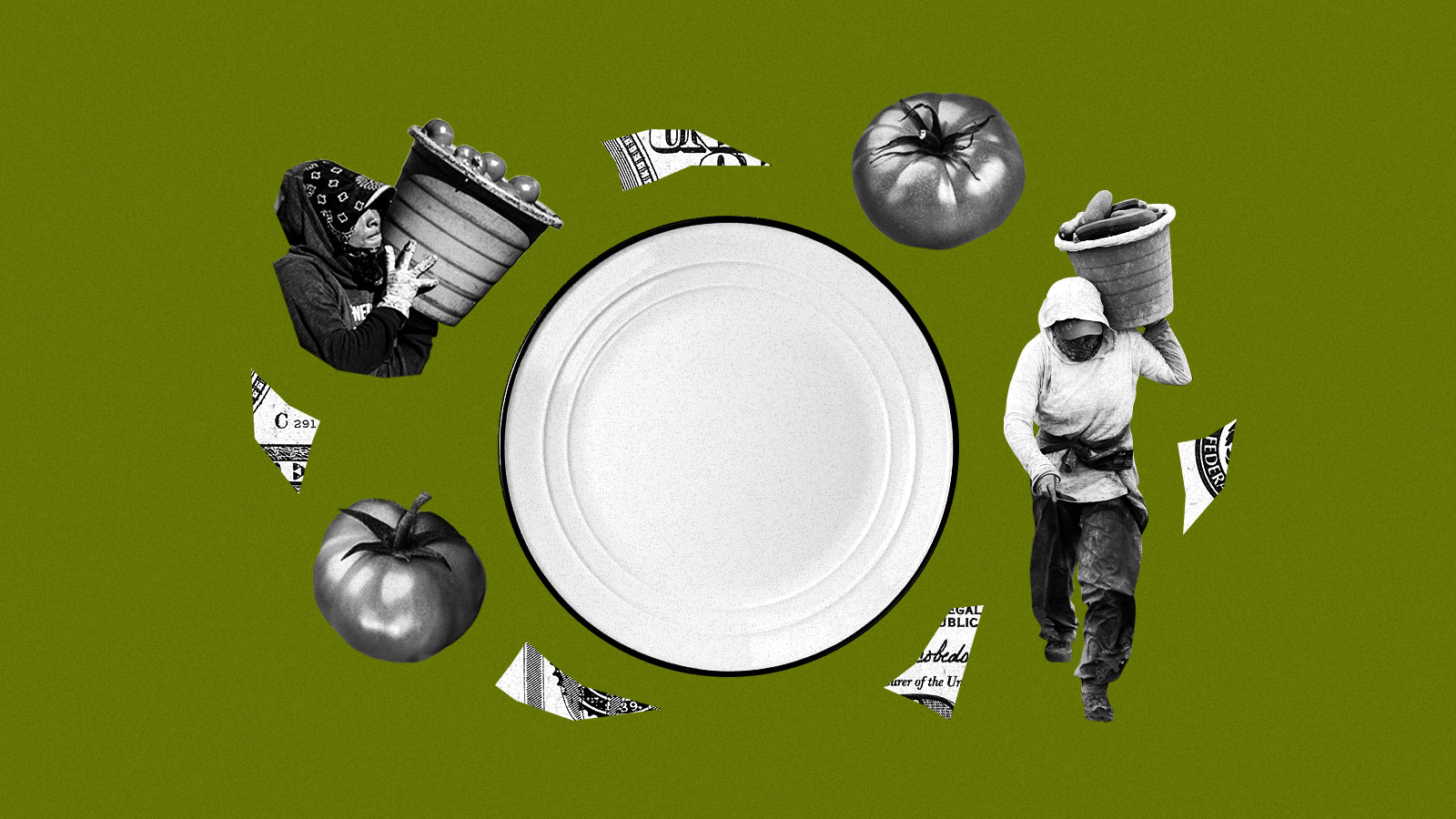
Cole pointed out that there can be many barriers to food access — for example, many people don’t apply for food stamps because they don’t realize they’re eligible, or because they may feel a sense of stigma around accepting government benefits. However, Cole said the root cause of hunger is most often wealth inequality and the high cost of living. “Getting people connected to all the benefits they’re eligible for is the first step in dealing with the food part of the problem,” she said. “But at the end of the day, the underlying problem is the money problem.”
In practice, disability, financial need, and climate impacts can all strike unexpectedly or all at once — and they can all make it harder for people to get the nutrition they need. Rubem Dasilva, a New Yorker who started receiving deliveries from Citymeals during the pandemic, said the service has helped him a lot. The 79-year-old, who lives not far from the theater district in Manhattan, has trouble affording groceries. And he avoids the matinee crowds on Wednesdays and Sundays for fear of getting jostled by pedestrians, making it harder to go to the grocery store. “I’ve been bumped like three or four times,” he said.
So the food program has been a lifesaver. “The meals are just great,” he said. “I am so grateful.”
For Dasilva, his mobility concerns do not prevent him from attempting to live life to the fullest. “I am very adventurous,” he said. “When the weather is great, I go to Central Park. I take my favorite form of travel, which is the Staten Island Ferry.” But even he has his limits. “When it’s too hot outside? I stay home.”
As climate change worsens, deadly prison heat is increasingly an everywhere problem
- Election 2024
- Entertainment
- Newsletters
- Photography
- AP Investigations
- AP Buyline Personal Finance
- AP Buyline Shopping
- Press Releases
- Israel-Hamas War
- Russia-Ukraine War
- Global elections
- Asia Pacific
- Latin America
- Middle East
- Election results
- Google trends
- AP & Elections
- College football
- Auto Racing
- Movie reviews
- Book reviews
- Financial Markets
- Business Highlights
- Financial wellness
- Artificial Intelligence
- Social Media
Drake Callender is flourishing as the last line of defense for Lionel Messi and Inter Miami
Inter Miami goalkeeper Drake Callender stops a kick on goal during the first half of an MLS soccer match against the Philadelphia Union, Saturday, Sept. 14, 2024, in Fort Lauderdale, Fla. (AP Photo/Marta Lavandier)
Inter Miami goalkeeper Drake Callender (1) deflects a corner kick during the first half of an MLS soccer match against the Philadelphia Union, Saturday, Sept. 14, 2024, in Fort Lauderdale, Fla. (AP Photo/Marta Lavandier)
Inter Miami goalkeeper Drake Callender (1) is unable to catch a goal by Philadelphia Union forward Mikael Uhre (7) during the first half of an MLS soccer match, Saturday, Sept. 14, 2024, in Fort Lauderdale, Fla. (AP Photo/Marta Lavandier)
Inter Miami goalkeeper Drake Callender prepares to work out at the MLS soccer team’s training facility, Friday, Sept. 13, 2024, in Fort Lauderdale, Fla. (AP Photo/Wilfredo Lee)
FILE - Inter Miami goalkeeper Drake Callender, center, deflects a corner kick during the first half of an MLS soccer match against the Philadelphia Union, Sept. 14, 2024, in Fort Lauderdale, Fla. (AP Photo/Marta Lavandier, File)
Inter Miami goalkeeper Drake Callender gestures during the first half of an MLS soccer match against the Philadelphia Union, Saturday, Sept. 14, 2024, in Fort Lauderdale, Fla. (AP Photo/Marta Lavandier)
FILE - Inter Miami goalkeeper Drake Callender, center, lunges to block a shot by Nashville SC midfielder Hany Mukhtar, left, during the first half of a CONCACAF Champions Cup tournament soccer match March 7, 2024, in Nashville, Tenn. (AP Photo/Mark Zaleski, File)
FILE - The ball bounces off the post behind Inter Miami goalkeeper Drake Callender and stays out of the net on a Vancouver Whitecaps shot during the second half of an MLS soccer match May 25, 2024, in Vancouver, British Columbia. (Darryl Dyck/The Canadian Press via AP, File)
FILE - Inter Miami goalkeeper Drake Callender (1) makes a save during the second half of an MLS soccer match against Orlando City, May 15, 2024, in Orlando, Fla. (AP Photo/Phelan M. Ebenhack, File)
- Copy Link copied

FORT LAUDERDALE, Fla. (AP) — Drake Callender is the answer to a pretty strong trivia question.
Years from now, it’s likely that some folks — when guessing who scored the goal that gave Inter Miami its first-ever trophy in the summer of 2023 — will choose Lionel Messi, for obvious reasons. The greatest player of his generation, probably the greatest player ever, would be the logical pick.
Spoiler alert: It wasn’t Messi.
“I did what had to be done,” Callender said.
Indeed, it was Inter Miami’s goalie — in the 11th round of a penalty-shot shootout — who scored what became the winner of that Leagues Cup final last year against Nashville SC. He scored, then saved Nashville goalie Elliot Panicco’s attempt to tie the game and extend the shootout to finish off the win. It’s a moment that forever will live in Inter Miami lore.
It’s also a reminder that in big moments, Callender is already proven. Major League Soccer’s playoffs start in about a month-and-a-half, and Callender — the go-to goalie for the best team in the league right now — can’t help but think about what it’s going to be like to challenge for the next trophy. The MLS Cup is very much on his mind, and with the way Inter Miami is playing and with Messi now back after a two-month absence because of an ankle injury, everything is looking good in his world.
“I think anytime you have opportunity to win a trophy and you do win that trophy, it makes you believe,” Callender said. “Now we know we can. We all believe and know we can win games, but part of playing the game is finding out if you can win trophies. And the group knows we can do this.”
It has been, to this point, the best season of Callender’s career. The goalie who turns 27 next month not only is the backstop for the best team in MLS but also has set a career-high for wins (19, against three losses and five ties), has tied a career best with five shutouts in MLS play this year and has the best goals-per-90-minutes average of his career at 1.37.
Put another way, the highest-scoring team in MLS — with Messi and Luis Suarez leading the way there — has one of the best goalies in MLS. It’s obviously a winning combination, as proven by how Inter Miami had a 10-point lead over Cincinnati in the Eastern Conference standings entering Sunday and a seven-point lead in the league’s overall standings over the LA Galaxy with a match in hand.
Inter Miami defender Julian Gressel had a simple way to describe Callender’s secret. “Resiliency,” Gressel said.
And that was on display Saturday.
Inter Miami surrendered a goal to the Philadelphia Union less than two minutes into the contest. Maybe Callender was sleep-deprived; he and his wife became parents for the first time a few days earlier. But with the match on the line, Callender was locked in — with Inter Miami protecting a 2-1 lead in the 85th minute, Sergio Busquets inexplicably lost control of the ball near the goal mouth. Callender made a point-blank stop to preserve the lead and Inter Miami would add a late goal to win 3-1.
“He’s bouncing back and gone through a lot the last few days, obviously,” Gressel said. “It’s been a little bit of a whirlwind for him but awesome to see him kind of step in like that and come up big when we need him. And that’s what he’s done for the whole season. I’m really happy for him.”
It’s hard not to be happy for Callender, who always seems to be one of the team’s happiest players.
When Messi arrived and joined Inter Miami in the summer of 2023, everything for everyone involved with the club immediately changed — Callender included. It’s a huge deal when Inter Miami plays now, which means more fans, more attention and more pressure.
“I’m good at blocking out the world,” Callender said. “All the noise and all the haters and the doubters and the media, sometimes it fuels me and sometimes I need a break from it. But it takes time to adjust to all the changes. I mean, I was watching this guy in Champions League a few years ago, and now I’m playing on the same team with him.”
Inter Miami didn’t get to the playoffs last year. Injuries took a toll and even Messi’s arrival wasn’t enough to pull the team from the bottom tier of the MLS standings. This season, not only will Inter Miami make the playoffs, it will be favored to win it all.
And that means another trophy moment may await the team’s goalie.
“Getting to the playoffs was one goal of ours,” Callender said. “But it’s just one step toward the big goal.”
AP soccer: https://apnews.com/hub/soccer
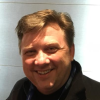

IMAGES
VIDEO
COMMENTS
By Dr. Sanchari Sinha Dutta, Ph.D. Reviewed by Emily Henderson, B.Sc. The human body has three primary lines of defense to fight against foreign invaders, including viruses, bacteria, and fungi ...
The first line of defence (or outside defence system) includes physical and chemical barriers that are always ready and prepared to defend the body from infection. These include your skin, tears, mucus, cilia, stomach acid, urine flow, 'friendly' bacteria and white blood cells called neutrophils. Pathogenic (disease-causing) microorganisms ...
skin - 1st line of defense. 15% of an adult's total weight. oil/sweat glands >> low pH on surface >> many microorganisms killed. prevents water loss. lyxozyme - enzyme in sweat that digests bacterial cell walls. stratum corneum - outer skin layer. cells constantly injured, worn, replaced. stratum basale - innermost skin layer, produces new ...
The innate immune response is really the sentinel of the human body; standing at the doors to detect invading pathogens. These sentinels fight off an invading organism while alerting the adaptive ...
The innate immune system is the body's first line of defense against intruders. It responds in the same way to all germs and foreign substances, which is why it is sometimes referred to as the "non-specific" immune system. It acts very quickly - for instance, it makes sure that bacteria that have entered the skin through a small wound are ...
A 9 minute video that explains First Line of defence in immune system. Please consider subscribing using the link: https://bit.ly/3kG2kKf0:00 Introduction 0...
The innate immune response forms the first line of defense against invading pathogens. Innate immunity includes barriers and a variety of cells and molecules that are part of the rapid response to threats to our health. In this interactive you will be introduced to the various aspects of the innate immune response and the ways in which they ...
The first line of defence is your innate immune system. Level one of this system consists of physical barriers like your skin and the mucosal lining in your respiratory tract. The tears, sweat, saliva and mucous produced by the skin and mucosal lining are part of that physical barrier, too. These quick and simple responses can eliminate some ...
Inevitably, these tissues serve as first line of defence against inorganic, organic, and microbial intruders. Epithelial cells are the main cell type of these tissues. Besides their function as cellular barrier, there is growing evidence that epithelial cells are of particular relevance as initial sensors of danger and also as executers of ...
It is a first line of defense. Innate immunity includes the external barriers of the body, like the skin and mucous membranes, which are the first line of defense in preventing diseases from entering the body. If this outer defensive wall is broken, the skin attempts to heal quickly and the second line of defence becomes involved. Adaptive Immunity
The innate immune system consists first of physical and chemical barriers to infection including the skin and mucous membranes and their secretions, ciliated surfaces, and body hairs. The second line of defense is an internal defense system designed to counter pathogenic threats that bypass the physical and chemical barriers of the body.
Beyond structural and chemical barriers to pathogens, the immune system has two fundamental lines of defense: innate immunity and adaptive immunity. Innate immunity is the first immunological mechanism for fighting against an intruding pathogen. It is a rapid immune response, initiated within minutes or hours after aggression, that has no immunologic memory. Adaptive immunity, on the other ...
The introduction of severe acute respiratory syndrome coronavirus 2 (SARS-CoV-2) into the human population represents a tremendous medical and economic crisis. Innate immunity—as the first line of defense of our immune system—plays a central role in combating this novel virus. Here, we provide a conceptual framework for the interaction of ...
If you're seeing this message, it means we're having trouble loading external resources on our website. If you're behind a web filter, please make sure that the domains *.kastatic.org and *.kasandbox.org are unblocked.
These include the skin, body hair, cilia, eyelashes, the respiratory tract, and the gastrointestinal tract. These form the first line of defence. The skin does more than providing us with fair or dark complexions. Our skin acts as a physical barrier to the entry of pathogens.
Free Essay: The first line of defense include structural, mechanical and chemical defenses. Structural defenses that people have are their skin, since the... Essay; Topics; Writing; ... The skin is the body's first line of defense it acts as a physical barrier and when there are breaks in the barrier, such as cuts the body reacts to produce ...
Vol. 135/11/1,281. On 17 September 2009 the White House announced a decision to terminate the Bush administration's plan for ten ground-based ballistic-missile interceptors in Poland and a new advanced ballistic-missile defense radar site in the Czech Republic in favor of a "phased, adaptive approach" for missile defense of Europe. 1 This ...
The first line of defense is a true and living faith. In the nineteenth century, when the United States had little military power except in wartime, U.S. power moved men all over the world, and America was the dream and ideal of millions. In those years, the U.S. peacetime army numbered from 200 to a maximum of 20,000 shortly before World War I ...
Dov S. Zakheim, First Line of Defense: The U.S. Navy Since 1945, by Paul B. Ryan, Political Science Quarterly, Volume 96, Issue 3, Fall 1981, Pages 499-500, ... A Review Essay . Black in White space: The Enduring Impact of Color in Everyday Life by Elijah Anderson. Diversifying the Courts: Race, ...
A line drawing of the Internet Archive headquarters building façade. An illustration of a heart shape "Donate to the archive" An illustration of a magnifying glass. ... First line of defense, 1755-56 : beginnings of the frontier forts / by William A. Hunter. by Hunter, William A. (William Albert), 1908-
Across Ohio, parents are the first line of defense for their children's safety, teaching right from wrong and guiding them toward success. The same standard should apply online, but the tools to ...
By delivering meals during bouts of extreme weather, including heat waves, food distribution organizations like Citymeals serve as a line of defense against the worst of climate impacts.
Leading 19-10 with 6:38 remaining in the game, the Texans entered the red zone for the first time since their touchdown drive in the second quarter. Houston had reached the Chicago 4-yard line after a 13-yard run by Cam Akers and C.J. Stroud's 16-yard completion to tight end Dalton Schultz.
Put another way, the highest-scoring team in MLS — with Messi and Luis Suarez leading the way there — has one of the best goalies in MLS. It's obviously a winning combination, as proven by how Inter Miami had a 10-point lead over Cincinnati in the Eastern Conference standings entering Sunday and a seven-point lead in the league's overall standings over the LA Galaxy with a match in hand.